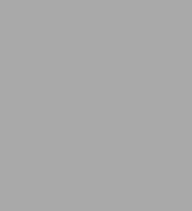
Wildlife and Wind Farms - Conflicts and Solutions: Onshore: Potential Effects
220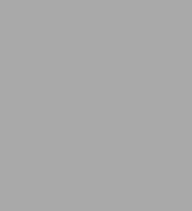
Wildlife and Wind Farms - Conflicts and Solutions: Onshore: Potential Effects
220Paperback(Reprint)
-
PICK UP IN STORECheck Availability at Nearby Stores
Available within 2 business hours
Related collections and offers
Overview
This unique multi-volume work provides a comprehensive overview of the interactions between wind farms and wildlife.
Volume 1 documents the current knowledge of the potential impacts upon wildlife during both construction and operation. An introductory chapter on the nature of wind farms and the impact assessment process is followed by a series of in-depth chapters documenting effects on climatic conditions, vegetation, terrestrial invertebrates, aquatic invertebrates and fish, reptiles and amphibians, birds, bats and terrestrial mammals. A synopsis of the known and potential effects of wind farms upon wildlife in perspective concludes the volume.
The authors have been carefully selected from across the globe from the large number of academics, consultants and practitioners now engaged in wind farm studies, for their influential contribution to the science. Edited by Martin Perrow and with contributions by 40 leading researchers including: Robert Barclay, Michael Dillon, Jan Olof Helldin, Hermann Hötker, Jeffrey Lovich, Manuela de Lucas and Eugene Takle. The authors represent a wide range of organisations and institutions including the Universities of Calgary, Iowa State, Lund & Wyoming, US Geological Survey, Michael-Otto-Institut im NABU, Norwegian Institute for Nature Research, Spanish Council for Scientific Research, Renewable Energy Systems and several leading consultancies.
Each chapter includes informative figures, tables, colour photographs and detailed case studies. Many of the latter are produced stand-alone from invited additional authors to ensure geographic spread and to showcase exciting new, often previously unpublished research.
This book is designed for practitioners, researchers, managers and for a range of students in higher education, particularly those involved with environmental, ecological, conservation, impact assessment and climate change studies.
Other volumes: Volume 2: Onshore: Monitoring and Mitigation (978-1-78427-123-7) Volume 3: Offshore: Potential Effects (978-1-78427-127-5) Volume 4: Offshore: Monitoring and Mitigation (978-1-78427-131-2)
Product Details
ISBN-13: | 9781784271190 |
---|---|
Publisher: | Pelagic Publishing |
Publication date: | 06/25/2017 |
Series: | Conservation Handbooks , #1 |
Edition description: | Reprint |
Pages: | 220 |
Product dimensions: | 6.60(w) x 9.50(h) x 0.90(d) |
Read an Excerpt
CHAPTER 1
The nature of wind farms
GERO VELLA
Summary
This chapter discusses the technological aspects of wind power; the policy, planning and statutory requirements underpinning its deployment; and the development process from site selection through to permit award, mitigation and monitoring. While this chapter presents a global perspective, many of the examples provided have a European focus drawn from the author's own experiences in developing wind farms in the UK. Research for the chapter was undertaken through a literature review of academic papers, government and industry reports and grey literature. The review found that policy support worldwide has led to the construction of wind farms in more than 90 countries and a contribution of around 3% to the renewable energy mix globally, but with much higher contributions in areas where there is a good wind resource. Large three-bladed horizontal-axis wind turbines continue to dominate commercial wind farms, but deployment of small to medium-sized turbines for domestic and rural applications is increasing, in addition to the use of vertical-axis wind turbines in urban locations. The focus of Environmental Impact Assessment as a key stage in the development process is discussed and the importance of robust site selection is highlighted as mitigation against potential environmental impacts.
Introduction
Since early history, humans have harnessed the power of the wind to propel boats, grind grain and pump water. When the generation of electricity became sufficient for industrial purposes at the end of the nineteenth century, the first prototypes of modern wind turbines were built using technology based on the classical windmill. Generally, these were to provide power to farms and remote locations. It was not until the oil crisis of the 1970s, with the consequential increase in the price of oil, that interest was raised in identifying ecologically and commercially viable alternative energies and wind power came into focus. For example, in the USA, the Federal Government worked with the National Aeronautics and Space Administration (NASA) to research and develop utility-scale wind turbines (Wind Energy Foundation 2015). Thereafter, concerns were raised over the environmental and economic threat of global warming due to a number of 'greenhouse' gases (GHGs). Thus, increasing atmospheric concentrations of GHGs, such as carbon dioxide and methane resulting from industrial processes, the refining and burning of fossil fuels, and intensive livestock farming, provided additional stimulus for research and development into the potential of wind power (Box 1.1).
The past decade has seen unprecedented deployment of wind power worldwide driven by renewable energy targets established in more than 160 countries [Renewable Energy Policy Network for the 21st Century (REN21) 2015]. The World Wind Energy Association (WWEA 2015) reports a global installed capacity of nearly 370,000 megawatts (MW) of wind power, the majority of which is distributed across Asia, North America and Europe (Figure 1.1). At the time of writing, China is the world leader, generating over 30% of global wind power, followed by the USA, Germany, Spain and India, with emerging markets in Brazil and Africa. Furthermore, the majority of the generation is from onshore wind, with only 2.5% being generated offshore by the end of 2014 [Global Wind Energy Council GWEC) 2015a], although the huge potential for offshore wind should be noted (see Wildlife and Wind Farms: Conflicts and Solutions, Volume 2: Offshore).
In addition to renewables targets and incentives such as green tariffs, the GWEC and Greenpeace International (2014), together with Zindler (2015), report that in an increasing number of world markets, wind power is the lowest cost option when adding new generation capacity to the electricity grid. As a result, wind power has now firmly established itself as a mainstream option for new electrical generation. Yet despite the rapid deployment of onshore wind, there are still a number of permitting barriers. These relate to potential impacts on birds, bats and other wildlife, and social concerns such as the visual and cultural setting of wind farms and their performance and value. This has led to an application refusal rate of approximately 50% per year in the UK between 2013 and 2014 (RenewableUK 2014b; 2015b). While many of the refusals relate to concerns over impacts to local communities, wind farms do present a number of potential impacts to different wildlife taxa which may contribute to delays in permitting or refusal. In brief, key impacts during construction and decommissioning include direct and indirect impacts associated with displacement of wildlife or their prey from construction noise and activity, habitat modification from installation of the wind turbines, access roads and other infrastructure, and habitat loss resulting from, for example, the removal of hedgerows and trees. During operation, key concerns relate to the collision risk to birds and bats, or their displacement if they avoid the wind farm and its surrounding area owing to turbine operation and maintenance or visitor disturbance. Displacement can include barrier effects in which birds are deterred from using their normal routes to feeding or roosting grounds. For reviews, see Hötker et al. (2006), Arnett et al. (2007), May and Bevanger (2011), Rydell et al. (2012), Arnett and Baerwald (2013) and Gove et al. (2013).
It is predicted that wind power could reach nearly 2 million MW by 2030, equating to approximately 19% of global electricity requirements and a saving of over 3 billion tonnes of carbon dioxide emissions annually (GWEC 2014). To achieve this, there needs to be continued research and development into wind power technology, supported by global and national policies. In addition, both governments and wind farm developers need to work together to reduce continued concerns over the potential environmental and social barriers.
Scope
Three themes are discussed in this chapter:
Technological aspects of wind power
Policy, planning and statutory requirements
The development process.
The first theme will review the technology: the types of wind turbines in use and how they work. The theme focuses on three-bladed wind turbines, which are most commonly deployed in wind farms, but also considers small to medium-sized wind turbines and vertical-axis wind turbines. The second theme will consider the planning and statutory permitting requirements, focusing on those relative to considering wildlife and the environment, using the UK as a specific example. The third theme will describe the development process that wind farm companies typically undertake in identifying wind farm sites, from initial site selection through to mitigation and monitoring.
Although this chapter presents a global perspective, many of the examples provided have a European focus drawn from the author's own experiences in developing wind farms in the UK.
Themes
The technology of wind power
Generation of electricity
Wind turbines use the kinetic energy of the wind to generate electrical energy. Traditional turbines consist of three main elements: the tower, the nacelle and, most commonly, three rotor blades. The steel tower is fixed to the ground by a concrete foundation. The nacelle, composed of glass-fibre reinforced plastic, sits at the top of the tower and is connected to the blades via the rotor hub. Both hub and blades are also typically composed of glass-fibre reinforced plastic. A rotor shaft inside the nacelle connects the hub to the generator, usually via a gearbox.
The wind blows on the angled blades of the rotor, causing the rotor shaft to rotate and convert some of the wind's kinetic energy into mechanical energy. The generator converts this mechanical energy into electrical energy. Traditional wind turbines employ a gearbox to increase the rotational speed of the rotor shaft and, therefore, the efficiency of the generator (Figure 1.2). An anemometer on top of the nacelle monitors wind speed and direction, starting the turbine when wind speeds are sufficient, the 'cut-in speed', and shutting it down when wind speeds exceed its maximum operating speed. In general, turbines cut in at around 4 or 5 metres per second (m/s) and shut down at speeds exceeding 30 m/s (Boyle 2004). Additional sensors can also direct (yaw) the nacelle and rotor into the wind, and pitch (feather) the blades to control energy capture.
A transformer at the base of the turbine converts the electricity generated by the wind turbine at around 700 volts (V) to a more typical voltage for distribution to electrical distribution networks such as 33,000 V, which is equal to 33 kilovolts (kV). Where a turbine connects to a high-voltage distribution network, usually when the turbine is part of a wind farm, buried cables transfer the medium voltage electricity to a substation where it is converted to high voltage (132/275/400 kV). The high-voltage electrical distribution network transmits the electricity around the country. The process of electricity generation and distribution is illustrated in Figure 1.3.
Turbine design
There are two main classes of wind turbine: those whose rotors spin about a horizontal axis, termed horizontal-axis wind turbines (HAWTs), and vertical-axis wind turbines (VAWTs), whose rotors spin about a vertical axis (Figure 1.4). HAWTs typically have either two or three blades. Three-bladed HAWTs are operated 'upwind', with the blades facing into the wind. Sensors mounted above the turbine nacelle orientate the rotor with respect to the wind. The rotor speed as well as the power output can be controlled by pitching the rotor blades along their longitudinal axis. This blade pitching allows the aerodynamically engineered blades to control lift and thus power generation from the wind, but also acts as a form of protection against extreme wind conditions and overspeed through pitching to reduce lift.
Most early HAWT designs operated at fixed speeds and utilised brakes to hold the blades until a sufficient wind speed was detected. Modern HAWTs (Figure 1.5) operate at variable speeds with sensors allowing the rotor blades to match the wind speed until peak or 'rated' speeds are attained. This allows cost-effective energy generation at low speeds in light winds.
Although one- and two-bladed wind turbines are available, the European Wind Energy Association (EWEA) notes that three-bladed designs are most commonly deployed in commercial wind farms as they are structurally efficient (or balanced), in addition to providing other benefits including lower acoustic noise emissions and visual impacts associated with the less frequent passage of only a single blade or two blades in comparison to three blades (EWEA 2004). To further improve the energy capture of commercial three-bladed HAWTs, blade aerodynamics has been a key focus of research and development.
VAWTs can be divided into two major groups: Savonius type, which use aerodynamic drag to extract power from the wind; and Darrieus type, which use lift (Figure 1.6). The advantages of VAWTs are that they can utilise winds from all directions, unlike traditional HAWTs which need to track the oncoming wind. This is an important advantage in locations where winds are turbulent, gusty and constantly changing direction, such as in towns and cities. The omnidirectional nature of electricity generation in VAWTs also provides mitigation against 'down-time' lost to the requirement to yaw or swivel to face the wind (Boyle 2004). In addition, the location of the gearbox and generator at the base of the turbine allows easy access and therefore generally reduces maintenance costs.
Turbine size
Modern wind turbines have shown a significant increase in size and rotor diameter since the 1970s and 1980s, leading to greater power generation. EWEA (2004) reports that in spite of repeated predictions that turbine size would level off at an optimum mid-range, the size of turbines deployed in commercial wind farms has increased year on year. From rotor blade diameters of 15–20 m in the 1980s, the commercial market is now ready to deploy turbines with rotor blade diameters greater than 125 m atop hub heights exceeding 100 m, such as the Enercon E-126 rated at 7,580 kW (Enercon 2015). Permitting applications for onshore wind farms in the UK regularly assume a turbine tip height of around 150 m for the purpose of impact assessment. Offshore, wind turbine sizes are even larger, as shown by planning permission for Creyke Beck offshore wind farm (Forewind 2013), which incorporates 10,000 kW (or larger) wind turbines with a maximum blade tip height of 313 m above sea level. For further information on the nature of offshore wind turbines, see Jameson et al, Chapter 1, Volume 3 of this series.
In parallel with the trend of increasing rotor blade length and the size of turbines, there has also been significant increase in the development and deployment of small and medium-sized wind turbines. These classes of turbine range in size from micro-turbines less than 20 m in height to medium-sized wind turbines of up to 55 m (Table 1.1). RenewableUK (2014a) commonly categorises small wind turbines as single-turbine installations, usually owned by individual homeowners or farmers for on-site electricity consumption, such as the example shown in Figure 1.7. In comparison, medium-sized wind projects are larger developments that may also supply electricity to the grid, and are often investor financed. Importantly, RenewableUK notes that despite the size differences, small and medium-sized projects are typically installed to provide on-site electricity to homes, farms and small communities. They only use the electricity grid to sell back any excess generation above their immediate energy needs. This is in contrast to large-scale wind farms that feed all their generated electricity direct to national electricity grids.
The WWEA reports that globally, 806,000 small wind turbines were in operation by 2012 (WWEA 2014), with over 25,000 small and medium-sized wind systems reported in the UK alone (RenewableUK 2015a). With inevitable advances in the manufacturing processes, the cost of these turbines is likely to decrease and one would imagine that their deployment would increase accordingly. Crucially, the small to medium-sized wind market provides the diversity of scale to allow a wider range of society to implement renewable energy options to suit their needs, thus reducing our reliance on energy from fossil fuels.
Wind farms
The term 'wind farm' generally refers to more than two wind turbines connected to the national electricity grid. Modern wind farms tend to comprise turbines that generate more than 1 MW, and typically 2 MW of power. As wind farms are only as productive as the wind resource that powers them, they tend to be sited at elevated and exposed locations where there are good average wind speeds and a minimum of obstacles and obstructions such as trees, hills and other buildings to affect the wind resource. Other important aspects of their siting include sufficient separation from noise-sensitive neighbours, good access to the electricity grid, good site access to transport the turbine components to the site, and an absence of special environmental or landscape designations (see The development process, below).
With policy support for renewable energy and wind power globally, the size of wind farms keeps increasing. At the time of writing, Alta Wind Energy Center (AWEC) in California, USA, is the largest single wind farm in the world. Once complete, it will have a capacity of 1,548 MW generated from up to 600 turbines (California Energy Commission 2015). Several of the world's largest wind farms are in the USA, with others scattered across the globe in Asia, Australasia and Europe (Table 1.2). In contrast, the UK's largest site at Whitelee near Glasgow, at 215 turbines producing 539 MW, is around a third of the size and capacity of the current world leader. It is, however, the second largest wind farm in Europe.
Planning and statutory requirements
In the UK, a number of government policies and parliamentary acts govern the planning process for onshore wind. Key policies include the UK Government's National Planning Policy Framework, the National Policy Statement for Renewable Energy Infrastructure, the Planning Act and the Localism Act, all of which apply to England and Wales; the Scottish Planning Policy for Renewable Energy, which applies to Scotland; and the Planning Policy Statement for Northern Ireland. Further requirements are set out in the individual Town and Country Planning Acts for England, Wales and Scotland.
(Continues…)
Excerpted from "Wildlife and Wind Farms, Conflicts and Solutions Volume 1"
by .
Copyright © 2017 Pelagic Publishing.
Excerpted by permission of Pelagic Publishing.
All rights reserved. No part of this excerpt may be reproduced or reprinted without permission in writing from the publisher.
Excerpts are provided by Dial-A-Book Inc. solely for the personal use of visitors to this web site.
Table of Contents
Contributors vi
Preface ix
1 The nature of wind farms Gero Vella 1
2 Climate Eugene S. Takle 24
3 Vegetation Margarida R. Silva Isabel Passos 40
4 Terrestrial invertebrates Sarah Elzay Lusha Tronstad Michael E. Dillon 63
5 Aquatic organisms William O'Connor 78
6 Reptiles and amphibians Jeffrey E. Lovich Joshua R. Ennen 97
7 Birds: displacement Hermann Hötker 119
8 Birds: collision Manuela de Lucas Martin R. Perrow 155
9 Bats Robert M.R. Barclay Erin F. Baerwald Jens Rydell 191
10 Terrestrial mammals Jan Olof Helldin Anna Skarin Wiebke Neumann Mattias Olsson Jens Jung Jonas Kindberg Niklas Lindberg Fredrik Widemo 222
11 A synthesis of effects and impacts Martin R. Perrow 241
Index 277