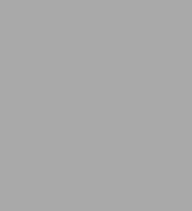
Tunnel Visions: The Rise and Fall of the Superconducting Super Collider
480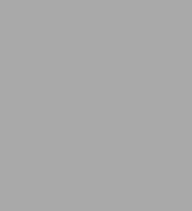
Tunnel Visions: The Rise and Fall of the Superconducting Super Collider
480Paperback(Reprint)
-
PICK UP IN STORECheck Availability at Nearby Stores
Available within 2 business hours
Related collections and offers
Overview
Product Details
ISBN-13: | 9780226598901 |
---|---|
Publisher: | University of Chicago Press |
Publication date: | 09/28/2018 |
Edition description: | Reprint |
Pages: | 480 |
Product dimensions: | 6.00(w) x 9.00(h) x 1.10(d) |
About the Author
Lillian Hoddeson, the Thomas Siebel Professor Emerita of the History of Science at the University of Illinois, is coauthor of Crystal Fire, Critical Assembly, True Genius, and Fermilab: Physics, the Frontier, and Megascience.
Adrienne W. Kolb was, until her retirement in mid-2015, the Fermilab archivist. She is coauthor of Fermilab: Physics, the Frontier, and Megascience.
Read an Excerpt
CHAPTER 1
Origins of the Super Collider
In all failures, the beginning is certainly half the whole. — GEORGE ELIOT, Middlemarch
During the late 1970s, US high-energy physicists could look back on three decades of unparalleled achievement. They had constructed a steady succession of particle accelerators with exponentially increasing energies and used them to probe the interior of the atomic nucleus, making one major discovery after another of the fundamental particles from which matter is made and about the forces binding them together. Combined with theoretical advances, in which US physicists also played a leading role, these discoveries culminated in the Standard Model of particle physics, the dominant paradigm to which almost all members of the discipline subscribed by 1980. This theory posits that ordinary matter is composed of basic building blocks called quarks and leptons — point-like particles, collectively called fermions, that carry half-integer spin. These constituents interact by exchanging other kinds of particles (such as the familiar photon) bearing integer spin called gauge bosons. The Standard Model succeeded in combining the previously dissimilar electromagnetic and weak forces into a single, unified force called the electroweak force. Unifications of fundamental forces are exceedingly rare and thus tremendously significant events in the history of physics, typically occurring about once every century.
Largely because of their ability to construct ever more powerful accelerators and colliders, in which two particle beams clash to generate the highest collision energies attainable, US physicists had taken the lead in this research. In the 1970s, proton or electron machines at Brookhaven National Laboratory (BNL) and Cornell University in New York, the Fermi National Accelerator Laboratory (FNAL, also called Fermilab) in Illinois, and the Stanford Linear Accelerator Center (SLAC) in California supplied US physicists with a variety of high-energy beams. Europeans strove to keep pace, collaborating to construct competitive proton machines at the European Center for Nuclear Physics (CERN) near Geneva, Switzerland, and electron machines at the Deutsches Electronen Synchrotron (DESY) in Hamburg, West Germany. But the entrepreneurial spirit and risk taking that characterized American experimenters had generally won this competition, allowing them to make the lion's share of the important discoveries while their European counterparts were able only to confirm these breakthroughs. During the 1960s and 1970s, five quarks and two additional leptons turned up initially at laboratories west of the Atlantic.
European physicists had evolved a more conservative tradition of building particle accelerators, colliders, and detectors, which were thoroughly engineered before construction began. While it meant that the equipment generally worked as designed from the outset, this approach also took longer to implement, giving more adventurous US physicists the inside track on important discoveries. On the other hand, it also meant that Europeans had the edge in constructing complicated particle detectors, such as the immense Gargamelle bubble chamber at CERN, which they used to obtain convincing evidence in 1973 for the weak neutral currents required by theories of electroweak unification.
US HIGH-ENERGY PHYSICS AT A CROSSROADS
As the 1970s ended, however, Europe was pulling abreast of America in high-energy physics, often called particle physics. Due in part to US funding delays, DESY's collider PETRA began operations in 1979, more than a year before its SLAC equivalent PEP. PETRA eventually allowed physicists to study the collisions of electrons with their antimatter counterparts, called positrons, at combined energies over 40 billion electron volts, or 40 GeV — about five times higher than previously attainable. This advantage meant that European physicists (plus several groups of US physicists working at DESY) received credit that year for discovering the gluon — the gauge boson responsible for conveying the strong force that sequesters quarks together inside protons and neutrons.
Moreover, the European particle-physics community, which had for years been steadily concentrating its efforts at CERN, was developing adventurous plans for the future as the 1980s began. CERN was adapting a large proton accelerator, the 300 GeV Super Proton Synchrotron, or SPS, to function also as a high-energy proton-antiproton collider able to produce the ultra-massive W and Z particles predicted by the Standard Model and thought to be responsible for radioactivity. And CERN was planning a far bigger machine, the Large Electron Positron (LEP) collider, which would occupy a 27 kilometer tunnel under the Swiss and French countryside and eventually allow electron-positron collisions to occur at energies up to 200 GeV.
In contrast, US high-energy physics had been buffeted by shifting economic and political forces during the 1970s. In part because of the Arab oil embargo of 1973 and the attendant surge in energy costs, a major shakeup occurred in its principal federal funding agency. An outgrowth of the Manhattan Project, the Atomic Energy Commission, or AEC (which funded almost all US accelerator building through the mid-1970s), was dissolved in 1974 and its responsibilities segregated into the Energy Research and Development Administration (ERDA) and the Nuclear Regulatory Commission (NRC). ERDA in turn became part of the even larger Department of Energy (DOE) under the Carter administration in 1977. High-energy physics was subsequently just one part of a larger energy portfolio, which included billions of dollars for solar and renewable-energy projects during the late 1970s. Funding for US high-energy physics was nearly flat (in constant dollars) during the latter half of the decade, while the costs of constructing its ever-larger particle accelerators, colliders, and detectors increased unabated.
The Cold War rationale for building these expensive scientific facilities had declined during the 1970s, after the administration of Richard Nixon and the Soviet government of Leonid Brezhnev tacitly agreed to détente in their relationship, thus encouraging scientific exchanges and joint projects such as the 1975 docking of the Apollo and Soyuz space capsules. High-energy physics had enjoyed a privileged status under the old AEC, whose General Advisory Council often made decisions in secret about proposed projects — which Congress then debated in closed sessions of the Joint Committee on Atomic Energy. But after the Energy Reorganization Act of 1974, the AEC ceased to exist. And congressional jurisdiction over energy projects was now assigned to separate House and Senate Appropriations Subcommittees on Energy and Water Development. Their members were much more interested in garnering lucrative projects for their districts and states than in helping to foster the research productivity of a relatively small (but still influential) group of physicists who worked mainly at national laboratories in California, Illinois, and New York. During the mid-to-late 1970s, US high-energy physics lost its privileged status as the flagship of the AEC fleet and became just one among many petitioners for federal largesse.
In Europe, by contrast, high-energy physics continued to enjoy its special status well into the 1980s. Rather than becoming a marquee attraction of the Cold War competition between the US and USSR, it increasingly served a prominent role as the highest expression of postwar European integration. Ministers could point to CERN as a shining example of how competing European countries can successfully cooperate with one another on joint projects of scientific and cultural merit. And CERN had over the years evolved a governing structure, its Council, that effectively insulated its operations from the political vicissitudes of the participant nations. Combined with the widely shared European desire to foster its intellectual vitality and success, CERN's governance meant it could rely on steady funding of major projects, which remained firmly under control of the physicist-managers running the laboratory.
The 1970s were also a decade of economic and industrial disruption in the United States. After the country abandoned the gold standard in 1971, the dollar plummeted against major foreign currencies, while the price of gold, oil, and other commodities soared. After the Arab embargo of 1973–74, oil and gasoline prices more than tripled, triggering a deep recession in the United States. European and Japanese automakers took major market shares away from US firms, which proved unable to manufacture small, fuel-efficient vehicles economically. And Japanese firms such as Panasonic and Sony grabbed most of the US market for consumer electronics from their American competitors. As the decade ended, the nation was deep in the throes of stagflation, with a flat or declining gross domestic product compounded by surging unemployment and double-digit inflation.
Amid such trying circumstances, it was difficult to maintain a healthy US high-energy physics program, given the ever-increasing price tags of the machines and equipment required to do this expensive research. Already constrained by the costs of the Vietnam War and of funding the Great Society programs of President Lyndon B. Johnson, the US budget for high-energy physics had peaked in 1970 during construction of the National Accelerator Laboratory (later renamed Fermilab) near Chicago. After its $250 million particle accelerator — extending four miles in circumference across the Illinois plains and boosting protons to energies up to 200 GeV — was completed in 1972, funding for the discipline fell by almost 50 percent in real terms by mid-decade. And many other programs — at Brookhaven, SLAC and elsewhere — had been severely constricted during Fermilab's construction.
Funding for US high-energy physics began to stabilize in the late 1970s, albeit haltingly. What might have seemed a modest budget increase when appropriated by Congress before the start of a fiscal year would often be eroded so much by inflation that it ended up being a decrease in terms of constant dollars by the end of that year. Thus the true budget for high-energy physics grew only marginally, if at all, in the latter half of the decade. New projects needed to keep the major national labs at the research frontier — such as SLAC's electron-positron collider PEP, Brookhaven's proposed Isabelle proton-proton collider, and a Fermilab project dubbed the Energy Doubler (later renamed the Energy Saver and then the Tevatron) — suffered from inevitable delays and postponements in this difficult funding climate.
And, despite the emergence of the Standard Model paradigm in the 1970s, there was still plenty of important research to be addressed. Besides the search for the all-important W and Z bosons, the carriers of the weak nuclear force in this theory, at least one predicted quark known as the top quark remained undiscovered. By 1980 nearly every high-energy physicist expected that these weighty particles would eventually turn up after particle colliders managed to attain the extremely high energies needed to create them (according to Einstein's E = mc2). Producing and detecting sufficient numbers of these particles to prove their existence was a primary rationale for constructing ever larger and costlier equipment.
Another all-important target on high-energy physicists' wish lists was the so-called Higgs boson, named after the British physicist Peter Higgs, who conceived it in 1964 as the consequence of theories about how the W and Z bosons could acquire large masses. In the following decade, the Higgs boson became the capstone of the emerging Standard Model, providing the consensus explanation of why quarks, leptons, and gauge bosons have the wide variety of masses observed. And it had to be unlike all of the other particles in that it could have no spin. In addition, particle theorists could not confidently predict what the Higgs mass might be — other than to say that it must come in below about 1,000 GeV, or 1 TeV. Otherwise, something was amiss with the Standard Model (see appendix 1 for more details).
If the Higgs boson were indeed that massive, designing and constructing a collider powerful enough to create it in sufficient numbers to detect it stretched the imaginations of machine builders throughout the high-energy physics community. It clearly had to be a multi-TeV collider involving protons and perhaps antiprotons. Because protons and antiprotons are composed of quarks and gluons, collisions between these fundamental constituents occur at energies only about a tenth that of their parent particles, whose energies must thus be ten times higher to compensate (see appendix 1). Given the limitations on the possible strengths of magnetic fields, even with the most advanced superconducting magnets, such a gargantuan machine able to produce particles with masses up to 1 TeV would extend tens of miles or kilometers in circumference and cost billions of scarce US dollars or Swiss francs (SFr, also written CHF). But the most daring leaders of the US high-energy physics community were undaunted by such awesome challenges — whether scientific, technological, economic, or political — as the new decade began.
DREAMS OF A WORLD ACCELERATOR
By the early 1980s, a constellation of accelerator visions had coalesced, partly out of the aspirations of high-minded physicists eager to advance their science while offering a contrasting example to the ideological Cold War confrontation. Since the mid-1950s, they had slowly elaborated the idea of a very large accelerator to serve physicists from countries on both sides of the Iron Curtain, which would share in the huge cost of such a mammoth machine, too expensive for any single nation to build. The organization of CERN earlier that decade served as an important step toward international cooperation in physics, for it demonstrated how individual nations could successfully collaborate on a major scientific project. In 1957 the International Union of Pure and Applied Physics (IUPAP) established a commission to help "encourage international collaboration among the various high-energy laboratories to ensure the best use of these large and expensive installations."
Soon physics meetings throughout the world included discussion of such an ambitious worldwide cooperative accelerator project to serve as a possible model for peaceful international collaboration. In September 1959, USSR Premier Khrushchev's successful summit meeting with US President Eisenhower encouraged dialogue about cooperative scientific projects. Eight months later a delegation of physicists that included Edward Lofgren of Lawrence Berkeley Laboratory (LBL) and Robert R. Wilson of Cornell visited the USSR to explore the joint construction of a large accelerator. But the opportunity for collaboration evaporated after the Soviet Union downed an American U-2 reconnaissance plane over its territory in May 1960.
A small group of idealistic physicists continued to discuss the dream that, as Wilson later wrote, "in building and operating a World Laboratory we would not only be exploring nature, but we also might be exploring some of the ingredients of peace." An August 1960 meeting organized by Wilson to consider 1,000 GeV (or 1 TeV) accelerators in a global context initiated serious discussions on the design of such a large international facility. Its basic technical concept would eventually serve as a starting point for the design of the Superconducting Super Collider.
A window for discussing international cooperation opened during the 1970s period of détente. Nixon and Brezhnev's historic agreement of June 1973 identified basic research on the fundamental properties of matter as one of three areas that were particularly useful for "expanded and strengthened cooperation for mutual benefit, equality and reciprocity between the U.S. and the U.S.S.R." In addition, scientific cooperation and the free flow of information were included as basic human rights in the August 1975 Helsinki Accords. By the mid-1970s, therefore, initial steps toward a World Accelerator had occurred, but there were still no concrete plans for building one; many of the physicists involved in the discussions of international cooperation were beginning to lose patience.
(Continues…)
Excerpted from "Tunnel Visions"
by .
Copyright © 2015 The University of Chicago.
Excerpted by permission of The University of Chicago Press.
All rights reserved. No part of this excerpt may be reproduced or reprinted without permission in writing from the publisher.
Excerpts are provided by Dial-A-Book Inc. solely for the personal use of visitors to this web site.