Greenhouse Gas Emission and Mitigation in Municipal Wastewater Treatment Plants available in Paperback
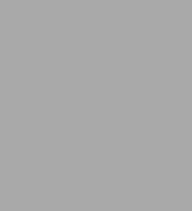
Greenhouse Gas Emission and Mitigation in Municipal Wastewater Treatment Plants
- ISBN-10:
- 1780406304
- ISBN-13:
- 9781780406305
- Pub. Date:
- 12/13/2017
- Publisher:
- IWA Publishing
- ISBN-10:
- 1780406304
- ISBN-13:
- 9781780406305
- Pub. Date:
- 12/13/2017
- Publisher:
- IWA Publishing
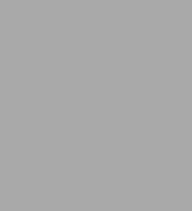
Greenhouse Gas Emission and Mitigation in Municipal Wastewater Treatment Plants
Paperback
Buy New
$98.00Overview
Product Details
ISBN-13: | 9781780406305 |
---|---|
Publisher: | IWA Publishing |
Publication date: | 12/13/2017 |
Edition description: | UK ed. |
Pages: | 160 |
Product dimensions: | 6.12(w) x 9.25(h) x 0.75(d) |
Read an Excerpt
CHAPTER 1
Warming climate and greenhouse gases
Xinmin Zhan, Guangxue Wu and Zhenhu Hu
1.1 WARMING CLIMATE
Climate change and global warming are two well-known terms all over the world, among the industry, academia, farmers, policymakers and the general public. The warming of our climate is evidenced by rising global temperatures, rising sea levels, diminishing ices in the Arctic Ocean and the Antarctic continent, retreating glaciers, and frequent extreme flood and drought events (NASA [National Aeronautics & Space Administration], 2017). The globally average temperature (combining the land and ocean surfaces) has had an increasing trend since 1880, with an increase of 0.85°C over the period of 1880–2012 (IPCC [Intergovernmental Panel on Climate Change], 2014). Even though we have implemented a number of actions to fight global warming, it is clear that the warming trend is continuing, as 15 of the 16 warmest years on record have taken place since 2001, and the year 2015 had a temperature 1°C or more higher the 1880–1899 average (NASA, 2017).
Oceans are always regarded as the conditioner maintaining the global temperature constant. Unfortunately, the ocean surface temperature also shows a warming tendency with the upper 75 m warmer by 0.11°C (0.05–0.13°C) per decade in the period 1971–2010 (IPCC, 2014). The upper 700 m of ocean water warmed from 1971 to 2010 (95–100% probability confidence) (IPCC, 2014) and the global oceanic temperature in the upper 700 m rose by 0.15°C between 1955 and 2016 (NODC [National Oceanographic Data Center], 2017).
The warming effects vary by region, with the effects being more obvious and significant in some regions than others (Figure 1.1). In Ireland, the observational data from five long stations show that the mean annual temperature increased by approximately 0.8°C over the period 1900–2012 (Gleeson et al. 2013).
Intensive scientific research has shown that the cause of the warming climate is anthropogenic greenhouse gas emissions, which have increased significantly since the pre-industrial era (IPCC, 2014): there is a 95–100% probability that the anthropogenic increase in GHG emissions has led to the global average surface temperature increase from 1951 to 2010. Between 1750 and 2011, 2040 ± 310 Gt CO2-eq (1 Gt = 109 tonnes) was emitted to the atmosphere, half of which occurred in the last 40 years, with anthropogenic GHG emissions in 2010 reaching 49 ± 4.5 Gt CO2-eq/year. Currently, the atmospheric CO2 level is 407 parts per million, while it was 280 parts per million 250 years ago, in the mid-18th century (NASA, 2017). Total annual anthropogenic GHG emissions increased sharply after 2000, by 1.0 Gt CO2-eq per year from 2000 to 2010. The annual increase was 0.4 CO2-eq per year over the period of 1970 to 2000 (Figure 1.2). This was due to the rapid economic development in emerging and developing countries, for example China, India, and Brazil.
An increasingly warming climate will damage our natural environment and human living systems. This damage will vary from region to region but will include: loss of livelihoods, infrastructure and economic stability; increased flood and drought events; increased heat-related human mortality; food-shortage and lack of food security; and reduced biodiversity of fauna and flora. Hence, all the states in the world are working together to fight the warming climate with the goal of limiting global temperature rise in the year 2100 to within 2°C of the pre-industrial temperature. In order to achieve this target, global anthropogenic GHG emissions need to be reduced by 40–70% by 2050 compared to the 2010 level, and reduced by almost 100% by 2100 (IPCC, 2014).
The three major GHG gases are carbon dioxide (CO2), methane (CH4) and nitrous oxide (N2O). They accounted for 76%, 16% and 6.2%, respectively, of total anthropogenic GHG emissions in 2010 (IPCC, 2014).
1.2 GREENHOUSE GASES AND GLOBAL WARMING POTENTIAL
Without greenhouse gases, after solar energy arrived at the Earth's surface, the heat would be radiated back into space. However, due to the presence of these gases which are spread over the Earth's surface and able to absorb the outgoing heat, some heat is trapped between the Earth's surface and the low atmosphere (Figure 1.3). This extra heat causes the temperature of the Earth's surface to rise.
In order to compare the impacts of different GHG gases on global warming, a metric, global warming potential (GWP), has been developed. GWP is defined as the amount of energy which the emission of 1 tonne of a gas will absorb over a certain period of time, normally 100 years, relative to the emission of 1 tonne of CO2. Hence, the GWP value of CO2 is 1 in 100 years, while the GWPs of CH4 and N2O are 28 and 265 over 100 years, respectively. Table 1.1 lists the GWP values for common greenhouse gases (IPCC, 2014). It should be noted that GWPs vary according to the methodology defining the impacts of future warming on the carbon cycle and the calculation methods used (USEPA [United States Environmental Protection Agency], 2017).
Based on GWP, we can use the following two equations to convert the emissions of CH4 and N2O to the equivalent emissions of CO2:
[MATHEMATICAL EXPRESSION OMITTED] (1.1)
[MATHEMATICAL EXPRESSION OMITTED] (1.2)
where, [MATHEMATICAL EXPRESSION OMITTED] and [MATHEMATICAL EXPRESSION OMITTED] are the emissions of CH4 and N2O gases respectively (tonne); and CO2-eq is the equivalent emission of CO2 (tonne).
Anthropogenic CO2 emissions are mainly due to burning of fossil fuels, and deforestation. Anthropogenic N2O emissions are due to agricultural use of chemical fertilizers, land-spreading of animal manure, and burning of biomass and fossil fuels. Anthropogenic CH4 emissions are caused by decomposition of organic matter during human activities, for example, land-spreading and storage of animal manure, rumen fermentation of ruminant livestock, and gas loss from anaerobic digesters of biowastes. They are also produced in wastewater treatment plants (WWTPs). CO2, CH4, and N2O are the main greenhouse gases generated during the wastewater treatment processes (Hendrickson et al. 2015).
1.3 GENERATION OF CO2 IN WASTEWATER TREATMENT PLANTS
CO2 is the largest contributor to greenhouse gas emissions. It is the product of the oxidation of organic matter:
C + O2 -> CO2 (1.3)
In the wastewater treatment process, CO2 is produced due to the oxidation of organic matter by microorganisms in aerobic or anaerobic conditions.
In aerobic conditions, microorganisms break down the organic matter so as to obtain energy and synthesize new cells through which organic matter is removed from wastewater:
Organic matter + O2 + nutrients (nitrogen, phosphorus, etc.) -> new cells + CO2 + H2O + ... (1.4)
If we assume that organic matter is biologically decomposed and nitrification is ignored, and use CaHbOcNd to represent organic matter, and CwHxOyNz to represent the growth of new microorganism biomass, the equation above is re-written as:
[MATHEMATICAL EXPRESSION OMITTED] (1.5)
The chemical composition of organic matter in wastewater can be represented approximately by the formula: C18H19O9N (Henze et al. 1996). If the growth of biomass and nitrification is ignored, then Eq. 1.5 is simplified to:
C18H19O9N + 17.5O2 + H+ -> 18CO2 + 8H2O + NH+4 (1.6)
In anaerobic conditions, when microorganisms degrade organic contaminants, CO2 is also produced with CH4, which will be detailed in a later section.
If chemical processes are adopted in WWTPs to breakdown organic matter, CO2 is also produced (Tchobanoglous et al. 2003):
organic matter + oxidants (Cl, O3, H2O2, etc.) -> intermediate oxygenated molecules (1.7)
intermediate oxygenated molecules + oxidants (Cl, O3, H2O2, etc.) -> simple end products (CO2, H2O, etc.) (1.8)
Since chemical oxidation processes are not commonly used in municipal WWTPs, we only need to consider CO2 production and emission in aerobic and anaerobic biological wastewater treatment processes. The IPCC excludes CO2 emission in the greenhouse gas emission inventory for WWTPs because the IPCC recognizes that CO2 emission from WWTPs is from biogenic origins and it doesn't contribute to the increase of anthropogenic CO2 concentrations in the atmosphere (Shaw & Koh, 2014). However, organic contaminants with fossil carbon origins, like surfactants, pharmaceuticals and personal care products, exist in wastewater influent into wastewater treatment plants, and account for up to 8–14% of total chemical oxygen demand (COD) concentration in a combined flow of residential and industrial wastewater, and 4–7% of COD in residential wastewater (Law et al. 2013). Hence, the CO2 emissions due to the biodegradation of these fossil origin organic contaminants should be taken into account when quantifying WWTP GHG emissions.
Indirectly, carbon dioxide is emitted in wastewater treatment processes as a result of fossil fuel combustion to meet the large energy requirement of WWTPs (Ashrafi et al. 2014).
1.4 GENERATION OF CH4 IN WASTEWATER TREATMENT PLANTS
CH4 is the second-most potent greenhouse gas after CO2 in terms of impacts on global warming. Its GWP is 28 times over a 100-year basis. The concentration of CH4 in the atmosphere has increased from 0.7 parts per million in pre-industrial times to 1.8 parts per million today (Zeebe, 2013). The trend still continues, with atmospheric CH4 concentrations increasing by approximately 7 parts per billion per year (Vollmer et al. 2015). Therefore, the mitigation of methane emission is very important when tackling global warming. Methane generation by anaerobic respiration has both natural and anthropogenic sources.
CH4 is generated by microorganisms under anaerobic conditions in sewers and WWTPs when they decompose organic matter:
Organic matter + H2O + nutrients (nitrogen, phosphorus, etc.) -> new cells + resistant organic matter + CO2 + CH4 + NH3 + H2S + ... (1.9)
The biological conversion of organic matter in wastewater to CH4 is complex and involves a series of microbial metabolic stages which occur in anaerobic conditions (Weiland, 2010). The conversion process consists of four steps: hydrolysis, acidogenesis, acetogenesis, and methanogenesis. Each step is completed by specific types of microorganisms on substrates generated from the preceding steps. This is illustrated in Figure 1.4.
Organic matter in wastewater is composed of carbohydrates (which include cellulose, hemicellulose and lignin), proteins, lipids, and other natural or synthetic compounds. Hydrolysis is the first step for the anaerobic degradation of organic matter (Hu et al. 2008). Via enzymes excreted by facultative and obligate bacteria and fungi, these organic compounds are hydrolyzed into smaller molecules, such as amino acids, fatty acids, peptides, and CO2, as well as a small amount of acetic acid and H2 (Cheng, 2009).
The second step is acidogenesis. The amino acids, fatty acids, polysaccharides, and peptides generated in the hydrolysis step are further converted to volatile fatty acids (VFAs) by acidogenic bacteria. Small amounts of acetic acid, H2, and CO2 are also produced.
The produced non-acetic acid VFAs are then converted into acetic acid, H2, and CO2. This step is acetogenesis. Acetic acid, H2 and CO2 are precursors for methane generation in the last step – methanogenesis. Acetic acid and H2/CO2 produced in the acetogenesis and previous steps are utilized by archaea to synthetize CH4. There are two different biochemical pathways for CH4 generation based on the substrates used. If acetic acid is used as the substrate, the reaction is as described in Eq. 1.10:
CH3COOH -> CH4 + CO2 (1.10)
This pathway, known as acetotrophic methanogenesis, is completed by obligate or facultative acetate-utilizing archaea. Acetotrophic methanogenesis is widely recognized as the main pathway for CH4 production. H2 and CO2 can also be used as substrates to form CH4, as described in Eq. 1.11:
4H2 + CO2 -> CH4 + 2H2O (1.11)
This pathway, hydrogenotrophic methanogenesis, is completed by obligate or facultative hydrogen-utilizing archaea.
Eq. 1.9 is simplified as:
CaHbOcNd + (d - nx)H+ -> nCwHxOyNz + mCH4 + sCO2 + rH2O + d (d - nx) NH+4 (1.12)
where s = a - nw - m, and r = c - ny - 2s.
If the anaerobic degradation process is complete, then:
[MATHEMATICAL EXPRESSION OMITTED] (1.13)
Based on Eq. 1.13, if we know the composition of the organic compounds and their amounts, the theoretical yield of CH4 from organic compounds can be predicted. If C18H19O9N is used to describe the chemical composition of municipal wastewater, then:
C18H19O9N + 9.5H2O + H+ -> 8.75CH4 + 9.25 CO2 + NH+4 (1.14)
when d = 0, namely when nitrogen is ignorable, then Eq. 1.13 is simplified to the well-known Buswell equation (Horbaj, 2004):
[MATHEMATICAL EXPRESSION OMITTED] (1.15)
1.5 N2O GENERATION IN WASTEWATER TREATMENT FACILITIES
N2O can be emitted during both nitrification and denitrification. The lifetime of N2O is about 120 years, which causes a long-term effect on global warming. Its GWP is 265 in 100 years. The atmospheric N2O concentration has been increasing at 0.73 ± 0.03 parts per billion/year over the last three decades (IPCC, 2014) and, at present, it is approximately 330 parts per billion (IPCC, 2014). Because CH4 and N2O have much shorter lifetimes than that of CO2, it is good practice to mitigate global warming by reducing non-CO2 emissions (Montzka et al. 2011). Nowadays, N2O emission receives extensive attention due to its high GWP, and should be carefully controlled from all sectors, including agricultural farming and wastewater management systems. Apart from GHG impacts, N2O in the atmosphere reacts with atomic oxygen to form NO, which induces the destruction of the stratospheric ozone (Ravishankara et al. 2009).
In the EU-27, N2O is mainly emitted in waste treatment activities (10 million tonnes CO2-eq emission), nitric acid production (27 million tonnes CO2-eq emission), and livestock farming (21 million tonnes CO2-eq emission) (López et al. 2013).
Biological nitrogen removal (BNR) in WWTPs includes nitrification and denitrification. More complicated than generation of CO2 and CH4 in WWTPs, during BNR, typically, there are three main pathways for N2O emission (Figures 1.5 and 1.6), i.e., nitrifier denitrification, hydroxylamine (NH2OH) oxidation and heterotrophic denitrification. Other pathways may also contribute to N2O emission. For instance, chemidenitrification and the nonenzymatic decomposition of nitrite can also lead to the production of N2O when there is a high concentration of nitrite, NO-2, in wastewater treatment systems (Anderson & Levine, 1986).
(Continues…)
Excerpted from "Greenhouse Gas Emission and Mitigation in Municipal Wastewater Treatment Plants"
by .
Copyright © 2018 IWA Publishing.
Excerpted by permission of IWA Publishing.
All rights reserved. No part of this excerpt may be reproduced or reprinted without permission in writing from the publisher.
Excerpts are provided by Dial-A-Book Inc. solely for the personal use of visitors to this web site.