Bacterial-Epithelial Cell Cross-Talk: Molecular Mechanisms in Pathogenesis available in Hardcover
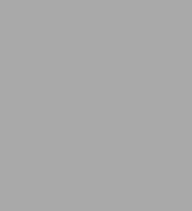
Bacterial-Epithelial Cell Cross-Talk: Molecular Mechanisms in Pathogenesis
- ISBN-10:
- 0521852447
- ISBN-13:
- 9780521852449
- Pub. Date:
- 09/07/2006
- Publisher:
- Cambridge University Press
- ISBN-10:
- 0521852447
- ISBN-13:
- 9780521852449
- Pub. Date:
- 09/07/2006
- Publisher:
- Cambridge University Press
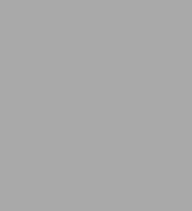
Bacterial-Epithelial Cell Cross-Talk: Molecular Mechanisms in Pathogenesis
Hardcover
Buy New
$156.00Buy Used
$132.29-
-
SHIP THIS ITEM
Temporarily Out of Stock Online
Please check back later for updated availability.
-
Overview
Product Details
ISBN-13: | 9780521852449 |
---|---|
Publisher: | Cambridge University Press |
Publication date: | 09/07/2006 |
Series: | Advances in Molecular and Cellular Microbiology , #13 |
Edition description: | New Edition |
Pages: | 452 |
Product dimensions: | 6.30(w) x 9.29(h) x 1.06(d) |
About the Author
Read an Excerpt
Cambridge University Press
978-0-521-85244-9 - Bacterial-Epithelial Cell Cross-Talk - Molecular Mechanisms in Pathogenesis - Edited by Beth A. McCormick
Excerpt
Part I Introduction to the host and bacterial pathogens
CHAPTER 1
Overview of the epithelial cell
W. Vallen Graham and Jerrold R. Turner
Bacteria must overcome multiple obstacles in order to achieve successful pathogenesis. In many cases, this requires bypassing the first line of host defense: the barrier provided by epithelial surfaces of the integument and the gastrointestinal, respiratory, and urinary tracts. To overcome these barriers, pathogenic organisms frequently initiate mechanisms that exploit essential cellular processes of the epithelium. These cellular processes are therefore critical to our understanding of bacterial pathogenesis. Their description is the goal of this chapter.
GASTROINTESTINAL TRACT
The gastrointestinal epithelium forms a critical interface between the internal milieu and the lumen. The latter should be considered the external environment, since the gut is essentially a tube running through the body that communicates with the external environment at each end. Thus, like the skin, the barrier formed by the gastrointestinal epithelium is critical in preventing noxious luminal contents from accessing the internal tissues. In contrast tothe skin, the gastrointestinal tract must also support passive paracellular and active transcellular transport of nutrients, electrolytes, and water. The barrier formed by the gastrointestinal epithelium must therefore be highly regulated and selectively permeable. Consistent with this, barrier permeability and epithelial transport function vary at individual sites within the gastrointestinal tract according to regional differences in the specific nutrients and ions transported.
Figure 1.1 The human respiratory and gastrointestinal tracts. Selected histological sections are displayed. Counterclockwise from the top right are sections including epithelium from lung alveolus, gastric fundus, colon, ileum, esophagus, and bronchus. See text for detailed descriptions.
Image not available in HTML version |
The oral cavity and esophagus are lined by stratified squamous epithelium (Figure 1.1), much like the skin. Keratinization, a normal feature of the skin, is abnormal in the esophagus and typically occurs only as a response to injury or in association with neoplasia. The esophageal squamous epithelium can be divided into three layers. The basal layer houses the stem cells and their progeny, which, in the normal state, extend up to three cell layers above the basement membrane. Normally this represents 10–15% of overall epithelial height, but it can increase dramatically during active inflammation, as often accompanies esophageal infection. Above the basal layer are the post-mitotic prickle and functional layers. Squamous cells become progressively more glycogenated and flattened as they pass through these layers on their way to the luminal surface. A variety of specialized cell types, including Langerhans cells, which process and present antigens, and endocrine cells, are intermixed with the squamous epithelium. Modified salivary glands are present within the esophageal submucosa. These salivary glands secrete mucus for lubrication, growth factors to augment epithelial cell growth and to aid in repair, and, in the distal esophagus, bicarbonate to neutralize gastric acid. The esophageal squamous mucosa ends at a sharply demarcated site, the Z-line, which marks the transition to the columnar epithelium of the stomach.
The stomach is a distensible saccular portion of the gastrointestinal tract in which food is mixed with gastric acid and digestive enzymes. This mixture, termed chyme, exits the gastric antrum through the pylorus at a controlled rate. The cardia is the small section of the stomach just distal to the Z-line. Like the cardia, the mucosae of the antrum and pylorus, which are the distal portions of the stomach, have long gastric pits and relatively short glands lined by foveolar, or mucus-producing epithelium. This epithelium is preferentially colonized by Helicobacter pylori. Thus, the antrum is most frequently affected and the optimal site of diagnostic biopsy for H. pylori infection. The fundus, which is a dome-shaped region projecting upward and to the left of the gastroesophageal junction, and the body of the stomach are lined by acid-producing, or oxyntic, mucosa with short pits and an expanded glandular compartment (Figure 1.1). The superficial areas of oxyntic mucosa are lined by foveolar cells that secrete neutral mucins, but the deep pits and glands contain parietal, chief, and endocrine cells. Parietal cells secrete acid as well as intrinsic factor, which is necessary for the absorption of vitamin B12 in the terminal ileum, while chief cells secrete pepsinogen. These areas are typically colonized by H. pylori only after loss of parietal cells and resultant increases in gastric pH. Chronic gastritis involving any portion of the stomach can also lead to metaplasia, with the appearance of small intestinal-like mucin-secreting goblet cells. Unlike gastric foveolar mucus cells, these cells express acid mucins, have a well-developed brush border, and are not readily colonized by H. pylori.
Despite its name, the small intestine is the longest portion of the gastrointestinal tract, extending for a length of approximately 6 m in adults. The small intestine is divided into three functionally distinct sections: duodenum, jejunum, and ileum. The primary function of each of these sections is to absorb luminal contents, although the specific molecules absorbed differ by region. For example, although 90% of absorption occurs within the first 100 cm of the small intestine, the jejunum is the principal site of absorption for Na+ cotransported monosaccharides and amino acids. The ileum is essential to the enterohepatic recirculation of bile salts and the absorption of certain vitamins, including B12. Moreover, the terminal ileum contains abundant lymphoid tissue, termed Peyer’s patches. These lymphoid follicles are a favored site of Yersina infection. Since the Peyer’s patches actively sample luminal antigens, including those provided by bacteria, the terminal ileum is an effective location for this function; luminal bacterial load averages 1 000 000 bacteria per 1 ml in the distal ileum as opposed to 100 bacterial organisms per 1 ml in the duodenum and jejunum.
Small-intestinal nutrient absorption is facilitated by tissue architecture (Figure 1.1). The surface area of the small intestine is increased greatly by grossly visible mucosal folds, smaller finger-like protrusions, or villi, and a dense microvillus brush border. These combine to provide an overall luminal surface area that is approximately 600-fold greater than the corresponding serosal surface area. Thus, the loss of villi and mucosal flattening that accompany some infections and generalized bacterial overgrowth syndromes can result in significant malabsorption due simply to loss of absorptive surface area.
In addition to regional specialization along the length of the small intestine, there is significant specialization from the regenerative basally located crypt to the tip of the villus. The epithelial stem cells reside in the crypt, where they proliferate and give rise to specialized enteroendocrine cells and Paneth cells, which remain in the crypt. The stem cells also differentiate into goblet cells, M-cells, and undifferentiated crypt cells. The goblet cell is named for its large mucin vacuole, which compresses the cytoplasm into a shape reminiscent of a wine goblet. Goblet cells are present throughout the crypt–villus axis (Figure 1.1). M-cells, or follicle-associated epithelial cells, are most prominent in the distal ileum overlying Peyer’s patches, but they can be found throughout the intestines. M-cells are specialized for the bulk endocytic sampling of luminal contents and are found in the flattened dome epithelium overlying lymphoid follicles. The most prominent feature of the M-cell is its shape, described as having microfolds, from which the ‘M’ is derived. The basal membrane of the M-cell is retracted from the basement membrane to form a cleft into which lymphocytes and macrophages migrate. Apically derived endocytic vesicles are rapidly released into this cleft by exocytosis, with some luminal particles arriving in the cleft space within 10 min of apical endocytosis. This highly efficient transport pathway is exploited as a route into and across the epithelium by some infectious organisms.
The undifferentiated crypt cells remain mitotically active while they are in the crypt and, thus, are somewhat intermediate between stem cells and terminally differentiated cells. Despite their name, undifferentiated crypt cells possess significant functional differentiation and effectively transport Cl− into the intestinal lumen. This creates an osmotic gradient that draws water into the lumen. The latter is essential for normal homeostasis and is present in an exaggerated form in many diarrheal diseases, including cholera. As these undifferentiated crypt cells migrate towards the villus, they lose their ability to divide and modify their gene-expression profile to become specialized for absorption. This includes expression of absorptive nutrient and ion transporters, brush-border digestive enzymes, and kinases unique to villus epithelium (Clayburgh, Rosen et al., 2004; Llor et al., 1999). Ultimately, these absorptive cells become senescent and are shed from the villus tip. This occurs via an orderly process that allows the epithelium to maintain barrier function despite cell shedding (Madara 1990; Rosenblatt et al., 2001).
The colon, which begins at the ileocecal valve and continues to the rectum, is approximately 1 m long in adults. The main functions of the colon are absorption of water and electrolytes and storage of waste products. Transport is accomplished by crypt and surface epithelial cells, which are functionally similar to those in the small-intestinal crypt and villus, although villi are not present (Figure 1.1). Bacterial load in the colonic lumen can be quite high, with typical yields of 1011 organisms per 1 ml. These commensal organisms typically coexist peacefully with the host and may serve beneficial roles (Abreu et al., 2005; Fukata et al., 2005; Neish et al., 2000; Rakoff-Nahoum et al., 2004). The presence of these commensal organisms may also prevent overgrowth of pathogenic organisms. For example, incomplete sterilization of the lumen by antibiotic treatment may allow overgrowth of Clostridium difficile, which release toxins that glucosylate and inactivate rho (Just et al., 1995). This results in a severe and sometimes fatal colitis characterized by dense adherent pseudomembranes composed of purulent material.
RESPIRATORY TRACT
The proximal larynx is lined by stratified squamous epithelium. Although this is similar to that present in the esophagus, glycogenation is less prominent in the laryngeal epithelium. This gives way to pseudostratified columnar epithelium, although patches of squamous epithelium continue to be present distally. The columnar epithelium can vary in thickness from only a few cells to many cells. Small round reserve cells characterize the basal layer, while the most superficial layers are covered by ciliated epithelial cells and variable numbers of mucin-producing goblet cells. Together, these surface cells provide an important line of defense, as the cilia beat continually and propel a thin layer of mucin with entrapped foreign material upward and out of the airway. This mucociliary clearance is critical, as demonstrated by patients with Kartagener syndrome, in whom ciliary immotility results in recurrent infection (Eliasson et al., 1977).
The larynx leads to the trachea and bronchi. These large-bore airways are encircled by cartilaginous rings, which prevent their collapse. The trachea and bronchi are lined by ciliated columnar epithelium similar to that seen in the distal larynx. Corynebacterium diphtheriae can colonize these sites and release a toxin that adenosine diphosphate (ADP)-ribosylates elongation factor 2, resulting in epithelial cell death. The mucosal necrosis and sloughing that follow result in accumulation of mucus, cellular debris, and inflammatory cells that obstruct the airways and kill by asphyxiation.
As the bronchi branch into smaller bronchioles, the pseudostratified epithelium becomes simpler, assuming a single-layer cuboidal morphology lined by ciliated and non-ciliated epithelial cells. The latter, termed Clara cells, secrete surfactant and serve as progenitor cells in repair after injury. The bronchioles terminate in the functional unit of the lung, the alveolus, where gas exchange takes place. Alveoli are lined by a thin layer of type 1 squamous and type 2 cuboidal pneumocytes (Figure 1.1). The terminally differentiated type 1 cells cover over 90% of the alveolar surface and provide a thin separation between the airspace and blood that allows for efficient gas exchange. Type 2 cells synthesize and secrete surfactant and also serve as alveolar stem cells, giving rise to additional type 2 cells as well as type 1 cells. In the presence of infection, for example with Streptococcus pneumoniae, the most common cause of community-acquired acute pneumonia, the alveoli may become filled with fluid and inflammatory cells, including neutrophils and macrophages. Infection spreads rapidly within contiguous alveoli, leading to consolidation of large areas of lung parenchyma and loss of the surface area necessary for gas exchange.
URINARY TRACT
The renal collecting ducts empty into the renal pelvis. The latter is lined by urothelium, which is histologically similar in the renal pelvis, ureters, and bladder. This epithelium is also termed transitional, as its appearance is intermediate between that of non-keratinizing stratified squamous epithelium, as seen in the esophagus, and pseudostratified columnar epithelium, like that of the bronchi. The thickness of the urothelium varies significantly, from only two to three cells thick in the renal pelvis to seven or more cells in the contracted bladder. As the bladder distends, the urothelium thins to two to three cells that are elongated parallel to the basement membrane. Like other stratified epithelia, proliferation occurs in the basal zone, which is populated by small cuboidal cells. The surface is comprised of terminally differentiated cells. In the bladder, these surface cells are termed umbrella cells due to their large ellipsoid shape. Like deeper cells, the umbrella cells become flattened and difficult to appreciate in the distended bladder. They are often absent in urothelial carcinoma, making their presence a somewhat reassuring, although non-diagnostic, feature in bladder biopsies. Bacterial infections of the bladder and urethra include Escherichia, Proteus, Klebsiella, and Enterobacter and are more common in women than men due to the shorter length of the female urethra. Such infections can extend in a retrograde fashion to involve ureters, renal pelvis, and renal parenchyma in ascending pyelonephritis.
VECTORIAL TRANSPORT
Despite the differences in specialized cellular functions and morphology, all of these tissues are lined by a polarized epithelium that provides protection from the environment. Therefore, for the purpose of understanding cellular architecture, the structure of these polarized epithelia can be generalized. At the cellular level, polarization allows for asymmetric morphology as well as distinct molecular composition and function of membrane domains. The free surface, which faces the lumen, is referred to as apical, while the surfaces in contact with the basement membrane and adjacent cells are referred to as basal and lateral, respectively. The basal and lateral membrane domains typically function as a single unit, termed the basolateral domain. Specific proteins and lipids can be targeted to apical and basolateral membrane domains. For example, Na+ nutrient cotransporters are recruited to the apical membrane in transporting epithelia (Harris et al., 1992). Similarly, the GM1 ganglioside used as a receptor for cholera toxin is also primarily apical (Lencer et al., 1995; Wolf et al., 1998). Other proteins, such as β1 integrins and interferon gamma (IFNγ) and tumor necrosis factor alpha (TNFα) receptors, predominantly localize to the basolateral surface in polarized epithelia (Boll et al., 1991; Madara and Stafford, 1989; Moller et al., 1994). Finally, some proteins, such as the polymeric immunoglobulin receptor, have more complex trafficking patterns. The polymeric immunoglobulin receptor is trafficked to the basolateral membrane first, where it picks up immunoglobulin cargo; then the receptor is endocytosed and moved to the apical membrane, where it releases the cargo (Casanova et al., 1990, 1991; Mostov et al., 1986).
In each case, the appropriately polarized distribution of proteins and lipids is necessary for proper epithelial function. For example, as noted above, Na+ nutrient cotransporter proteins are apically targeted in intestinal and renal tubular epithelium (Harris et al., 1992; Turner et al., 1996; Wright et al., 1997). In the case of glucose absorption, glucose is transported into the cell against its concentration gradient by SGLT1, the apical intestinal Na+/glucose cotransporter by Na+ cotransport using the Na+ gradient, from high outside the cell to low inside the cell, as the driving force (Hediger et al., 1987). This Na+ gradient is maintained by the Na+/K+ ATPase. The Na+/K+ ATPase is localized to the basolateral membrane along with the facilitated glucose transporter GLUT2 (Dominguez et al., 1992; Miyamoto et al., 1992). Thus, after SGLT1 transports Na+ and glucose across the apical membrane, glucose and Na+ exit the cytoplasm by crossing the basolateral membrane. This allows for directional net absorption and generates an ionic gradient that draws in water from the lumen. Similar mechanisms move water and solutes across the alveoli (Nielson and Lewis, 1990). Clearly, none of this would be possible in the absence of polarized membrane domains.
Membrane polarization is also essential for secretion. The cystic fibrosis transmembrane conductance regulator (CFTR) is a transporter responsible for apical cyclic adenosine monophosphate (cAMP)-activated anion conductance (Anderson et al., 1991; Kartner et al., 1991; Tabcharani et al., 1991). Cystic fibrosis is linked to mutations in CFTR, and these mutations are responsible for the poorly hydrated viscous luminal accumulations that cause intestinal, pancreatic, and airway obstruction (Gregory et al., 1990; Riordan et al., 1989). In normal subjects without CFTR mutations, cholera toxin activates CFTR by elevating intracellular cAMP levels (Breuer et al., 1992). This results in net transport of Cl− ions into the intestinal lumen, followed by massive fluid accumulation (Field, 2003). Thus, cholera takes advantage of normal cellular processes to cause massive diarrhea, which spreads infection in areas with poor sanitation.
MEMBRANE TRAFFIC
In addition to transporters that allow solutes to cross apical and basolateral membrane domains, epithelia also utilize vesicular routes in order to traffic materials and to respond to extracellular stimuli. The trafficking of immunoglobulin A (IgA) from basolateral to apical surfaces by polymeric immunoglobulin receptor is a particularly well characterized example of such transport. As noted above, the polymeric immunoglobulin receptor is initially trafficked to the basolateral membrane. Here, it binds polymeric immunoglobulin, most prominently IgA (Casanova et al., 1990, 1991; Mostov et al., 1986). The binding of IgA triggers internalization of the complex and trafficking of the endocytic cargo to the apical surface. A proteolytic cleavage event at this surface results in release of IgA at the apical, or luminal, surface along with a portion of the receptor, termed the secretory component (Aroeti et al., 1993; Casanova et al., 1991; Song et al., 1994). Although this finely tuned process plays an important role in mucosal immune protection, it has apparently not been able to escape the attention of Streptococcus pneumoniae. These bacteria exploit the presence of uncleaved polymeric immunoglobulin receptor at the apical membrane to bind to the secretory component portion of the receptor and invade host cells (Zhang et al., 2000). The binding of S. pneumoniae to polymeric immunoglobulin receptor drives transcytosis in reverse, from apical to basolateral, thereby effectively transporting bacteria across the epithelium (Brock et al., 2002). This pathway is clinically relevant, as the pattern of polymeric immunoglobulin receptor expression, greater in nasopharyngeal than distal respiratory epithelium, likely also explains the more frequent colonization of upper airways by S. pneumoniae (Zhang et al., 2000).
© Cambridge University Press