Dendritic Cell Interactions with Bacteria available in Hardcover
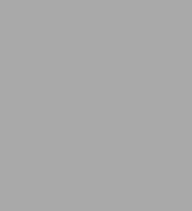
- ISBN-10:
- 0521855861
- ISBN-13:
- 9780521855860
- Pub. Date:
- 04/19/2007
- Publisher:
- Cambridge University Press
- ISBN-10:
- 0521855861
- ISBN-13:
- 9780521855860
- Pub. Date:
- 04/19/2007
- Publisher:
- Cambridge University Press
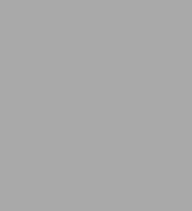
Hardcover
Buy New
$140.00Overview
Product Details
ISBN-13: | 9780521855860 |
---|---|
Publisher: | Cambridge University Press |
Publication date: | 04/19/2007 |
Series: | Advances in Molecular and Cellular Microbiology , #14 |
Pages: | 266 |
Product dimensions: | 6.30(w) x 9.25(h) x 0.79(d) |
About the Author
Read an Excerpt
Cambridge University Press
978-0-521-85586-0 - Dendritic Cell Interactions with Bacteria - by Maria Rescigno
Excerpt
PART I Dendritic cells and their role in immunity
CHAPTER 1
Subpopulations and differentiation of mouse dendritic cells
Carlos Ardavín
Universidad Autónoma
1.1 DENDRITIC CELL SUBPOPULATIONS
Dendritic cells (DCs) have an essential function in the immune system by participating in primitive defense responses that constitute the innate immunity, as well as in the induction and regulation of antigen-specific immune responses. This allows DCs to control infections caused by parasitic and microbial pathogens, to block tumour growth and to exert a precise regulation of T cell, B cell and NK cell immune responses. In addition, DCs also fulfill a pivotal role in the induction and maintenance of T cell tolerance. The functional diversity characterizing the DC system relies essentially on the remarkable plasticity of the DC differentiation process, which dictates the acquisition of DC functional specialization through the generation of a large collection of DC subpopulations (reviewed by Shortman and Liu, 2002). Dendritic cells are located both in the lymphoid organs (such as the spleen or the lymph nodes), and in non-lymphoid tissues (such as the skin or the liver), and can be classified in two major categories: conventional DCs (cDCs), and plasmacytoid DCs (pDCs). Whereas in turn cDCs comprise multiple DC subpopulationsendowed with specific functions, little is known about the functional heterogeneity of pDCs. A summary of the most relevant phenotypic and functional characteristics of the main DC subpopulations present in mice is shown in Table 1.1.
A first group of cDCs includes those that are common, and largely restricted, to the majority of organized lymphoid organs of the immune system, and perform their specific functions, as immature or mature DCs, within these organs. This group of lymphoid organ-restricted cDCs comprises three main DC subpopulations, namely CD8+ CD11b− DCs (herein called CD8+ DCs), CD8− CD11b+ DCs (herein called CD8− DCs), and CD8− CD11b− DCs (herein called CD11b− DCs). CD8− DCs
Table 1.1 Location and phenotypic characteristics of the principal mouse DC subpopulations
pDCs | CD8+DCs | CD8− CD4+DCs | CD8− CD4−DCs | CD11b−DCs | Langerhans cells | Dermal DCs | |||
---|---|---|---|---|---|---|---|---|---|
Location | |||||||||
Thymus | Yes | Yes | No | Yesa | Few | No | No | ||
Spleen | Yes | Yes | Yes | Yes | No | No | No | ||
Skin | b | No | No | No | No | Yes | Yes | ||
Peripheral LNs | Yes | Yes | Few | Yes | Few | Yes | Yes | ||
Intestinal Peyer’s patches | Yes | Yes | Few | Yes | Yes | No | No | ||
Mesenteric LNs | Yes | Yes | Few | Yes | Yes | No | c | ||
Other locations | Bone marrow, blood, lung | Liver | Liver | Liver | Epidermis, intestinal S-E, lung S-E, vagina S-E | Intestinal L-P,d bronchial L-P,d lung interstitium,d liver parenchyma,d kidney parenchyma,d CNS parenchyma | |||
Phenotype | |||||||||
CD11c | + int | + high | + high | + high | + high | + high | + high | ||
CD11b | − | + low | + high | + high | − | + high | + high | ||
CD8 | +e | + | − | − | − | − (↠ +)f | − | ||
CD4 | +e | − | + | − | − | − | − | ||
B220 | + | − | − | − | − | − | − | ||
CD62L | + | − | − | − | Not analyzed | − | − | ||
DEC-205 | − | + high | − | − | − | + high | + low | ||
Abbreviations: CNS, central nervous system; LNs, lymph nodes; L-P, lamina propria; S-E, stratified epithelium.
Notes
aThymic CD8− DCs have been reported to express low levels of CD4, although it has been suggested that in fact CD8− thymic DCs are CD4−, since CD4 appears to be picked up by thymic DCs from surrounding CD4+ T cells.
bpDCs are absent from the skin in steady state, but can be recruited to this location during inflammation.
cDCs with similar characteristics than those defining dermal DCs after migration to the peripheral LNs are found in the mesenteric LNs; these cells have been claimed to correspond to intestinal L-P DCs.
dInterstitial DCs that have been suggested to be functionally related to dermal DCs have been described in these locations.
epDCs have been demonstrated to upregulate both CD8 and CD4 during activation.
fLangerhans cells have been demonstrated to upregulate CD8 during in vivo maturation and migration from the skin to the draining lymph nodes.
can be in turn subdivided in CD8− CD4+ DCs and CD8− CD4− DCs. Whereas CD8+ DCs and/or CD8− DCs are present in the thymus, spleen, lymph nodes and lymphoid tissue of the intestinal and respiratory tracts (reviewed by Shortman and Liu, 2002), the CD11b− DC subpopulation appears to be predominantly related to the intestinal lymphoid system (reviewed by Johansson and Kelsall, 2005), representing approximately one-third of the DCs found in the Peyer's patches and mesenteric lymph nodes. CD8+ DCs and CD8− DCs do not appear to migrate or recirculate to other effector organs of the immune system to fulfill their functions. In this sense, CD11b− DCs have been tentatively included in this category since they appear to be present in the majority of lymphoid organs (reviewed by Johansson and Kelsall, 2005), but their immunobiology, and particularly their migratory behavior, remains largely unknown.
A second group of cDCs comprises those located, in an immature state, in antigen-uptake sites within non-lymphoid organs. Upon contact with an antigen, these DCs migrate through the lymph vessels to the lymph nodes, where they interact with antigen-specific T cells, and in some cases with other effector cells of the immune system, including DCs, NK cells and B cells. This group of migrating-cDCs comprises Langerhans cells (located in the epidermis, and other stratified and pseudo-stratified epithelia of the intestinal, respiratory and reproductive tracts), and interstitial DCs. These in turn include dermal DCs, and other interstitial DC subpopulations, as those found in the lamina propria of the intestinal and respiratory tracts, as well as those located in the lung interstitium, and in the parenchyma of the liver, kidney and CNS.
A number of experimental evidences suggest that in the steady state both lymphoid-organ restricted cDCs and migrating-cDCs are generated locally from blood-borne, immediate DC precursors, originating in the bone marrow (reviewed by Ardavín, 2003). Interestingly, during ongoing immune responses, a strong increase in the absolute number of both lymphoid organ-restricted cDCs and migrating-cDCs has been reported, raising the problem of the identity of the immediate DC precursors responsible for the generation of these de novo-formed DCs. It could be hypothesized that the same precursors are responsible for the generation of cDCs under steady state and infection. Alternatively, during infection, apart from, or instead of the precursors functioning in steady state, additional inflammatory precursors, with equivalent or complementary DC differentiation potential, could be recruited. Although this remains an open issue in DC biology, different research groups have reported that during inflammation and/or infection, monocytes, which represent so far the best known DC precursors, could be recruited to both peripheral tissues (such as the dermis) and to lymphoid organs, and differentiate locally into DCs (reviewed by Leon et al., 2005). The relationships between monocyte-derived DCs (or other putative de novo-formed inflammatory DCs) and the pre-existing lymphoid organ-restricted cDCs or migrating-cDC subpopulations, has to be addressed. In this sense, data from our laboratory suggest that during infection with Leishmania major, monocytes are actively recruited to the popliteal lymph nodes draining the site of infection, where they differentiate into CD11b+ CD8− DCs, with phenotypic characteristics that differ from those defining the DC subpopulations pre-existing in the popliteal lymph nodes (C. Ardavín et al., unpublished data). Although the role played by monocyte-derived DCs during Leishmania infection has to be defined, our results support the concept that the immune responses against certain pathogens could involve the participation of newly-formed DCs endowed with specific functions.
In contrast to cDCs, pDCs appear to differentiate in the bone marrow, and migrate to the blood and lymphoid organs, where they are activated and participate in anti-viral immune responses (reviewed by Colonna et al., 2004). In addition, it has been shown that pDCs can be recruited to the inflamed skin during atopic dermatitis, contact dermatitis or psoriasis, and to systemic lupus erythematosis (SLE)-associated skin lesions (reviewed by Valladeau and Saeland, 2005). However, the possibility that during inflammation and/or infection pDC precursors are recruited to the lymphoid organs and differentiate locally, has not been addressed in depth and therefore cannot be excluded.
An integrated model of the development and function of the mouse DC system is represented in Figure 1.1.
1.1.1 Lymphoid organ-restricted cDCs
In the steady state, CD8+ DCs, CD8− DCs and CD11b− DCs are found in the lymphoid organs as immature DCs; however, under situations of inflammation and/or infection DCs enter into a maturation program as a result of the engagement of DC activating receptors (reviewed in Johansson and Kelsall, 2005 and Villadangos and Heath, 2005). Globally, these DC subpopulations are considered to induce T cell responses after capturing antigens that gain access to the lymphoid organs through the blood or lymph vessels. In addition, as discussed below, at least CD8+ DCs can also cross-present antigens captured in the vicinity of epithelial surfaces by migrating DCs
Image not available in HTML version |
that transport these antigens to the lymph nodes and transfer them to CD8+ DCs (Belz et al., 2004).
Maturation of lymphoid organ-restricted DCs could involve a local migration process, from the antigen capture zones to the T cell areas within the same lymphoid organ, but not the migration between different organs through the lymphatics. In particular, it has been reported that upon activation, CD8− DCs migrate from the outer to the inner part of the splenic white pulp (De Smedt et al., 1996; Reis e Sousa et al., 1997), and from the subepithelial dome to the interfollicular region of the Peyer's patches (Iwasaki and Kelsall, 2000). In general, antigen presentation by immature CD8+ DCs or CD8− DCs leads to T cell tolerance, whereas antigen uptake by these DC subpopulations in the presence of maturation stimuli leads to the induction of T cell immunity by mature CD8+ DCs or CD8− DCs (reviewed by Steinman and Nussenzweig, 2002).
CD8+ DCs
In steady state, CD8+ DCs are located in the cortico-medullary zone and medulla of the thymus (reviewed by Ardavín, 1997) (where they participate in the negative selection of the developing thymocytes) and in the T cell areas of the lymphoid organs (reviewed by Villadangos and Heath, 2005) (i.e. inner part of the splenic white pulp, deep cortex of the lymph nodes, and interfollicular regions of the lymphoid aggregates of the intestinal tract). CD8+ DCs are less efficient than CD8− DCs in capturing antigens by endocytosis or phagocytosis (Leenen et al., 1998). However, they display a high capacity to internalize apoptotic cells (Iyoda et al., 2002), which has been correlated with their ability to induce peripheral tolerance to self tissue-associated antigens in the steady state (Belz et al., 2002; Liu et al., 2002). CD8+ DCs can stimulate CD4+ or CD8+ T cell responses by direct- or cross-priming, after antigen uptake in the lymphoid organs (reviewed by Villadangos and Heath, 2005). Alternatively, it has been proposed that after antigen uptake in non-lymphoid organs by dermal or interstitial DCs, which subsequently migrate to the lymph nodes, these antigens can be cross-presented by lymph node CD8+ DCs, as the result of antigen transfer from the migrating-DCs to resident CD8+ DCs (reviewed by Villadangos and Heath, 2005). In this regard, results from various research groups agree on the idea that CD8+ DCs are the most effective, if not the sole, DC subpopulation capable of stimulating a T cell response by cross-presentation in vivo (den Haan et al., 2000; Pooley et al., 2001), although it has been recently reported that Langerhans cells can stimulate OVA-specific T cells in vivo by cross-priming (Mayerova et al., 2004). The cross-priming capacity of CD8+ DCs has been proposed to rely on their potential to process exogenous antigens to direct them to the MHC I pathway (den Haan et al., 2000; Pooley et al., 2001) and/or on the ability of this DC subset to internalize cell-associated antigens (Schulz and Reis e Sousa, 2002).
Data derived from different experimental systems support the concept that CD8+ DCs are efficient producers of interleukin (IL)-12, and are mainly involved in the induction of Th1 responses (reviewed in Iwasaki and Medzhitov, 2004). In this sense, it has been proposed that CD8+ DCs play an essential role in the induction of CD8+ T cell responses, particularly during infection by both cytolytic and noncytolytic viruses (Belz et al., 2004, 2005), although recent data indicate that they are also involved in the initiation of T cell immunity against intracellular bacteria (Belz et al., 2005). On the other hand, CD8+ DC involvement in the induction of anti-protozoan parasite immunity is controversial. Whereas CD8+ DCs have been demonstrated to play an essential role in the induction of Th1 responses against Toxoplasma gondii (Aliberti et al., 2000), they do not appear to participate in anti-Leishmania major Th1 protective responses (Filippi et al., 2003; Ritter et al., 2004).
CD8− DCs
In contrast to CD8+ DCs, CD8− DCs appear to be mainly located adjacent to the principal antigen entry zones of the lymphoid organs, i.e. the outer part of the splenic white pulp in association with the marginal zone, the subsinusal layer beneath the lymph node subcapsular sinus and the subepithelial area of the lymphoid tissue of the intestinal tract (reviewed by Villadangos and Heath, 2005). In the spleen, around two-thirds of CD8− DCs are CD4+, whereas in the Peyer`s patches and both in the peripheral and mesenteric lymph nodes the vast majority of CD8− DCs correspond to the CD4− subset (reviewed by Johansson and Kelsall, 2005). However, it has been reported that during infection by the mouse mammary tumor virus, there was a progressive increase in the proportion of CD4+ DCs among the CD8− DC subpopulation located in the popliteal lymph nodes draining the infection area (Martin et al., 2002).
CD8− DCs display a high endocytic and phagocytic capacity, but are inefficient in capturing apoptotic cells. In this sense, it has been reported that CD8− DCs are responsible for the in vivo stimulation of CD4+ T cells after immunization with antigen-loaded DC-derived exosomes (Thery et al., 2002). Data on the role of CD8− DCs in the induction of T cell responses in vivo are limited, and in particular those addressing differentially the function of CD8− CD4+ DCs versus CD8− CD4− DCs. Globally, results derived from in vivo, but principally in vitro experiments performed with splenic CD8− DCs support the concept that, in contrast to their CD8+ counterparts, CD8− DCs appear to be poor IL-12 producers, and would be mainly involved in the induction of Th2 responses (reviewed in Iwasaki and Medzhitov, 2004). In addition, CD8− DCs from the Peyer's patches (belonging to the CD4− subset) have been demonstrated to produce high amounts of IL-10, and to induce Th2 responses leading to IgA production (Iwasaki and Kelsall, 1999; Sato et al., 2003). Moreover, these cells can also induce the differentiation of IL-10 producing T regulatory cells (Iwasaki and Kelsall, 2001). However the Th1 versus Th2-polarizing potential of CD8− DCs remains controversial, and therefore needs to be addressed carefully, because it has been reported that CD8− DCs can produce IL-12 in the presence of anti-IL-10 (Fallarino et al., 2002; Maldonado-Lopez et al., 2001) in response to α-galactosylceramide (Fujii et al., 2003) and Toll-like receptors (TLR)-7 ligands (Edwards et al., 2003).
© Cambridge University Press