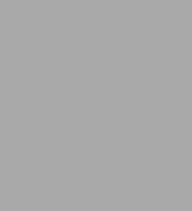
- ISBN-10:
- 0521674557
- ISBN-13:
- 9780521674553
- Pub. Date:
- 01/19/2006
- Publisher:
- Cambridge University Press
- ISBN-10:
- 0521674557
- ISBN-13:
- 9780521674553
- Pub. Date:
- 01/19/2006
- Publisher:
- Cambridge University Press
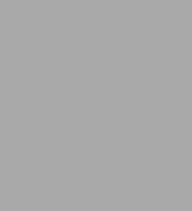
Buy New
$72.99Overview
Product Details
ISBN-13: | 9780521674553 |
---|---|
Publisher: | Cambridge University Press |
Publication date: | 01/19/2006 |
Series: | Ecology, Biodiversity and Conservation |
Pages: | 236 |
Product dimensions: | 5.98(w) x 8.98(h) x 0.51(d) |
About the Author
Read an Excerpt
Cambridge University Press
0521854342 - Nonequilibrium Ecology - by Klaus Rohde
Excerpt
Introduction
Ecology has long been shaped by ideas that stress the sharing of resources and the competition for those resources, and by the assumption that populations and communities typically exist under equilibrium conditions in habitats saturated with both individuals and species. This view can be traced back to Linnaeus, who considered an equilibrium in nature; Adam Smith, who contributed the idea that competition can lead to equilibrium in a community; and Malthus, who suggested that greater growth in demand than in supply would lead to competition for limited resources. Among well known ecologists, Hutchinson (1948) took it for granted that stability (owing to "self-correcting mechanisms") is characteristic of most ecological systems and permits their persistence, and, according to Dobshansky (1957, cited by Cooper 2001): "natural selection, and hence the evolutionary process, are the outcome of competition; and therefore are governed by density-dependent factors." Some ecologists were always aware of the possibility of nonequilibria, but the majority ignored it, especially in connection with theory in ecology. In several widely used older ecological texts, competition and equilibria are discussed in depth, but nonequilibria are not mentioned at all or only in a very cursory fashion (e.g., Pielou 1969; MacArthur 1972; Cody and Diamond 1975; Ehrlich et al. 1977). This has changed somewhat in recent years, particularly in population ecology (e.g., Chesson and Case 1986; Diamond and Case 1986; DeAngelis and Waterhouse 1987; Krebs 2001). Nevertheless, many workers still seem to be pre-occupied with looking for evidence of competition and equilibria. In the 20 review articles in Fenchel (1999), which deal with aspects of ecology supposed to be of major current and future interest, only one contains the term nonequilibrium as important for future research in the list provided by the editors (Lindström et al. 1999). Negative results, i.e., results failing to provide evidence for competition, seem to be reported as rarely as they were 20 years ago, when Pickett (1980) and Price (1980, 1983, 1984) drew attention to this lack of information. Rosenzweig and Ziv (1999) state "Theory suggests that higher diversity should shrink niches, allowing the coexistence of more species locally," which clearly is an assumption of equilibrium ecology, and even some of the basic assumptions of Hubbell's (2001) neutral theory of biodiversity are not different from those of equilibrium ecology. He assumes, for example, that communities are saturated with individuals leading to a zero-sum game, that numbers of individuals of one species can increase only at the expense of individuals of other species, and that there is saturation with species.
There is no recent book that focuses on nonequilibrium aspects of ecology. This book aims at filling this gap. After a brief outline of concepts and problems, an outline of historical milestones in the evolution of ideas, and the description of some empirical studies demonstrating equilibrium and nonequilibrium, I define the major problem discussed in this book (the relative importance of equilibrium and nonequilibrium), using the arguments in favour of prevailing equilibrium conditions in nature provided by Rosenzweig (1995). I then discuss evidence, including some detailed examples, for nonequilibrium and equilibrium in populations (and metapopulations), in extant communities and in ecological systems over evolutionary time, including nonequilibrium due to recent and present mass extinctions. The assumption that competition is of overriding importance is central to equilibrium ecology, and much space is devoted to its discussion. An autecological comparison of two parasite species, using evidence from phylogeny, morphology, life-cycle, and ecological studies, attempts to evaluate whether equilibrium assumptions, in particular assumptions on interspecific competition, can explain adaptations and extant patterns of distribution. Finally, because communities of some taxa appear to be shaped more by competition than others, an attempt is made to find an explanation for these differences.
The bias towards equilibrium ecology is at least partly due to a bias in selecting models for study. Indeed, there has been a strong bias towards pest and some other insect species occurring at high densities, and towards birds and terrestrial vertebrates, which in many cases live in communities that are to a large degree saturated and exposed to competition for limiting resources. This book discusses some such examples, but, in addition, uses many examples from systems that have often been neglected, i.e., parasitic and aquatic systems. Species in such systems represent the majority of eukaryotic species on Earth and their study may give a more balanced view of how ecological systems work. Also, many parasites live in well defined habitats and communities with an almost unlimited number of replicas, which can easily be manipulated in natural experiments, i.e., they are excellent objects for ecological studies.
1 · Concepts and problems
Concepts of equilibrium (balance of nature) and nonequilibrium
The concepts of equilibrium/nonequilibrium have been used differently by different authors, as will be shown in the following selected examples.
Pianka (1974), in the second edition of his widely used Evolutionary Ecology, makes the case succinctly for equilibrium in ecological systems (modified somewhat in later editions). The main points listed by him are that:
ecological systems and their components have been shown to be in dynamic equilibrium near steady states in many studies;
in communities, production and respiration "must ultimately balance";
even nonclimax communities, which have not reached a steady state, are probably "in some kind of equilibrium," determined by the frequency of disturbances and destruction of other successional stages and the rate of successional change;
in most communities, rates of energy influx and outflow in each trophic level balance out exactly;
on islands, immigration and extinction of species are balanced;
in populations, over long periods, birth rates equal death rates; and
prey-predator and similar pairs must be "in some sort of ecological and evolutionary balance to coexist with one another over any period of time."
The assumption that competition plays a central role in ecology is implicit in Pianka's discussion. This notion has since been explicated by Chesson and Case (1986), who define the assumptions of "classical competition theory" as follows:
life history characteristics of species are adequately summarized by the per capita growth rate of species;
deterministic equations are sufficient to model population growth, and environmental fluctuations need not be considered;
the environment is spatially homogeneous and migration is unimportant;
competition is the only important biological interaction; and
coexistence requires a stable equilibrium point.
They further summarize the consequences of these assumptions, i.e., that n species can coexist only if there are no fewer than n limiting resources, and that there is a limiting similarity between species: species evolved in response to interspecific competition. Assumption 4 (above) is relaxed when predators are present, i.e., n species may coexist when fewer than n resources are present. If the environment favours different species in different patches, n species may coexist in at least n patches, even if the species use the same limiting resource.
The authors then contrast these assumptions with those of nonequilibrium situations. Nonequilibrium is seen as "any situation where species densities do not remain constant over time in each spatial location." Even if fluctuations occur at small spatial scales that level each other out at larger scales, an explanation would still be a nonequilibrium explanation if the fluctuations are an essential part of the explanation. Chesson and Case (1986) discuss the following four points that deviate from classical equilibrium assumptions:
populations are not at a point equilibrium but competition still occurs continuously and is important; this may permit more than n species to coexist on n resources (e.g., Armstrong and McGee 1980);
fluctuations in population density or environmental variables are dominant, population dynamics may be density-independent;
means and variances of environmental fluctuations are not constant over time; and
populations are random-walking, but time to extinction is so long that species persist over a long time ("slow competitive displacement").
Concerning nonequilibrium, Cappucino (1995) argues that only randomly walking populations are unambiguously nonequilibrial. All other usages are misplaced. Thus, the most commonly used meaning of nonequilibrium in populations refers to situations where local populations do not trend towards a point equilibrium (density-vagueness of Strong [1984]; stochastic boundedness of Chesson [1978]). However, according to Cappucino, fluctuations in such populations are bounded and therefore regulated and not nonequilibrial. Moreover, if all subpopulations of a metapopulation are randomly walking, the metapopulation as a whole must also randomly walk to extinction (Chesson 1981).
An important discussion of equilibrium in nature is provided by Cooper (2001), who looks critically at the various arguments given by different authors in favour of equilibrium. He distinguishes two "balance of nature" arguments in population/community ecology. The first argument is based on the assumption that there is a strong tendency towards constancy in population size. But how much variability is allowed before the population is no longer considered to conform to this alleged constancy? The second argument has been developed to overcome this difficulty. It simply assumes that the balance is represented by populations tending to persist. But this persistence must be the result of some kind of regulation. The reason is that random-walking populations must become extinct over time; therefore, those that do persist must be regulated in some way, in the sense that they must display statistical stability over time.
Cooper refers to several authors who have proposed explanations of stability (i.e., May 1973; Chesson 1981, 1982; Murdoch 1994; Dennis and Taper 1994; Turchin 1995). The last two papers discuss the problem in terms of stationary probability distributions (SPD) in population density- time series. Population densities are assumed to fluctuate around a mean density level, and the variance of fluctuations is bounded in the long term. Turchin (1995) defines equilibrium (or being regulated) as existence of an SPD. Since most populations persist, i.e. have an SDP, they must be in equilibrium. In other words, they must be regulated. Also, according to this argument, regulation implies density dependence, because populations would either become extinct or increase ad infinitum, if density-dependent factors did not operate (May 1986). Most likely, density-dependent factors are competitive ones, at least if predation and other such influences do not limit population density to a level below which competitive effects can become operative. However, as pointed out by Cooper (2001) and others to whom he refers, all populations become extinct sooner or later, and one would have to demonstrate that populations persist longer than expected if they simply executed random walks. Furthermore, a tendency to return may only be a necessary but not a sufficient condition for equilibrium (or regulation), i.e., empirical evidence for the occurrence of regulation has still to be given. In other words, Cooper argues that the inclination of many (if not most authors) to use a-priori arguments for a balance in nature (equilibrium, regulation) is ill advised.
History of equilibrium and nonequilibrium ecology: some milestones in the evolution of ideas
This account concentrates on the development of some important ideas in the equilibrium-versus-nonequilibrium debate, it does not attempt to give a complete history of equilibrium and nonequilibrium ecology. An early account of species equilibrium was given by Wilson (1969), and an excellent history of population ecology is given by Kingsland (1995). Harris (1986) provides some information on the development of equilibrium and nonequilibrium theory in ecology, and Egerton (1973) has given a detailed discussion of the history of the concept of balance in nature (see also Pimm 1991). According to Walter and Patterson (1995), "an uncritical and scientifically unsupported belief in the strength and central role of competition (both interspecific and intraspecific) in evolution and ecology is traceable to Darwin (1859) and beyond." Darwin wrote in his Origin of Species (1859, cited by Silverton 1980) that "Battle within battle must be continually recurring (in nature) with varying success; and yet in the long-run the forces are so nicely balanced, that the face of nature remains uniform for long periods of time, though assuredly the merest trifle would often give the victory to one organic being over another." The "beyond" Darwin includes Linnaeus, who considered an equilibrium in nature; Adam Smith, who contributed the idea that competition can lead to equilibrium in a community; and Malthus who suggested that greater growth in demand than in supply would lead to competition for limited resources (see Hengeveld and Walter 1999 for a further historical discussion). Hutchinson (1948) takes it for granted that stability (owing to "self-correcting mechanisms") is characteristic of most ecological systems and permits their persistence. Or, as stated by Dobshansky (1957, cited by Cooper 2001) "natural selection, and hence the evolutionary process, are the outcome of competition; and therefore are governed by density-dependent factors." This assumption is the basis for Orians' (1962) opposition to the views of Andrewartha and Birch (1954), who did not recognize the predominant role of competition and the general existence of equilibria, and who were therefore supposed to have left ecology without a basis in the theory of natural selection.
As mentioned previously, Hutchinson (1948, 1959) believed that competition is a major factor determining species diversity and patterns in ecological communities, but he nevertheless recognized in 1961 that there are many more phytoplankton species in lakes than allowed by the classical competition theory (the "paradox of the plankton"). He suggested that nonequilibrium processes may provide an explanation for this, particularly those associated with temporal variations in lakes. Among eminent ecologists, Gleason (1926), Ramensky (1926), and much later, Whittaker (1967) were of the opinion that nonequilibria are important, and as early as 1952, von Bertalanffy concluded that living systems are open systems characterized by a continuous flow of substances and energy across their boundaries, that cannot establish true equilibria as found in closed systems.
Significant contributions to the establishment of what can be called nonequilibrium ecology are provided by Andrewartha and Birch (1954, 1984), and also Andrewartha (1970). Andrewartha and Birch, based on their extensive and intensive studies of many natural populations, conclude that too much emphasis on competition is fallacious. Most natural populations never become sufficiently dense to use a great proportion of the resources that they require. Density-dependent factors and competition therefore do not become operative (for details see p. 62).
In 1974, Levin and Paine published a seminal paper in which they showed that disturbance increased environmental heterogeneity, preventing local patches from ever achieving equilibrium, but, according to Berryman (1987), the first explicit statement of the viewpoint of nonequilibrial ecology was given by Caswell (1978), who said "equilibrium theories are restricted to behavior at or near an equilibrium point, while nonequilibrium theories explicitly consider the transient behavior of the system." This still implies the existence of equilibrium points, but systems are rarely at or close to these points. As earlier shown experimentally by Huffaker (1958) and theoretically by Maynard Smith (1974), spatial dimensions can prevent a system from "reaching a closed deterministic solution." In Berryman's view, "drawing distinctions between equilibrium and nonequilibrium theories of populations is distracting and misleading." Instead, there needs to be a theory developed that would explain population behavior both close to and far from equilibrium.
Extensive discussions of the nonequilibrium aspects of ecology are given in Evolutionary Biology of Parasites by Price (1980), and in A New Ecology. Novel Approaches to Interactive Systems edited by Price et al. (1984). In the Introduction to the latter volume, Price et al. (1984) point out that looking at various textbooks on ecology gives the clear impression that interspecific competition is of great importance, whereas the opposite view, i.e., that interspecific competition may be unimportant or only one of several equally important processes, has found little credence. An important reason for this unbalanced view, in the opinion of these authors, is the fact that negative data (for example those on the absence of competition) are not considered worthy of publication. Furthermore, "many scientists feel compelled to fit data into some existing body of theory, and do not feel equally compelled to falsify theory." Thus, very little of the supposed evidence for interspecific competition has been tested objectively (see Connell 1980, and pp. 64-65). Price (1984) therefore proposes that ecologists should test several hypotheses ("paradigms") to elucidate simultaneously processes determining community structure. These hypotheses include the null hypothesis that species respond individualistically to selection pressures, without competing for resources, simply living where conditions are favourable (Gleason 1926, Ramensky 1926); the resource heterogeneity hypothesis, that the number of species in a community is positively correlated with the number of resources, and their abundance with the abundance of the resources needed by the species; the island or patch size hypothesis, according to which area alone determines the number of species; the time hypothesis, according to which species numbers are determined by (ecological or evolutionary) time; and the enemy impact hypothesis, which says that enemies (e.g., parasites or predators) limit population sizes below the level at which resources become limiting. But not only should the relative importance of the various hypotheses be examined, but also the mechanisms involved. Hypotheses may be difficult to test for many communities and under many conditions. Price (1984, p. 374) lists characteristics of communities that make them suitable for hypothesis testing.
Price (1980) devotes a large chapter in his Evolutionary Biology of Parasites to "nonequilibria" in populations and communities, and other chapters also contain relevant information on this subject. In particular, the chapter on "Ecological niches, species packing, and community organization" gives a comprehensive discussion of nonequilibrium in parasite communities. Equilibrium is a population state in which birth and death rates are equal, i.e., where there is no population growth (MacArthur and Wilson 1967, May 1973), or - more realistically in stochastic environments - where population size fluctuates around an average with steady average variance (May 1973). If variance is high, systems are unstable, if it is low, they are stable. Price (1980) draws attention to the difference between parasites and predators or browsers: a parasitic individual typically spends much of its life in a single or perhaps two patches (host individuals), whereas the latter search many patches. Globally, for parasites, there may be equilibrium, i.e., the proportion of patches occupied may remain stable, but within a patch, nonequilibrium conditions are highly likely (e.g., orange mites studied by Huffaker 1958). Furthermore, parasite distributions within host populations are usually overdispersed, leading to undercolonization of most host individuals. Price notes that disruptions in parasite-host systems commonly occur, resulting in nonequilibrium states. The complexity of biotic interactions characteristic of a parasitic way of life, according to Price, is the main factor generating nonequilibrium; (also May 1973: complexity decreases stability). Price discusses several examples in detail. In each of these examples, patchy resources (host individuals) lead to a low probability of colonization, and ephemeral patches result in a high probability of extinction. Schistosoma populations provide one of his examples. Here, patchiness of resources is indicated by the patchy distribution of water, the independent differentiation of snail host populations, highly specific parasite strains, and patchiness of snail and vertebrate host distribution. Ephemeral patches are due to temporary availability of water, high mortality, sterility or reduced fecundity of snail hosts, the dynamics of snail and vertebrate hosts, and developing host resistance.
However not only in populations, but also in communities of parasites, equilibrium conditions are unlikely, since the parasite populations of which they are comprised are all in nonequilibrium (Price 1980). Mechanisms leading to nonequilibrial communities are the same as for populations: if colonization probabilites are low and extinction probabilities high - which is usually the case - nonsaturation and nonequilibrium will result. Price discusses a number of examples and concludes that, if interspecific competition occurs in such systems, it is intermittent.
Strong (1984) introduced the term density-vagueness to describe parameters of birth and death rates that are only weakly explained by density. Sometimes, the density effect can be inferred only with imagination, or the correlation may be low (although statistically significant). Explanations other than density may be age, weather, migration, and others. He provides evidence that density dependence is not evident in a large number of studies and life-cycle stages, but he also points out why density dependence may sometimes be underestimated (but also overestimated). Nevertheless, for insects at least, density effects, where they occur, are often weak, intermittent, or discontinuous.
The belief that equilibrium conditions and competition are the major factors determining ecological processes, underlies and informs most ecological modelling. The concepts of evolutionary stable strategy (ESS), continuously stable strategy (CSS), and neighborhood invader strategy (NIS), for example, are based to a large degree on such an assumption (e.g., Apaloo 2003, further references therein).
Among major recent approaches, mentioned by Paine (2002), are the necessity to distinguish between open and closed systems (already recognized by von Bertalanffy 1952 and Levin and Paine 1974), and that "equilibrium may exist only in an abstract sense." Chesson and Case (1986) plead for a pluralistic approach: coexistence may be partly due to differences in resource use, partly because species respond differently to the environment, and partly because the advantage of one species over another is very small, leading to very slow competitive displacement; (see also the discussion in McIntosh 1987).
One of the clearest and most radical redefinitions of the aims of ecology comes from Hengeveld and Walter (1999, further references therein) and Walter and Hengeveld (2000, further references therein; see also Walter 1995 and Walter and Patterson 1995). Hengeveld and Walter distinguish two paradigms in current ecology, which have coexisted for some time but are mutually exclusive; they are the demographic and the autecological paradigms. The former, which is the better developed and generally accepted approach, accepts that different species are demographically similar although they fulfill different functions in communities. Intra- and interspecific competition are of paramount importance, leading to coevolution of species by optimization processes. Optimization is thought to be possible because the abiotic component of the environment is on average constant. In contrast, the autecological approach accepts species as dissimilar entities which are affected by abiotic as well as demographic factors. Optimization cannot occur because the environment is very variable in space and time. In the demographic paradigm, the important question is why do so many species share the same resources, and the emphasis is on evolution as the result of short-term ecological optimisation processes. In the autecological paradigm, the central question relates to how species arose and how they persist within a variable and heterogeneous environment. It focuses on the idiosyncratic nature of adaptations, species and their spatial response to environmental circumstances. In the demographic paradigm, nature is balanced, i.e., there are population equilibria maintained demographically by biotic processes; structured communities exist that consist of populations of several species and are saturated with species that optimally partition resources; and ecological-evolutionary processes occur in a discrete locality. In the autecological paradigm, because of the continually changing environmental (biotic and abiotic) conditions which do not permit optimization, emphasis is on survival and reproduction and not on quantification and comparison of differences in reproductive outputs between species. Physiological, morphological and
© Cambridge University Press