Green Chemistry: An Introductory Text / Edition 2 available in Hardcover
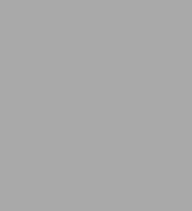
Green Chemistry: An Introductory Text / Edition 2
- ISBN-10:
- 1847558739
- ISBN-13:
- 9781847558732
- Pub. Date:
- 05/20/2010
- Publisher:
- RSC
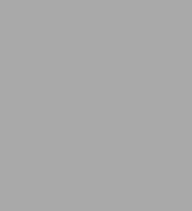
Green Chemistry: An Introductory Text / Edition 2
Hardcover
Buy New
$42.00Buy Used
$30.87-
-
SHIP THIS ITEM
Temporarily Out of Stock Online
Please check back later for updated availability.
-
Overview
Product Details
ISBN-13: | 9781847558732 |
---|---|
Publisher: | RSC |
Publication date: | 05/20/2010 |
Edition description: | Second Edition,New edition |
Pages: | 328 |
Product dimensions: | 6.40(w) x 9.30(h) x 1.10(d) |
About the Author
Read an Excerpt
Green Chemistry: An Introductory Text
By Mike Lancaster
The Royal Society of Chemistry
Copyright © 2002 The Royal Society of ChemistryAll rights reserved.
ISBN: 978-1-84755-100-9
CHAPTER 1
Principles and Concepts of Green Chemistry
1.1 INTRODUCTION
During the twentieth century chemistry changed for ever the way we live. Perhaps the greatest perceived benefits, to the general public, have come from the pharmaceuticals industry with developments of painkillers, antibiotics, heart drugs and, more recently, Viagra. However, it is difficult to think of an important facet of modern life which has not been transformed by products of the chemical and related industries, for example:
Transportation – production of gasoline and diesel from petroleum, fuel additives for greater efficiency and reduced emissions, catalytic converters, plastics to reduce vehicle weight and improve energy efficiency.
Clothing – man-made fibres such as rayon and nylon, dyes, water proofing and other surface finishing chemicals.
Sport – advanced composite materials for tennis and squash rackets, all-weather surfaces.
Safety – lightweight polycarbonate cycle helmets, fire-retardant furniture.
Food – refrigerants, packaging, containers and wraps, food processing aids, preservatives.
Medical – artificial joints, 'blood bags', anaesthetics, disinfectants, anti-cancer drugs, vaccines, dental fillings, contact lenses, contraceptives.
Office – photocopying toner, inks, printed circuit boards, liquid-crystal displays.
Home – material and dyes for carpets, plastics for TVs and mobile phones, CDs, video and audio tapes, paints, detergents.
Farming – fertilizers, pesticides.
The value of the chemical industry is shown in Figure 1.1. In the UK over 450 000 people are employed by the industry (including pharmaceuticals and plastics) and the industry is manufacturing's number one exporter.
In many countries, however, the chemical industry is often viewed, by the general public, as causing more harm than good. There are several reasons for this, including general ignorance of the end use and value of the industry's products; however, a major reason is that the industry is perceived as being polluting and causing significant environmental damage. There is a certain amount of truth in this view with well-publicized disasters such as Bhopal causing both environmental damage and loss of life. As well as specific disasters, general pollution which came to the public's attention in the 1960s and 70s through eutrophication, foaming rivers, the discovery of persistent organic pollutants and the famous 'burning' Cuyahoga river, have all played a part in formulating this view of the chemical industry.
Chemists and engineers engaged in development of chemical products and processes have never set out to cause damage to the environment or human health. These have occurred largely through a lack of knowledge, especially of the longer-term effects of products entering the environment and possibly an over-reliance on procedures to ensure operations are carried out safely. The challenge for the chemical industry in the twenty-first century is to continue to provide the benefits we have come to rely on, in an economically viable manner, but without the adverse environmental side effects.
1.2 SUSTAINABLE DEVELOPMENT AND GREEN CHEMISTRY
Current thinking on sustainable development came out of a United Nations Commission on Environment and Development in 1987 (Bruntland Commission), which defined sustainable development as: '... meeting the needs of the present without compromising the ability of future generations to meet their own needs.' Although the ideals on which sustainable development is based are not new, indeed Thomas Jefferson made similar comments in 1789, the Bruntland Commission did catalyse the sustain-ability debate. Since 1987 Governments, NGOs, society in general and industry sectors have considered what sustainable development really means and how best to start to achieve it from their own standpoint. Issues that will have a significant impact on how the move towards sustainability is approached, include time-scale, likely future technology developments and population forecasts. Two of the key aspects of sustainable development from a chemicals and energy perspective are: 'how fast should we use up fossil fuels?' and 'how much "waste" or pollution can we safely release to the environment?' Whilst there are no agreed answers to these questions there is general agreement to develop more renewable forms of energy and to reduce pollution.
The Natural Step, an international movement, started in Sweden, dedicated to helping society reduce its impact on the environment has developed four system conditions for sustainability:
Materials from the Earth's crust (e.g. heavy metals) must not systematically increase in nature.
Persistent substances produced by society (e.g. DDT, CFCs) must not systematically increase.
The physical basis for the Earth's productive natural cycles must not be systematically deteriorated.
There must be fair and efficient use of resources with respect to meeting human needs.
This approach recognizes that the Earth does have a natural capacity for dealing with much of the waste and pollution which society generates; it is only when that capacity is exceeded that our lifestyle becomes unsustainable.
During the early 1990s the US Environmental Protection Agency (EPA) coined the phrase Green Chemistry 'To promote innovative chemical technologies that reduce or eliminate the use or generation of hazardous substances in the design, manufacture and use of chemical products.' Over the last 10 years Green Chemistry has gradually become recognized as both a culture and a methodology for achieving sustainability. The 12 Principles of Green Chemistry (Box 1.1) help show how this can be achieved. Many of these 12 principles, e.g. catalysis and increased use of renewable resources, are expanded on in later chapters. When looking at Green Chemistry from an industrial perspective it is important to take the costs of implementing green technology into account; from this point of view it is helpful to look at Green Chemistry as a reduction process (Figure 1.2). From this perspective it becomes obvious that through application of Green Chemistry concepts significant savings can be made, arising from reduced raw material use, lower capital expenditure, lower costs of waste treatment and disposal, etc. The fundamental challenge for the chemical industry is to continue to provide the benefits to society without overburdening or causing damage to the environment, and all this must be done at an acceptable cost.
1.3 ATOM ECONOMY
One of the fundamental and most important principles of Green Chemistry is that of atom economy. This essentially is a measure of how many atoms of reactants end up in the final product and how many end up in byproducts or waste. The percentage atom economy can be calculated as 100 times the relative molecular mass (RMM) of all atoms used to make wanted product divided by the RMM of all reactants, Box 1.2. The real benefit of atom economy is that it can be calculated at the reaction planning stage from a balanced reaction equation. Taking the following theoretical reaction:
X + Y = P + U (1.1)
the reaction between X and Y to give product P may proceed in 100% yield with 100% selectivity but because the reaction also produces unwanted materials U its atom economy will be less than 100%.
Traditionally chemists are taught to maximize the yield of a reaction. Whilst this is a worthy goal and is an effective measure of the efficiency of a particular reaction it is not a particularly good measure for comparing efficiencies between different reactions. Taking two of the production routes for maleic anhydride as an example (Scheme 1.1), it is evident that the butene oxidation route is considerably more atom efficient and avoids 'wasting' two carbon atoms as CO2. Comparison of the two routes is interesting since both occur under similar reaction conditions of 400°C in the presence of a promoted vanadium pentoxide catalyst. Initial processes were based on benzene but for a while butene oxidation became the preferred route since it involved simpler separation technology.
Today most plants use butane as a feed stock because of the lower raw material price. Whilst, at the design stage, the choice of butene over benzene would appear obvious, the two routes do have differing selectivities, negating some of the atom economy benefits of the butene route.
Using benzene typical selectivities of around 65% are obtained commercially whilst for butene it is approximately 55%. If we multiply the theoretical atom economies by these figures we obtain practical atom economies of 28.7% for the benzene route and 35.6% for butene. This is a useful illustration of how the atom economy concept is a valuable additional tool in measuring overall reaction efficiency, and how good atom economy can compensate for poorer yields or selectivities.
By taking the atom economy of various synthetic routes into account at the planning stage the chosen strategy is likely to produce a greater weight of products per unit weight of reactants than might otherwise have been the case. There are, however, a number of common reaction types which are inherently atom efficient and a number which are not (Table 1.1). Although the reactions under the 'Atom Economic' heading are generally atom efficient, each specific reaction should be considered individually since, for example, unrecoverable 'catalysts' sometimes need to be used in significant amounts. On the other hand some atom un-economic reactions may involve, for example, elimination of water, which does not significantly detract from the greenness.
1.4 ATOM ECONOMIC REACTIONS
1.4.1 Rearrangement Reactions
Rearrangements, especially those only involving heat or a small amount of catalyst to activate the reaction, display total atom economy. A classic example of this is the Claisen rearrangement, which involves the rearrangement of aromatic allyl ethers as shown in Scheme 1.2. Although ortho-substituted products usually predominate, some para-alkylated products are also formed, reducing overall yield. The reaction may however be both high yielding and atom economic when di-ortho-substituted aromatic allyl ethers are used.
The Fries rearrangement of phenolic esters (Scheme 1.3) is normally 'catalysed' by stoichiometric amounts of a Lewis acid such as AlCl3. The requirement for this large amount of 'catalyst' is due to it complexing with the product; work-up with water hydrolyses the complex producing copious amounts of aluminium waste. This significantly reduces the atom economy of the reaction as the AlCl3 should be considered a reagent rather than a catalyst since it is not recovered in a reusable form.
A useful solution to this problem is the photo Fries rearrangement. Here UV light is used to generate RCOO radicals and the reaction proceeds via an intermolecular free radical route rather than through nucleophilic attack as in the conventional process. The selectivity of these reactions may be improved by imposing steric control through carrying out the reactions in zeolite or cyclodextrin cages.
Another valuable rearrangement reaction that is usually 'catalysed' by stoichiometric amounts of catalyst is the Beckmann rearrangement (Scheme 1.4). This reaction is used commercially for converting cyclohexanone oxime into caprolactam, a key intermediate for nylon 6; 20% oleum is the usual catalyst. A wide range of heterogeneous catalysts have been explored, which avoid the need for using oleum. In particular, certain zeolites, notably [B]-MFI, have been shown to give excellent yields (up to 94% in fluidized-bed reactors), and good stability and ease of regeneration.
One other 100% atom economic rearrangement is worth mentioning briefly. When vinylcyclopropanes are heated they readily undergo ring expansion to cyclopentenes. The temperature required varies significantly, depending on the molecule; for example 1-phenyl-2-vinylcyclopropane may be converted to phenylcyclopentene in reasonable yield at 200°C.
Many other versatile so-called rearrangements involve elimination of water. Whilst this does reduce the atom economy these reactions are worth considering when devizing a green synthesis. The pinacol rearrangement of vic diols into aldehydes or ketones is catalysed by acids: frequently sulfuric acid is used, but there are also many examples of supported acid catalysts such as FeCl3 on silica being used. The mechanism involves protonation of one of the hydroxyl groups followed by loss of water, alkyl migration to give the more stable carbocation and final regeneration of the proton (Scheme 1.5). All compounds in which a carbocation can be generated α to a carbon atom bearing a hydroxyl group may undergo similar rearrangements.
1.4.2 Addition Reactions
As the name suggests, these reactions involve addition of a regent to an unsaturated group and as such nominally display 100% atom economy.
A=A + B-C [right arrow] AB-AC (1.2)
When the addition is initiated by attack of the π-electrons in an unsaturated bond on an electrophile to form a carbocation the reaction is an electrophilic addition, a very common class of reactions for alkenes. The reaction is governed by Markovnikov's rule, which states that in addition of HX to a substituted alkene, the H will form a bond to the carbon of the alkene carrying the greater number of hydrogen atoms. Another way of expressing this is that the most stable carbocation will be formed (Scheme 1.6).
The Michael reaction involving addition to carbon–carbon double bonds containing an electron-withdrawing group is catalysed by base. A variety of heterogeneous bases which can be reused are known, including alumina, KF on alumina and phenolates supported on silica, the last having proved particularly effective for addition of β-keto esters to enones (Scheme 1.7).
Addition reactions to carbonyl groups are also important atom economic reactions. By using chiral ligands (see Chapter 4) catalytic hydrogenation can be carried out enantioselectively, adding to the overall greenness. This technology is becoming increasingly used in the pharmaceutical industry; for example S-naproxen (Scheme 1.8) can be made in high entioselectivity using chiral ruthenium phosphine catalyst. Diels–Alder reactions provide one of the few general methods of forming two carbon–carbon bonds simultaneously. The main features of these reactions are described in Box 1.3. The reaction finds widespread industrial use; for example hardeners for epoxy resins are made by reaction of maleic anhydride with dienes such as 2-methyl-1,4-butadiene.
An atom economic route to the insecticide aldrin (Scheme 1.9) was developed some fifty years ago. This very potent insecticide was later banned in most countries owing to its toxicity to wild life. This example illustrates the need to look at the whole product lifecycle, not just the synthetic route.
1.5 ATOM UN-ECONOMIC REACTIONS
1.5.1 Substitution Reactions
Substitutions are very common synthetic reactions; by their very nature they produce at least two products, one of which is commonly not wanted. As a simple example 2-chloro-2-methylpropane can be prepared in high yield by simply mixing 2-methylpropan-2-ol with concentrated hydrochloric acid (Scheme 1.10). Here the hydroxyl group on the alcohol is substituted by a chloride group in a facile SN1 reaction. Whilst the byproduct in this particular reaction is only water it does reduce the atom economy to 83%.
Most substitutions have lower atom economies than this and produce more hazardous and a greater variety of by-products. Hexanol is much less reactive than 2-methylpropan-2-ol in substitution reactions; one way of converting this to the chloride involves reaction with thionyl chloride (Scheme 1.11); here the unwanted by-products are HCl and SO2 reducing the overall atom economy to 55%. This readily illustrates how, even in simple reactions, half of the valuable atoms in a reaction can be lost as waste.
For many years phenol was made on a large industrial scale from the substitution reaction of benzene sulfonic acid with sodium hydroxide. This produced sodium sulfite as a by-product. Production and disposal of this material, contaminated with aromatic compounds, on a large scale contributed to the poor economics of the process, which has now been replaced by the much more atom economic cumene route (see Chapter 2, Schemes 2.2 and 2.3).
1.5.2 Elimination Reactions
Elimination reactions involve loss of two substituents from adjacent atoms; as a result unsaturation is introduced. In many instances additional reagents are required to cause the elimination to occur, reducing the overall atom economy still further. A simple example of this is the E2 elimination of HBr from 2-bromopropane using potassium t-butoxide (Scheme 1.12). In this case unwanted potassium bromide and t-butanol are also produced reducing the atom economy to a low 17%.
(Continues...)
Excerpted from Green Chemistry: An Introductory Text by Mike Lancaster. Copyright © 2002 The Royal Society of Chemistry. Excerpted by permission of The Royal Society of Chemistry.
All rights reserved. No part of this excerpt may be reproduced or reprinted without permission in writing from the publisher.
Excerpts are provided by Dial-A-Book Inc. solely for the personal use of visitors to this web site.
Table of Contents
Chapter 1 Principles and Concepts of Green Chemistry 1
1.1 Introduction 1
1.2 Sustainable Development and Green Chemistry 3
1.2.1 Green Engineering 4
1.3 Atom Economy 7
1.4 Atom Economic Reactions 9
1.4.1 Rearrangement Reactions 9
1.4.2 Addition Reactions 11
1.5 Atom Un-economic Reactions 13
1.5.1 Substitution Reactions 13
1.5.2 Elimination Reactions 15
1.5.3 Wittig Reactions 16
1.6 Reducing Toxicity 17
1.6.1 Measuring Toxicity 19
Review Questions 21
Further Reading 22
Chapter 2 Waste: Production, Problems, and Prevention 23
2.1 Introduction 23
2.2 Some Problems Caused by Waste 25
2.3 Sources of Waste from the Chemical Industry 26
2.4 Cost of Waste 29
2.5 Waste Minimization Techniques 33
2.5.1 The Team Approach to Waste Minimization 34
2.5.2 Process Design for Waste Minimization 36
2.5.3 Minimizing Waste from Existing Processes 38
2.6 On-site Waste Treatment 39
2.6.1 Physical Treatment 41
2.6.2 Chemical Treatment 42
2.6.3 Biotreatment Plants 46
2.7 Design for Degradation 47
2.7.1 Degradation and Surfactants 48
2.7.2 DDT 49
2.7.3 Polymers 50
2.7.4 Some Rules for Degradation 51
2.8 Polymer Recycling 52
2.8.1 Separation and Sorting 53
2.8.2 Incineration 55
2.8.3 Mechanical Recycling 56
2.8.4 Chemical Recycling to Monomers 57
Review Questions 60
Further Reading 60
Chapter 3 Measuring and Controlling Environmental Performance 61
3.1 The Importance of Measurement 61
3.1.1 Lactic Acid Production 62
3.1.2 Safer Gasoline 65
3.2 Introduction to Life Cycle Assessment 66
3.2.1 Four Stages of LCA 68
3.2.2 Carbon Footprinting 72
3.3 Green Process Metrics 74
3.4 Environmental Management Systems (EMS) 77
3.4.1 ISO 14001 77
3.4.2 The European Eco-Management and Audit Scheme (EMAS) 81
3.5 Eco-Labels 82
3.6 Legislation 83
3.6.1 Integrated Pollution Prevention and Control (IPPC) 84
3.6.2 Reach 87
Review Questions 88
Further Reading 89
Chapter 4 Catalysis and Green Chemistry 90
4.1 Introduction to Catalysis 90
4.1.1 Comparison of Catalyst Types 92
4.2 Heterogeneous Catalysts 94
4.2.1 Basics of Heterogeneous Catalysis 94
4.2.2 Zeolites and the Bulk Chemical Industry 97
4.2.3 Heterogeneous Catalysis in the Fine Chemical and Pharmaceutical Industries 105
4.2.4 Catalytic Converters 114
4.3 Homogeneous Catalysts 117
4.3.1 Transition Metal Catalysts with Phosphine or Carbonyl Ligands 117
4.3.2 Greener Lewis Acids 121
4.3.3 Asymmetric Catalysis 122
4.4 Phase Transfer Catalysis 128
4.4.1 Hazard Reduction 129
4.4.2 C-C Bond Formation 130
4.4.3 Oxidation using Hydrogen Peroxide 131
4.5 Biocatalysis 132
4.6 Photocatalysis 135
4.7 Conclusions 137
Review Questions 137
Further Reading 138
Chapter 5 Organic Solvents: Environmentally Benign Solutions 139
5.1 Organic Solvents and Volatile Organic Compounds 139
5.2 Solvent-free Systems 141
5.3 Supercritical Fluids 144
5.3.1 Supercritical Carbon Dioxide (scCO2) 146
5.3.2 Supercritical Water 156
5.4 Water as a Reaction Solvent 157
5.4.1 Water Based Coatings 162
5.5 Ionic Liquids 163
5.5.1 Ionic Liquids as Catalysts 165
5.5.2 Ionic Liquids as Solvents 166
5.6 Fluorous Biphase Solvents 170
5.7 Comparing Greenness of Solvents 172
5.8 Conclusions 173
Review Questions 174
Further Reading 174
Chapter 6 Renewable Resources 175
6.1 Biomass as a Renewable Resource 175
6.2 Energy 175
6.2.1 Fossil Fuels 175
6.2.2 Energy from Biomass 179
6.2.3 Solar Power 186
6.2.4 Other Forms of Renewable Energy 188
6.2.5 Fuel Cells 189
6.3 Chemicals from Renewable Feedstocks 194
6.3.1 Chemicals from Fatly Acids 196
6.3.2 Polymers from Renewable Resources 204
6.3.3 Some Other Chemicals from Natural Resources 210
6.4 Alternative Economies 215
6.4.1 Syngas Economy 216
6.4.2 Hydrogen Economy 217
6.5 Biorefinery 218
6.6 Conclusions 219
Review Questions 220
Further Reading 220
Chapter 7 Emerging Greener Technologies and Alternative Energy Sources 221
7.1 Design for Energy Efficiency 221
7.2 Photochemical Reactions 224
7.2.1 Advantages of and Challenges Faced by Photochemical Processes 225
7.2.2 Examples of Photochemical Reactions 227
7.3 Chemistry using Microwaves 231
7.3.1 Microwave Heating 231
7.3.2 Microwave-assisted Reactions 232
7.4 Sonochemistry 236
7.4.1 Sonochemistry and Green Chemistry 237
7.5 Electrochemical Synthesis 239
7.5.1 Examples of Electrochemical Synthesis 241
7.6 Conclusions 244
Review Questions 244
Further Reading 245
Chapter 8 Designing Greener Processes 246
8.1 Introduction 246
8.2 Conventional Reactors 247
8.2.1 Batch Reactors 247
8.2.2 Continuous Reactors 250
8.3 Inherently Safer Design 252
8.3.1 Minimization 254
8.3.2 Simplification 255
8.3.3 Substitution 255
8.3.4 Moderation 256
8.3.5 Limitation 257
8.4 Process Intensification 258
8.4.1 Some PI Equipment 260
8.4.2 Some Example of Intensified Processes 263
8.5 In-process Monitoring 266
8.5.1 Near-infrared Spectroscopy 268
8.6 Process Safety 269
Review Questions 270
Further Reading 270
Chapter 9 Industrial Case Studies 271
9.1 Introduction 271
9.2 Methyl Methacrylate 271
9.3 Greening of Acetic Acid Manufacture 273
9.4 EPDM Rubbers 277
9.5 Vitamin C 280
9.6 Leather Manufacture 282
9.6.1 Tanning 284
9.6.2 Fatliquoring 288
9.7 Dyeing to be Green 288
9.7.1 Some Manufacturing Improvements 289
9.7.2 Dye Application 292
9.8 Polyethylene 293
9.8.1 Radical Process 293
9.8.2 Ziegler-Natta Catalysis 294
9.8.3 Metallocene Catalysis 295
9.8.4 Post Metallocene Catalysts 297
9.9 Eco-friendly Pesticides 298
9.9.1 Insecticides 298
9.10 Epichlorohydrin 301
Review Questions 302
Chapter 10 The Future's Green: An Integrated Approach to a Greener Chemical Industry 304
10.1 Society and Sustainability 304
10.2 Barriers & Drivers 305
10.3 Role of Legislation 307
10.4 Green Chemical Supply Strategies 309
10.5 Greener Energy 311
10.6 Conclusions 312
Review Questions 312
Further Reading 313
Subject Index 314