Understanding Batteries / Edition 1 available in Paperback
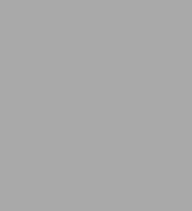
- ISBN-10:
- 0854046054
- ISBN-13:
- 9780854046058
- Pub. Date:
- 07/19/2001
- Publisher:
- RSC
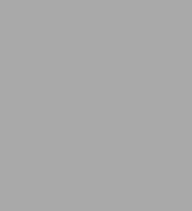
Paperback
Buy New
$41.00Buy Used
$30.13-
-
SHIP THIS ITEM
Temporarily Out of Stock Online
Please check back later for updated availability.
-
Overview
Product Details
ISBN-13: | 9780854046058 |
---|---|
Publisher: | RSC |
Publication date: | 07/19/2001 |
Series: | RSC Paperbacks , #28 |
Pages: | 254 |
Product dimensions: | 7.45(w) x 9.70(h) x (d) |
Read an Excerpt
Understanding Batteries
By Ronald M. Dell, David A. J. Rand
The Royal Society of Chemistry
Copyright © 2001 The Royal Society of ChemistryAll rights reserved.
ISBN: 978-0-85404-605-8
CHAPTER 1
Energy Storage in Batteries
1.1 BATTERIES – THEIR HISTORY AND DEVELOPMENT
A battery is a chemical device for the storage of electricity. Since electricity cannot be stored directly (except in electrolytic capacitors or superconducting coils, both of which have major technical and economic limitations) it is necessary to utilize an indirect form of storage. Possibilities include the conversion of electrical energy into potential energy (pumped-hydro schemes), kinetic energy (flywheels), thermal energy (night storage heaters), or chemical energy. One form of chemical energy is hydrogen, generated by electrolysis, which may be stored and subsequently converted back into electricity in a fuel cell. Electrolysers and fuel cells are, like batteries, electrochemical energy-conversion devices. Electrolysers play an important role in the chemical industry (for instance, in the production of chlorine and caustic soda and of metals such as aluminium and copper), while fuel cells are assuming ever greater importance for localized electricity generation, combined heat and power schemes, and as power sources for electric and hybrid electric vehicles. Electrolysers and fuel cells, however, lie outside the scope of the present book.
The convenience of batteries lies in the wide range of sizes in which they may be manufactured or assembled into packs, their ability to supply electrical power instantly, their portability (for smaller sizes), and the option of single-use or multiple-use units. The last-mentioned feature provides a useful means for classifying the many different battery systems into two broad categories: (i) 'primary batteries', which utilize the chemicals once only, in a single discharge, and then are thrown away; (ii) 'secondary batteries', which may be recharged and used again. With the latter batteries, the charging process involves the uptake of electricity and the conversion of the chemicals back into their original forms, so that they are available for a further discharge. Thus, battery charging is a special form of electrolytic process. The discharge-charge cycle may be repeated until the secondary battery deteriorates and its capacity to store charge falls below a practical level. Secondary batteries used to be known as 'accumulators' but the term has largely dropped out of use, at least in the English language. It is quite common (and acceptable) for secondary batteries to be referred to as 'rechargeable batteries'.
It is just 200 years since the invention of the first battery. This has been ascribed to Alessandro Volta (1745–1827), Professor of Natural Philosophy (physics) at the University of Pavia in Italy. His name is commemorated for all time by the unit of electrical potential, the volt. Volta's famous experiment, described in a letter to the Royal Society of London in 1800, consisted of assembling a pile of alternate silver (or brass or copper) and zinc (or tin) discs, with each pair of dissimilar metals separated from the next by a piece of cloth which was saturated with brine. One end of the pile terminated in a silver disc and the other in a zinc disc, and a continuous current of electricity was produced as soon as the two were connected by a wire conductor. This was the first galvanic, or primary, battery and became known as 'Volta's pile'. Batteries have come a long way in 200 years! It is interesting to note that the French word for battery ('la pile') stems directly from Volta's device.
The next significant step in the development of batteries was the invention of the Daniell cell by John Daniell (1790–1845), Professor of Chemistry at King's College, London. In 1836, Daniell took a copper vessel filled with copper sulfate solution and in it immersed a gullet of an ox. This unusual, and somewhat repugnant, receptacle contained a solution of sulfuric acid and a vertical zinc rod. Discharge of the resulting cell caused the zinc electrode to dissolve and copper to be deposited at the positive electrode. The cell gave a voltage of 1.1 V. This was possibly the first practical galvanic cell to give a continuous current of useful magnitude. Further modifications (Figure 1.1) included the use of porous ceramic pots ('separators') instead of animal membranes, substitution of sulfuric acid by zinc or magnesium sulfate, and the development of multi-cell batteries. Daniell cells were adopted by commercial telegraphic systems following a rapid expansion of such services in the early 1850s.
A subsequent major advance was made by the French chemist Georges Leclanche (1839–1882) who, in 1866, invented the primary cell which bears his name (see Section 5.1, Chapter 5). This consists of a zinc rod as the negative electrode and a carbon rod as the positive electrode, both immersed in a solution of ammonium chloride contained in a glass jar. The positive electrode was housed in an inner porous ceramic pot and packed around with a mixture of powdered manganese dioxide and carbon. The cell, which has been extensively developed ever since, gives a voltage of 1.5 V. A major advance took place in the late 19th century when the idea of using a zinc can as both container and electrode was patented and came into general use.
Before the invention of these galvanic cells, the only electricity known and available was static electricity, as produced by friction between dissimilar materials or in thunderstorms. The first recorded electrostatic machine was made in 1663 by the German physicist Otto von Guericke (1602–1686). In 1797, George Pearson (1751–1828) reported that in order to electrolyse water the electrostatic machine had to be discharged 14 600 times, and then produced only about 5.5 ml of a gaseous mixture of hydrogen and oxygen. Not surprisingly, therefore, the availability of a continuous current from a galvanic cell caused a revolution in technology. It formed the basis of telegraphy and of the electric door bell, and later of radio reception. In the chemical field, the supply of electricity allowed the development of electroplating, electroforming and, for the first time, the electrolytic extraction of metals such as sodium, potassium, and calcium. Copper, for use in electrical equipment, could be purified by electrorefining.
The first effective demonstration of a secondary (rechargeable) cell was given in 1859 by the French chemist Gaston Plante (1834–1889). This cell consisted of two concentric spirals of lead sheet, separated by porous cloth and immersed in dilute sulfuric acid within a cylindrical glass vessel [Figure 1.2(a)]. The lead–acid battery' gave an output of 2 V, but very little current initially because of the low surface area of the plates. By a series of discharges and charges, the chemical reactions at the surface of the plates resulted in the gradual build-up of deposits of higher surface area and the current progressively improved. This became known as the 'formation process', a term still used today in the initial charging of lead–acid batteries. In March 1860, Planté presented a battery often cells (20 V) to the Académie Française in Paris; an illustration of an early battery of Planté cells is shown in Figure 1.2(b).
An important advance in the technology of the lead–acid battery was achieved by the French chemical engineer Camille Fauré (1840–1898) who, in 1881, showed how the level of electrical charge, or the 'capacity', of the system could be greatly increased by coating the lead plates with a paste of lead dioxide and sulfuric acid. This process also reduced the time of plate formation from months to hours, and thus became part of the basic technology of the lead–acid battery industry. Without the ingenuity of Planté and Fauré, car engines would still be started by hand cranking!
Around the turn of the 19th century, the first work on batteries with alkaline solutions was reported. In Sweden, Waldemar Jungner (1869–1924) took out a patent for a 'nickel–cadmium battery'. This had a positive electrode of nickel hydroxide and, as the negative, a mixture of cadmium and iron powders. The electrodes were immersed in a concentrated solution of potassium hydroxide. At about the same time, Thomas Edison (1847–1931), who was working independently in the USA, developed and patented the 'nickel–iron battery'. This system was similar in principle, except that it used an all-iron negative electrode rather than a cadmium–iron mixture. Both cells gave 1.2 V. There was considerable rivalry between the two inventors when they learnt of each other's work and patent suits ensued. Ultimately, both alkaline batteries were brought into commercial use (see Sections 9.3 and 9.4, Chapter 9). These, in outline, were the principal discoveries of the 19th century upon which the modern battery industry is founded.
The 20th century has seen major advances in battery science and technology. Primary zinc (Leclanché) batteries have been greatly improved by the invention of alkaline batteries (Chapter 5), while advances in materials technology and cell design have revolutionized the performance of the lead–acid battery (Chapter 8). Entirely new secondary batteries have been developed and commercialized, notably the nickel–metal-hydride battery (Chapter 9) and the lithium-ion battery (Chapter 10). Many batteries for specialized applications are now manufactured, and these are introduced throughout this book. Finally, there are a number of secondary batteries in an advanced stage of development, but not yet fully commercialized (Chapter 11). Certainly, at the beginning of a new millennium, battery science and development is flourishing.
1.2 BATTERY APPLICATIONS AND MARKETS
In the 1940s, the principal domestic uses for batteries were in pocket torches (flashlamps), in a few toys, in vehicles (for starting, lighting and ignition), and in radios. Before the advent of transistors and solid-state electronics, when thermionic valves (tubes) were used in radios, it was necessary to have a source of low-voltage electricity to heat the filament and a d.c. high voltage to accelerate electrons between the cathode and the anode of the valve. Many radios in those days were remote from the electricity supply and in such circumstances two batteries were required, namely, a 2 V lead–acid 'accumulator' to supply the filament current and a 'high tension' battery consisting of 100 or 120 Leclanché cells wired in series to supply the high d.c. voltage. The radio weighed several kilograms, had to be maintained in an upright position to avoid spillage of acid, and was anything but 'portable'. No doubt there were other applications for batteries in industry and commerce, for example emergency lighting and back-up for the primitive telephone systems of the day.
Over the past 50 years, the applications for small sealed batteries in the home (consumer batteries) have expanded phenomenally. Today, small primary or rechargeable batteries are employed in a huge number of appliances. Some examples are as follows.
Household: telephones, clock-radios, security alarms, smoke detectors, portable fluorescent lamps, torches and lanterns, door-chimes, car central-locking activators.
Workshop and garden: portable tools (e.g., screwdrivers, drills, sanders), portable test meters, hedge trimmers, lawnmowers.
Entertainment: portable radios and televisions, compact disc players, tape recorders, remote controllers for televisions and videos, electronic games and toys, keyboards.
Personal hygiene and health: toothbrushes, bathroom scales, hair trimmers, shavers, blood-pressure monitors, hearing aids, heart pacemakers.
Portable electronic devices: watches and clocks, cameras, camcorders, calculators, organizers, mobile telephones, laptop and notebook computers, bar-code readers.
The average family may now have as many as 40 to 60 consumer batteries at any one time in and around the home. Many of these will be batteries of advanced design and construction that give greatly improved performance as a result of developments in materials science and technology. Although most small consumer batteries are still of the primary ('throw-away') variety, there is a growing trend to adopt rechargeable batteries as being more economical.
Moving to larger secondary batteries, principally lead–acid, there has been a growth in the market for engine-starter batteries to mirror the growth in transport. Every internal combustion engine – whether it be in a car, a van or truck, a bus, a ship, an aircraft, or even a static engine – requires a starter battery. In the road transport market, these used to be known as 'starting, lighting, ignition (SLI) batteries' to mirror their principal functions. With vehicles becoming ever more sophisticated, the required number of electric motors and other electrical facilities has mushroomed. The demands upon the battery have grown correspondingly, to the point that soon it may be necessary to fit two batteries to the private car; one battery for engine starting, the other for running the auxiliaries (see Section 8.3.5, Chapter 8). These developments have resulted in a change in name from 'SLI batteries' to 'automotive batteries' to reflect the many other functions of the modern car battery.
There has been a similar proliferation in the demand for very large, installed battery packs. Almost every public building (airports, hospitals, hotels, railway stations, stores and supermarkets, etc.) must have an uninterruptible power supply (UPS) and this requires a battery pack to take over seamlessly when the mains supply fails, until such time as a local generator can be started. Most aircraft, factories, office blocks, ships, telephone exchanges and even power stations have their own uninterruptible supply. Other applications for large primary or rechargeable batteries are in the defence field (missiles, submarine traction batteries, torpedoes), in space vehicles (satellites, space probes), in solar energy storage (cathodic protection systems, electric fences, navigation beacons, remote-area power supplies, telecommunications, water pumping), and in electric and hybrid electric vehicles (bicycles, fork-lift trucks, golf-carts, invalid conveyances, specialist road vehicles, tugs and tractors, a few cars). Although many of these applications utilize long-established lead–acid or nickel–cadmium rechargeable batteries, these have been transformed into superior units in recent years by major advances in materials and design. In the past decade, new rechargeable batteries have been introduced into consumer markets. Moreover, there are now specialized batteries with unconventional chemistries for use in the military and the space fields.
The size range of batteries is now enormous. The scale extends from small button cells of energy content ~0.1 Wh, all the way to load-levelling batteries of energy content ~10 MWh. (Note, a battery of energy content 0.1 Wh is theoretically capable of supplying a power of 0.1 watt for an hour, or 1.0 watt for a tenth of an hour, although in practice the recoverable energy does depend upon the discharge rate.) Few other commodities come in such a range of sizes, certainly not pots of paint or packets of cornflakes! Some typical applications for batteries of differing size are given in Table 1.1.
The size and value of the market for different classes of battery is difficult to estimate. The mass markets are in consumer primary and secondary cells, automotive batteries, and in industrial batteries for stationary and mobile power. As long ago as 1989, the annual markets in the USA for primary and secondary batteries were estimated as US$2.3 billion and US$4.2 billion, respectively. In 1991, the world battery market was said to be US$21 billion; 40% by value was supplied by primary batteries and 60% by secondary batteries. Undoubtedly, the market has grown since then. A more recent (1997) estimate gave the world demand for small, consumer secondary cells as a little over two billion units, of value US$3–5 billion. Add to this the huge demand for primary cells and larger rechargeable batteries and it is clear that this is indeed a substantial industry. Moreover, the major expansion of the battery industry in developing nations, such as China, and the present trend towards more sophisticated, higher cost batteries (such as alkaline-manganese primary batteries, and nickel–metal-hydride and lithium-ion secondary batteries) are greatly accelerating the industry turnover. For example, global sales of nickel–metal-hydride batteries have grown from 310 to 800 million units between 1995 and 1998, while sales of lithium-ion batteries have increased from 35 to 280 million units. Today, the battery industry worldwide is a vast business with strong prospects for substantial growth in the near future.
(Continues...)
Excerpted from Understanding Batteries by Ronald M. Dell, David A. J. Rand. Copyright © 2001 The Royal Society of Chemistry. Excerpted by permission of The Royal Society of Chemistry.
All rights reserved. No part of this excerpt may be reproduced or reprinted without permission in writing from the publisher.
Excerpts are provided by Dial-A-Book Inc. solely for the personal use of visitors to this web site.