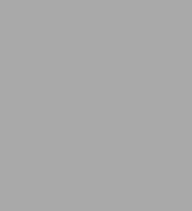
- ISBN-10:
- 0750311053
- ISBN-13:
- 9780750311052
- Pub. Date:
- 08/05/2016
- Publisher:
- Iop Publishing Ltd
- ISBN-10:
- 0750311053
- ISBN-13:
- 9780750311052
- Pub. Date:
- 08/05/2016
- Publisher:
- Iop Publishing Ltd
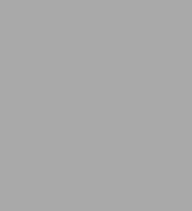
Buy New
$159.00Buy Used
-
-
SHIP THIS ITEM
Temporarily Out of Stock Online
Please check back later for updated availability.
-
Overview
Product Details
ISBN-13: | 9780750311052 |
---|---|
Publisher: | Iop Publishing Ltd |
Publication date: | 08/05/2016 |
Edition description: | New Edition |
Pages: | 100 |
Product dimensions: | 7.26(w) x 10.37(h) x 0.66(d) |
About the Author
Table of Contents
Preface xiv
Acknowledgement xviii
Author biography xix
1 Atomic considerations 1-1
1.1 Isotopes 1-1
1.2 Nuclear stability and radioactive decay 1-2
1.3 Alpha-decay (α-decay) 1-3
1.4 Beta-decay (β-decay) 1-3
1.5 Beta+/positron emission or electron capture 1-4
1.6 Gamma emission 1-4
1.7 How do the mechanisms relate to each other? 1-4
1.8 Radioactive half-life 1-5
1.9 Decay series 1-6
1.10 Observations on isotope stability 1-7
1.11 Binding energy 1-7
1.12 Fission and fusion 1-9
1.13 Spontaneous fission 1-10
1.14 Inducing fission and chain reactions 1-11
1.15 Neutron absorption and fissile and fertile isotopes 1-11
1.16 Increasing fission yield 1-12
1.17 What are the key criteria for nuclear fission? 1-13
1.17.1 Required components 1-13
1.17.2 Desirable components 1-14
References 1-14
2 Radiation damage 2-1
2.1 Key definitions 2-2
2.1.1 Primary knock-on atom (pka) 2-2
2.1.2 Threshold displacement energy (TDE) 2-2
2.1.3 Displacements per atom (dpa) 2-2
2.2 Radiation damage 2-3
2.3 Prediction of damage-the Kinchin-Pease methodology 2-4
2.3.1 Nuclear and electronic stopping factors/powers 2-5
2.3.2 How do these powers effect the stopping of the incident particles? 2-5
2.3.3 The Kinchin-Pease methodology for predicting levels of damage 2-5
2.3.4 Modifications to Kinchin-Pease 2-8
2.4 Implications of damage 2-9
2.4.1 No recovery from damage 2-9
2.4.2 Full recovery from damage 2-9
2.4.3 Partial recovery from damage 2-9
2.5 Outcomes from damage 2-9
2.5.1 Basic recovery 2-9
2.5.2 Damage vs recovery 2-11
2.6 Modelling damage build-up in materials 2-12
2.6.1 Direct impact 2-13
2.6.2 Defect accumulation 2-14
2.6.3 How do they compare? 2-14
2.7 The bulk effects of damage 2-18
2.7.1 Expansion (swelling) 2-18
2.7.2 Radiation-induced segregation 2-19
2.7.3 Thermal conductivity 2-19
2.7.4 Embrittlement 2-20
2.7.5 Cracking 2-20
References 2-22
3 Nuclear fuel, part 1: fuel and cladding 3-1
3.1 What is required from fuel in a fission reactor? 3-1
3.2 Reminder of the fission process 3-1
3.3 What are the realistic types of fuel? 3-2
3.4 Uranium 3-2
3.4.1 Oxides of uranium 3-4
3.4.2 Uranium (IV) oxide (UO2) 3-4
3.4.3 Uranium (VI) oxide (UO3) 3-5
3.4.4 Uranium (IV/VI) oxides 3-6
3.4.5 Hyper/hypostoichimetric UO22±x 3-7
3.5 Plutonium 3-8
3.5.1 Oxides of plutonium 3-8
3.5.2 Plutonium (TV) oxide (PuO2)) 3-9
3.5.3 Mixed oxide fuel (MOX) 3-10
3.5.4 What form is the fuel? 3-10
3.6 Fuel containment 3-11
3.7 Zirconium-based cladding 3-14
3.7.1 Material properties of zirconium 3-14
3.7.2 Development of zirconium-based alloys for nuclear applications 3-15
3.7.3 Zirconium hydridation 3-17
3.8 Iron-based cladding 3-19
3.8.1 Advanced gas-cooled reactor cladding 3-19
3.9 How do fuel and cladding relate to each other? 3-19
References 3-20
4 Nuclear fuel, part 2: operational effects 4-1
4.1 Initial stages 4-1
4.2 Classical effects from heating 4-2
4.2.1 Why does sintering occur? 4-3
4.2.2 What are the outcomes of sintering? 4-3
4.2.3 Why do we care? 4-4
4.3 Fission products 4-4
4.3.1 Volatiles/gaseous 4-6
4.3.2 Metal particulates 4-6
4.3.3 Combined effects 4-6
4.3.4 Key impacts of fission 4-7
4.4 Initial reactor operation 4-7
4.5 Fuel cladding under operation within the core 4-10
4.5.1 Damage formation and expansion 4-11
4.5.2 Hardness and ductility 4-11
4.6 Fuel and cladding 4-11
4.6.1 Pellet-clad interaction (PCI) 4-12
4.7 Cladding corrosion 4-13
4.7.1 Corrosion mitigation and CRUD 4-14
4.7.2 Stress corrosion cracking (SCC) 4-15
4.7.3 The role of zirconium hydrides 4-16
4.7.4 Radiolysis of cooling water 4-17
References 4-18
5 Evolution of reactor technologies 5-1
5.1 Generation I-prototype reactors 5-2
5.1.1 Pressurised water reactors (PWR) 5-6
5.1.2 Gas-cooled reactor (MAGNOX) 5-7
5.2 GenII-commercial reactors 5-9
5.2.1 Advanced gas-cooled reactors (AGR) 5-10
5.3 GenerationIII/generationIII+-evolved designs 5-11
5.3.1 Westinghouse AP and Areva EPR 5-11
5.3.2 GE-Hitachi ABWR 5-13
5.3.3 General design considerations 5-13
5.4 Molten salt reactors 5-14
5.4.1 The molten salt reactor experiment (MSRE) 5-14
5.4.2 Aircraft reactor project 5-14
5.5 Summary 5-16
5.5.1 Early reactors (GenI) 5-16
5.5.2 Commercial reactors (Genii) 5-17
5.5.3 Evolved designs (GenIII/Genin+) 5-17
References 5-17
6 The challenges for materials in new reactor designs 6-1
6.1 Generation IV-genesis 6-1
6.2 Reactor types 6-2
6.2.1 Very high temperature reactor (VHTR) 6-2
6.2.2 Molten salt reactor (MSR) 6-2
6.2.3 Supercritical water reactor (SCWR) 6-2
6.2.4 Gas-cooled fast reactor (GFR) 6-4
6.2.5 Sodium-cooled fast reactor (SFR) 6-4
6.2.6 Lead-cooled fast reactor (LFR) 6-5
6.3 Material challenges in GenIV 6-6
6.3.1 Fuel 6-6
6.3.2 TRI-ISOtropic (TRISO) fuel 6-7
6.4 Containment 6-9
6.4.1 Key requirements for cladding 6-10
6.4.2 Liquid fuel 6-11
6.5 Radiation damage 6-14
6.6 Alternative reactor technology 6-16
6.7 Travelling wave reactor 6-16
6.8 Thorium reactors 6-17
6.8.1 Properties of thorium 6-17
6.8.2 Thorium-based fuel 6-17
6.8.3 Thorium reactor designs 6-19
6.9 Small modular reactors (SMR) 6-20
6.9.1 Proposed designs 6-20
References 6-21
7 The challenges of nuclear waste 7-1
7.1 Sources of nuclear waste 7-1
7.2 Natural sources of uranium/thorium 7-2
7.2.1 Oklo-a natural nuclear reactor 7-3
7.2.2 Development of ceramic waste forms 7-5
7.2.3 Supercalcine 7-7
7.2.4 Designing ceramic waste forms 7-7
7.2.5 Synroc 7-8
7.2.6 Criticality prevention 7-9
7.3 Long-term effects in waste forms 7-9
7.3.1 Radiation damage 7-9
7.4 Long-term behaviour of nuclear waste 7-10
7.4.1 Bulk effect of amorphisation 7-11
7.4.2 Helium bubble formation 7-12
7.5 Geological disposal of nuclear waste 7-12
7.5.1 Corrosion and nuclear waste 7-13
7.6 Ceramics and glasses-comparison 7-14
7.6.1 Ceramics 7-14
7.6.2 Glasses 7-15
7.6.3 Glass-ceramics 7-16
7.7 Transmutation 7-17
7.7.1 Implications of beta-decay 7-17
References 7-20
8 Materials and nuclear fusion 8-1
8.1 Atomic background and recap 8-1
8.2 Requirements for fusion 8-4
8.3 ITER-the International Thermonuclear Experimental Reactor 8-5
8.3.1 Design of ITER 8-5
8.4 Outcomes and challenges in fusion 8-5
8.4.1 Fuel 8-6
8.4.2 Heat 8-6
8.4.3 Helium 8-7
8.4.4 Neutron production 8-8
8.5 Material requirements 8-8
8.5.1 First/plasma-facing wall 8-9
8.6 Radiation damage and the first wall 8-9
8.7 Sputtering 8-10
8.8 Gas bubble formation 8-12
8.8.1 Direct implantation of He from plasma 8-12
8.9 The divertor 8-13
8.10 Breeding and heat generation 8-16
8.11 Tritium breeding 8-17
8.11.1 Solid breeding 8-17
8.11.2 Liquid breeding 8-17
8.11.3 Pb-Li eutectic 8-17
8.11.4 Molten salt-FLiNaBe 8-17
8.11.5 Comparison of methods 8-18
8.12 Challenges in fission and fusion 8-20
References 8-20
9 Mistakes made and lessons learnt 9-1
9.1 Windscale-Pile 1 9-1
9.1.1 Wigner energy 9-3
9.1.2 Graphite 9-3
9.1.3 Timeline of incident 9-4
9.1.4 Aftermath 9-5
9.1.5 What caused the tire? 9-6
9.1.6 Key outcomes from the fire 9-6
9.2 Three Mile Island-Reactor 2 9-6
9.2.1 The China syndrome 9-7
9.2.2 Three Mile Island nuclear reactor complex 9-7
9.2.3 Timeline of incident 9-7
9.2.4 Aftermath 9-9
9.2.5 What caused the initial problem that led to the incident? 9-10
9.2.6 Key outcomes from the incident 9-10
9.2.7 Loss of coolant accident (LOCA) 9-10
9.3 Chernobyl-Reactor 4 9-12
9.3.1 Reaktor Bolshoy Moschonosty Kanalny (RBMK)-1000 9-12
9.3.2 Timeline of incident 9-13
9.3.3 Aftermath 9-15
9.3.4 What caused the incident? 9-15
9.3.5 Key outcomes from the incident 9-16
9.4 Fukushima Daiichi 9-16
9.4.1 Boiling water reactor (BWR) 9-17
9.4.2 Timeline of incident 9-18
9.4.3 Aftermath 9-19
9.4.4 What caused the incident? 9-20
9.4.5 Key outcomes from the incident 9-20
9.5 How do the incidents compare? 9-20
References 9-21