Biotic Interactions in the Tropics: Their Role in the Maintenance of Species Diversity available in Hardcover, Paperback
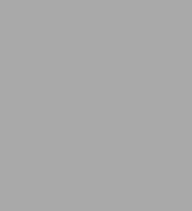
Biotic Interactions in the Tropics: Their Role in the Maintenance of Species Diversity
- ISBN-10:
- 0521847079
- ISBN-13:
- 9780521847070
- Pub. Date:
- 09/08/2005
- Publisher:
- Cambridge University Press
- ISBN-10:
- 0521847079
- ISBN-13:
- 9780521847070
- Pub. Date:
- 09/08/2005
- Publisher:
- Cambridge University Press
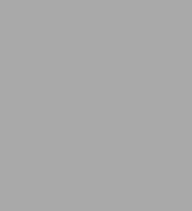
Biotic Interactions in the Tropics: Their Role in the Maintenance of Species Diversity
Buy New
$221.00Overview
Product Details
ISBN-13: | 9780521847070 |
---|---|
Publisher: | Cambridge University Press |
Publication date: | 09/08/2005 |
Series: | Ecological Reviews |
Pages: | 582 |
Product dimensions: | 6.69(w) x 9.61(h) x 1.26(d) |
About the Author
Michelle Pinard is Lecturer in Tropical Forestry at the University of Aberdeen. Her research focuses on sites in Ghana, Ethiopia, Malaysia, Sri Lanka, Brazil and Bolivia.
Sue Hartley is Reader in Ecology at the University of Sussex, specialising in the study of plant-animal interactions.
Read an Excerpt
Cambridge University Press
0521847079 - Biotic Interactions in the Tropics - Their Role in the Maintenance of Species Diversity - Edited by David F. R. P. Burslem, Michelle A. Pinard and Sue E. Hartley
Excerpt
PART 1
Plant-plant interactions
CHAPTER ONE
Plant-plant interactions in tropical forests
JOHN J. EWEL
US Forest Service
ANKILA J. HIREMATH
ATREE, Bangalore
Introduction
Some interactions between plants are uniquely conspicuous elements of certain tropical forests; the giant lianas that wend through the canopy and the epiphyte-laden branches of cloud forests are striking examples. Nevertheless, the fundamental processes involved are no different from those in extra-tropical communities, even though diverse, sometimes uniquely tropical, mechanisms may be involved. An individual of one plant species interacting with an individual of a second plant species can lead to any of the same five outcomes at any latitude, and these consist of all combinations of negative, positive and neutral effects (except the non-interaction described by the mutually neutral interaction, 0/0). But interactions among plants in forests seldom involve such simple one-on-one relationships. More commonly, multiple players are involved and the interactions change with time: the liana binds crowns of several trees, the fallen palm frond damages multiple seedlings, and the solum is shared by roots of many species. Furthermore, positive and negative interactions occur simultaneously, so the observer sees only an integrated net effect of multiple interactions ( Holmgren et al. 1997).
Most symbiotic (mutually positive) interactions in tropical forests involve relationships between plants and animals or between plants and microbes - fungi, bacteria, algae - described elsewhere in this volume. What, if anything, distinguishes plant-plant interactions from plant-microbe, plant-animal or animal-animal interactions? Even though they employ different biotic services (pollination, dispersal; see Ghazoul, Chapter 10, this volume; Muller-Landau & Hardesty, Chapter 11, this volume), higher plants (with a handful of exceptions) all use the same abiotic resources: water, carbon dioxide, photosynthetically active solar radiation and the same suite of 13 mineral elements. Furthermore, the sporophyte is immobile, precluding spatial shifts to accommodate changing conditions. Not surprisingly, then, most plant-plant interactions result in negative net impacts on at least one of the players as they compete in place for a common set of resources. Although recent years have witnessed a resurgence of interest in cooperative plant-plant interactions that benefit both participants, most examples concern physically harsh environments and are related to disturbance (Bertness & Callaway 1994).
Identification of interactions is relatively straightforward, but quantitative assessment of their ramifications is much more difficult. The approach we have used to assess consequences of interactions involved construction and 12 years of observation of communities on a fertile soil in the humid lowlands of Costa Rica. The experimental communities contain few species, representative of the life forms that have proven most evolutionarily successful in forests of the humid tropical lowlands. Unlike most studies of interspecific plant interactions, ours involve perennial species having different stature at maturity. Fast growth gives quick results, long-term observations provide an opportunity to assess changes, and perennial plants representative of successful life forms in natural forest give a semblance of real-world relevance. Three tree species (Hyeronima alchorneoides, Cedrela odorata and Cordia alliodora) are grown in monoculture and together with two monocots, one a perennial giant herb (Heliconia imbricata) and the other a palm (Euterpe oleracea) (Fig. ). We and our colleagues have used this approach to assess invasibility (Gerwing 1995; Hummel 2000; Merwin et al. 2003), productivity (Haggar & Ewel 1994; 1995; 1997), stand structure (Ewel & Bigelow 1996; Menalled et al. 1998; Kelty 2000), and nutrient use (Ewel & Hiremath 1998; Heneghan et al. 1999; Hiremath 2000; Hiremath & Ewel 2001; Hiremath et al. 2002; Reich et al. 2003; Bigelow et al. 2004; Russell et al. 2004), in addition to a number of topics involving abiotic factors or intertrophic interactions.
In this chapter we restrict consideration to interactions among established plants - seedlings (or sporlings) through adults. First, we briefly review the mechanisms involved in some prominent, tropical plant-plant interactions, both physical and biogeochemical. Next, drawing primarily on 12 years of research using the experimental communities of low life-form diversity, we turn to manifestations of plant-plant interactions at the stand level, particularly the consequences of using a common resource base. We assess the results of resource use and partitioning in terms of competition, compensation and complementarity, and we consider their implications for ecosystem functioning. We then turn to the often-ignored temporal dynamics of plant interactions, particularly resource use, and discuss time-dependent changes in plant-plant relationships as they affect individuals, communities and systems of the future. Overall findings are then interpreted in terms of implications - positive, neutral, and negative - for the maintenance, restoration and management of tropical ecosystems.
Figure 1.1 Three-life-form community at age 8 years, with tree overstorey (Cedrela odorata, in this example), palm mid-storey (Euterpe oleracea) and perennial-herb understorey (Heliconia imbricata). La Selva Biological Station, in the humid Atlantic lowlands of Costa Rica.
Image not available in HTML version |
Mechanisms
Physical interactions
Perhaps second only to the striking diversity of tropical forests, physical interactions among plants are one of the most conspicuous features of tropical forests: the fallen tree trunk that leads to a row of seedlings germinating upon it, the right-angle bend in the stem of a treelet temporarily flattened years ago by a fallen palm frond, the tree crowns festooned with epiphytes, the tangles of lianas using one another as paths to the canopy, and crown shyness due to abrasion. At one extreme, a plant can be a passive participant. For example, an important yet easily overlooked interaction among plants is detritus-fall from large individuals that breaks, bends or kills smaller ones. Although this can occur in any forest, it is an especially important phenomenon in fast-turnover tropical forests. The agent may be branches or fronds, a rain of heavy detritus that damages an average of 10 to 20 per cent of a given cohort of seedlings (Aide 1987; Clark & Clark 1991; Mack 1998; Drake & Pratt 2001) and therefore can affect future composition. At the other extreme, some interactions involve intricate mechanisms and processes, illustrative of the potential of plant evolution. Tropical forests are rich with examples, such as relationships between hemiparasites and their hosts.
In some plant-plant interactions, one member is a passive player in an interaction that is beneficial to the second member. The protection from direct sunlight afforded by a tall plant to a shorter one is an example. Although solar radiation commonly limits plant growth, many plants cannot thrive if exposed to direct sunlight. There are a number of potential causes: a plant may not be able to dissipate the heat accumulated by direct exposure to sunlight; it may lack the shielding that prevents chlorophyll degradation; or it may be unable to sustain an internal water balance when faced with high transpiration demand. Nevertheless, although many species survive and grow in light shade, few of them do better as shade intensity increases; most plants, including tropical trees, respond positively to increasing light.
Some plants are able to detect shifts in radiation wavelengths caused by neighbouring plants and follow that detection with a rapid growth response (Ballaré et al. 1990; Schmitt et al. 1999). Although the phytochrome mechanism involved has not been widely tested, growth responses to shifts in red-to-far-red ratios among tropical seedlings (Lee et al. 1996) indicate that this is probably a common phenomenon.
In most plant-plant interactions, the costs and benefits are less well defined than those just described. Epiphytes, hemiepiphytes, vines and hemiparasites, for example, all involve one plant providing physical substrate, and sometimes nutrition, for another (usually, but not always, of a different life form). The dependence of one life form on another for physical support is a common phenomenon in the tropics, and the wide range of scaffolding, substrates and trellises available, coupled with life forms capable of exploiting them, enrich tropical ecosystems: diversity breeds diversity. The most common forms of some large families are epiphytic (e.g. Orchidaceae, Bromeliaceae), and the local species richness of epiphytes can rise to 200 species or more, constituting up to half the flora (Nadkarni et al. 2001). The consequences of provision of support by one species for another can range from positive to nil to fatal.
Epiphytes that colonize tree limbs benefit from being perched in the canopy (the obligate habitat of many of them), often without apparent harm to the host. This is a reasonably clear case of facilitation (+/0). In some cases, however, heavy epiphyte loads increase the risk of phorophyte breakage (+/-). The relationship may also be mutually beneficial (+/+), for example if epiphytes slow the passage of atmosphere-borne nutrients that would otherwise pass through the system quickly and be lost before being captured by tree roots (Nadkarni 1981).
Many epiphylls, the leaf-colonizing subset of epiphytes, are capable of fixation of atmospheric nitrogen (e.g. Goosem & Lamb 1986), although the degree to which this nitrogen becomes available to the host is unclear. Epiphylls are generally thought to have a negative impact on their hosts because they capture solar radiation that might otherwise energize the leaf they are growing on or because they hold water that fosters the growth of pathogens (Coley & Kursar 1996). They might also filter incoming direct-beam radiation, reducing the likelihood of chlorophyll-bleaching in the host. Nevertheless, epiphyll growth is far more common in the understorey than in the canopy, which argues against such a sun-screening role being widespread. The abundance of epiphylls on shaded leaves may simply be a consequence of the drought vulnerability of epiphylls, most of which are non-vascular plants.
Hemiepiphytes, which are uniquely tropical, shift their growth habit with age. These plants (principally members of the Moraceae, Clusiaceae, and Araliaceae) begin life as epiphytes but become self-supporting and soil-rooted as adults, a feat that requires substantial morphological, anatomical and physiological plasticity (Holbrook & Putz 1996). By starting life in the crowns of trees, hemiepiphytes circumvent severe competition for light as seedlings. During their epiphytic phase they use the nutrient-rich humus that accumulates in the crown of the host tree (Putz & Holbrook 1989). A small subset of hemiepiphytes is commonly dubbed 'stranglers', although they might more accurately be called 'suicide facilitators', for it is the host tree that continues to enlarge when enveloped by such a hemiepiphyte, eventually leading to its death - a distinct disadvantage of having vascular cambium.
Among the most intricate plant-plant interactions are those between hosts and their hemiparasites or parasites. In the case of the hemiparasitic mistletoes (Loranthaceae, Viscaceae, others), both host and parasite photosynthesize, but the mistletoe has invasive haustoria that penetrate the host's branches, tapping its water supply and, in some cases, its photosynthate (Calder 1983; Lamont 1983; Marshall & Ehleringer 1990). Some parasites show little outward evidence of parasitism, as with the widespread (and valuable) sandalwood (Santalum spp.) and Australia's Nuytsia, both of which look superficially like other trees, but whose roots invade those of non-conspecific hosts (often other trees) on which they depend (Calladine & Pate 2000). In still other cases the parasite has lost its capacity to photosynthesize, thereby becoming entirely dependent upon the host. The most renowned example may be Southeast Asia's Rafflesia, notorious because it produces the world's largest (and one of its most foul-smelling) flower.
Vines are a notoriously successful life form in the tropics and, like obligate epiphytes, dependent upon other life forms for support. Seasonal shutdown of water flow leads to high risk of cavitation in plants having large vessels. It is not uncommon for lianas to have vessels with diameters of 200 µm or more (Ewers et al. 1991), and diameters as high as 700 µm have been recorded (Tyree & Ewers 1996), so it is not surprising that lianas are a much more species-rich life form in the humid tropics than in cold or dry biomes. The impacts of vines on the host plants that provide them with structural support are invariably negative. These impacts include mass load on tree crowns (Putz 1991), interception of solar radiation (Dillenburg et al. 1995) and competition for water (Pérez-Salicrup & Barker 2000; Pérez-Salicrup 2001). In experiments in which trellises of varying diameters were provided or removed at ground level, Putz (1984) concluded that vine growth and survival can be trellis-limited, demonstrating once again the dependence of one life form upon the same or another life form. Twining vines cannot climb large-diameter trellises, so vines themselves become a common path to the canopy for other vines.
Biogeochemical interactions
Although inconspicuous (and hard to measure), chemical interactions among plants can dictate local success, diversification or extinction. They involve common use of elements that are often in short supply relative to plant demand, sharing of water supplies and the production of chemicals by one species that either impact directly or serve as signals to neighbours.
The mineral nutrients contained in plant detritus generally become fair game for any plant in the neighbourhood once they are released by decomposition of the organic matrix. Some plants, however, shortcut the process by capturing detritus behind leaf bases (e.g. some palms: Raich 1983; Putz & Holbrook 1989) or in baskets or tanks formed by leaves (e.g. bromeliads and basket-forming ferns). The ability to intercept detritus can be nutritionally significant; Reich et al. (2003), for example, found that bromeliads switched from atmospheric to host-tree litter sources of nitrogen as their tank diameters, and therefore their detritus-capturing capacity, increased with age.
The provision of nitrogen-rich detritus that can nourish co-occurring species is an example of a passive action by one species that benefits another. Although this phenomenon occurs across a wide range of latitudes, it is especially common in the tropics, where legumes are prominent components of many forests. Nitrogen fixation in the tropics is not restricted to those legumes (primarily in the subfamilies Papilionoideae and Mimosoideae) that have symbiotic associations with bacteria capable of reducing the diatomic nitrogen of the atmosphere. The non-legumes include plants symbiotic with actinomycetes or cyanobacteria that fix nitrogen: e.g. Myrica (Myricaceae) and Casuarina (Casuarinaceae), both symbiotic with Frankia; Parasponia (Ulmaceae; symbiotic with Bradyrhizobium); Psychotria (Rubiaceae; symbiotic with K lebsiella), and Gunnera (Gunneraceae, symbiotic with Nostoc).
Even though nitrogen-fixing tree species commonly resorb about half of their foliar nitrogen prior to abscission, the concentrations achieved in leaves are so high, often 4 per cent or more, that a considerable quantity reaches the forest floor. Once there, it enters the detrital food chain and some of it becomes available to co-occurring plants (e.g. DeBell et al. 1989; Binkley 1992). In time, this inadvertent provision of growth-stimulating nitrogen to competitors presumably can prove detrimental to the nitrogen-fixer. Nevertheless, trees that host nitrogen-fixing bacteria may exhibit their highest rates of fixation when young (e.g. Pearson & Vitousek 2001), so the benefits to competitors may be short-lived.
With relaxation of constraints caused by low nitrogen, other nutrients eventually limit growth. In stands comprising a relative-abundance gradient of a non-nitrogen fixing (Eucalyptus saligna) and a nitrogen-fixing tree species (Albizia falcataria [syn. Falcataria moluccana and Paraserianthes moluccana]), Kaye et al. (2000) found that although available soil nitrogen increased with increasing relative abundance of Albizia, available soil phosphorus declined, presumably because of uptake by trees. Whereas sequestration of nitrogen in above-ground biomass increased with increasing relative abundance of the nitrogen-fixing tree, sequestration of phosphorus in biomass peaked at a Eucalyptus: Albizia mix of 1:2, the same as carbon.
Water, like nitrogen, can be made available to a second species through the actions of a first. Hydraulic lift, whereby water taken up by deep roots flows at night from shallow roots into the surrounding soil, occurs in savannas (Scholz et al. 2002) and probably in other tropical ecosystems. Once in the surface soil, the water potentially becomes available to the plant that expelled it, to a competitor or to evaporative loss.
Unlike nitrogen, which can accumulate in plants and soil through biotic processes, phosphorus is limited from the start by parent material (except for the modest amounts that might enter through atmospheric deposition or flooding). As phosphorus is taken up and sequestered in vegetation, the available supply in the soil declines, until it can eventually limit plant growth. In their classic monograph on shifting cultivation, Nye and Greenland (1960) suggested that extraction of phosphorus by tree roots deep in the soil could lead to replenishment and maintenance of phosphorus in surface soils. Setting out to test this hypothesis, Kellman and Hudson (1982) severed the tap roots of pine trees in a tropical savanna, and after five years of study Kellman (1986) concluded that the tap roots had no effect on the concentrations of foliar phosphorus (or cations). Thus, the best tropical test to date of the intuitively appealing deep-pumping hypothesis has yielded null results.
Allelochemicals are an important means of interaction not only between plants and animals (see Massey et al., Chapter 14, this volume), but also between plants; they constitute an exciting and very active area of chemical ecology (Dicke & Bruin 2001; Callaway 2002). Examples of chemically mediated communications between plants include wounded-plant-to-neighbours (Baldwin et al. 2002), root-to-root (Schenk et al. 1999), pollen-to-stigma (Ottaviano et al. 1992), and plant-smoke-to-seed (Keeley & Fotheringham 1997). Although plant communication research has not had a strong tropical component (and is unlikely to have one soon as the field becomes increasingly focused on molecular biology; e.g. Kessler & Baldwin 2002), it is inconceivable that tropical plants will not possess the full suite of signals and detectors discovered in plants of the temperate zone; they are likely, in fact, to offer some exciting surprises as well.
Support for widespread existence of allelopathy as a mechanism of plant-plant interaction has waned in the past couple of decades. Williamson (1990), however, makes a strong case for the fact that allelopathy is held to a higher standard of proof than are most interactions between plants, and he marshals evidence for both the direct production of allelochemicals by plants and the allelopathic properties of substances resulting from breakdown of non-allelopathic exudates. The species-rich genus Eucalyptus, widely planted throughout the tropics and subtropics, has borne the brunt of popular accusations that it is allelopathic, but the evidence is equivocal (Willis 1991). In any event, the production of allelochemicals and their exudation into the soil to impede colonization by competitors would be an expensive defence mechanism in the humid tropics, where the flow-through of soil water can easily be 75 per cent or more of rainfall; prodigious rates of chemical production would be required to keep up with losses. If allelopathy is a tropical phenomenon, it seems more logical to seek it in the dry tropics than in rainforests.
Outcomes: the three 'C's of plant interactions
The results of competition-density experiments containing two or more species can vary from no interaction between the species to complete dominance by one of them. The intermediate results, i.e. those situations in which two presumed competitors coexist, are of particular interest to community ecologists because they offer clues regarding concomitant use of resources, and therefore mechanisms that promote diversity.
Additive experiments, whereby a fully stocked stand of one species is interplanted with one or more additional plant species, are useful tools for assessing resource availability and invasibility (Snaydon 1991). The continuum of possible results from such experiments can be broken down into three broad categories: (1) competitive dominance of the stand by one species whose growth is not affected by a second species that contributes little to total productivity; (2) compensatory productivity, whereby the growth of both species is slowed by competition, but the sum of their productivity is greater than that achieved by either species in monoculture, and (3) complementarity, in which two species share resources in ways that enable at least one of them to be as productive as it would be in monoculture and resulting in total productivity greater than that achieved by either species in monoculture. In the Costa Rican experiments we have encountered all three outcomes: competition, compensation
Figure 1.2 Productivity of trees and monocots when grown together in an additive design. Each data point represents one replication for one year. Ninety community-years of productivity are plotted: 3 polycultures × 3 replications × 10 years. The dashed lines differentiate low NPP values (i.e. a year of productivity at an average rate ≤ 1 g m-2 d-1) from higher values. The diagonal connects the highest observed annual average monocot and tree NPP values (7.2 and 8.7 g m-2 d-1, respectively).
Image not available in HTML version |
and complementarity. Furthermore, we learned that the response depended on when observations were recorded, as relationships among species changed with age.
© Cambridge University Press