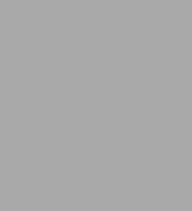
The Unknown Universe: The Origin of the Universe, Quantum Gravity, Wormholes, and Other Things Science Still Can't Explain
272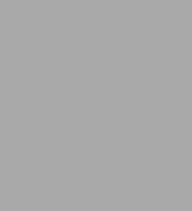
The Unknown Universe: The Origin of the Universe, Quantum Gravity, Wormholes, and Other Things Science Still Can't Explain
272Hardcover
-
PICK UP IN STORECheck Availability at Nearby Stores
Available within 2 business hours
Related collections and offers
Overview
Product Details
ISBN-13: | 9781601630032 |
---|---|
Publisher: | Red Wheel/Weiser |
Publication date: | 04/21/2008 |
Pages: | 272 |
Product dimensions: | 5.40(w) x 8.30(h) x 1.00(d) |
About the Author
Read an Excerpt
CHAPTER 1
Cosmic Acceleration
Darkness there, and nothing more.
Poe's famous midnight thinker stared into the darkness, seeing nothing, nothing except what his imagination allowed. Now we stare out into the darkness, using every machination of modern technology, but are still limited by our imaginations. The telescope expands our view of the universe to an inconceivable size, and allows us to see into the cold blackness that stretches back to the beginning of time. Today, as we peer into the dawn of the universe, we are bringing back questions from the past like mysterious fossils etched in bedrock. Our most cherished notions about the nature of matter are challenged, and Einstein's famous theory of general relativity, the cornerstone of our understanding of gravity, is placed under the microscope.
These recent observations are not the first to rock our view of the cosmos, but, unlike the previous explosive revelations, these remain unsolved. The ultimate solution may cause us to abandon our most esteemed model of the universe, or may prove the existence of a new kind of matter never seen before.
To appreciate the full effect, let's step back and soak up a little history. It is fun to ask the question, "Where on Earth was helium discovered?" It is fun because the answer is nowhere — nowhere on Earth, that is. Helium was discovered on the sun, even though we never landed there and never will. This is because elements are like hapless criminals, leaving their fingerprints on everything they touch. To understand the details we must take a detour through the fascinating world of spectroscopy.
Start with hydrogen, the simplest atom. If you fill a glass tube with hydrogen and force an electric current through it, the gas will radiate, producing a dull glow. This is precisely what happens in the garish neon signs that emit the familiar sanguine radiance hawking everything from Jell-O to Mr. Peanut.
? Question:
Suppose we take this light, from hydrogen, and pass it through a prism. What happens? (I will give you some answers, three of which are wrong, just to get the wheels rolling.)
[] 1. Nothing.
[] 2. It is refracted (bent), but still looks bluish.
[] 3. It explodes into the colors of a rainbow (the same thing that would happen to sunlight).
[] 4. Four distinct colors emerge, each one refracted by a different amount.
This experiment is repeated countless times every year in every college physics labs, but as the 19th century entered its retirement years, this conundrum had physicists questioning their most cherished notions. It was as shocking as waking up one morning to a green sky. When explanations were ultimately found, it was so hard on some physicists that they refused to accept the new ideas. In fact, this era gave birth to the famous quote, "Physics progresses gravestone by gravestone."
If you gave this question to the physicists back then, before they did the experiment, they would circle number 3 as their prediction. I hear them pontificating, as they stroke their beards, "Sunlight breaks into the rainbow, and so does light from a hot iron poker, or light from a brilliant light-bulb filament, and so would light from any other substance. Get with the program."
Maybe the last sentence is a stretch, but the correct answer knocked their whiskers off. To put it in perspective, recall the lesson of Copernicus and Ptolemy: Old Claudius Ptolemaeus had us believing that everything revolved around the Earth, and no one ever shook things up us as much as Nicolaus Copernicus, telling us we are spinning wildly on an axis. The Church tried the usual — burning people at the stake (Giordano Bruno was burned at the stake in Italy in 1600) — but even its fiery dogma of ignorance was broken down by truth and reality. Copernicus's upheaval was nothing compared to the hydrogen issue. Luckily the Church was unable to burn anybody this time, so the revolution went a little more quickly, stymied slightly by old physicists clinging to their lost reality. (I sometimes worry I am clinging to a lost reality.)
In the actual experiment, the light is passed through a thin slit in order to collimate the beam. For this reason the light that is observed appears as thin lines — exactly four thin lines. If you would like to see the real thing, stop off at the physics department of any university; they will be glad to show you. Just ask to see the spectrum of hydrogen from a gas discharge tube. Or, check out http://cfa-www.harvard.edu/seuforum/galSpeed/.
So why did these thin little lines reduce the Copernican Revolution to just another day at the beach? These lines, along with a few other experiments, gave birth to quantum mechanics, a view of nature that boggles the mind. While allowing us to understand our world, at the same time it severely limits our knowledge of the universe.
The essential point for now is that, according to quantum mechanics, energy is quantized. The hydrogen atom can absorb and emit light, but only by undergoing transitions through a discrete number of energy levels. It is like sitting in an airplane. You can move up or back, but you must take a seat, so your net displacement is quantized. In fact, I once interviewed a hydrogen atom who was kind enough to explain all this. This quantization means that light can only be emitted at certain, discrete wavelengths, which you saw in Figure 1.1. These wavelengths are different for each atom. For example, the spectrum of helium consists of more than four lines and looks nothing like Figure 1.1.
We are making good progress. Now you can understand how helium was discovered on the sun: The spectrum was observed. But that is still not my main point. My main point is this: Elements can be discovered by observing the spectra of their light, and the spectra are unique.
While physicists have continually brought us to ever smaller scales, down to protons and neutrons, and now even smaller, to the quarks that are the building blocks of these teeny particles, astronomers have been making our universe larger and larger. The first modern explosion came with the discovery of an island universe, which I saw one night in Massachusetts, riding back from a lecture given by John Wheeler, one of Einstein's most famous students. I was studying generalizations of Einstein's theory and was very grateful for the encouragement Wheeler gave me. He had spoken at Mt. Holyoke College, and we were driving back to RPI (Rensselaer Polytechnic Institute, in Troy, New York), where I was a graduate student. We stopped somewhere on a peak in the Berkshires (there are no restrooms nearby), and Frank Ferrandino, an astrophysics student, was looking up as we were looking down. He was pointing out the constellations, and showed us how to find Andromeda, which we saw with our naked eyes. You have to know just where to look, and it is faint, but you can tell it is not quite a point as are the other stars. Even with a telescope it is nebulous, a fact good enough to justify one of the early names, nebula. Then came the Great Debate. Two astronomers duked it out: In one corner was Harlow Shapley, saying our Milky Way was the whole enchilada, while Heber Curtis was saying it was time to think outside the box. I am taking a little poetic license here; the actual debate, in 1920, took place at the Smithsonian Museum of Natural History, and the idea of another galaxy was tough to swallow back then. Copernicus was hard enough, and now this? Years gave Curtis the victory (see the technical note at the end of the chapter about Cepheid variables), and nowadays we know there are many billions of galaxies, and galaxies such as our beloved Milky Way are more common than candy bars on Halloween. Another name for these blurry spots, as others came to found, was island universe — my favorite — but this moniker was also doomed.
This little outing in the Berkshires was quite a moment for me, seeing another galaxy for the first time, but it was broken by an angry motorist, honking his horn to assuage his annoyance. As he passed, the pitch of his horn lowered — an effect heard all the time — but being physicists (or, back then, physicist wannabes), we plunged into a discussion of the Doppler effect, which includes the mathematical formulas that describe the lowering of pitch, or increase in wavelength, as the source of the sound moves away from us.
By the way, and back to galaxies, this brings up another question.
? Question:
How do you find out what these mysterious clouds of light are made of?
[] 1. Send out a probe, collect some stuff, and bring it back to Earth to analyze.
[] 2. Ask an astrologer.
[] 3. Send out a manned probe, after we settle on Mars.
[] 4. Examine the spectra.
Number 1 would take hundreds of millions of years, if we could do it at all. I will not even address number 2, and number 3 is another dig. Anyway, I know you got the right answer, and astronomers wasted no time doing just that: examining the spectra. They did it for every galaxy they found, and just as one blazing enigma yielded to explanation, more provocative ones ignited in their place.
Vesto Melvin Slipher is a name you do not hear every day. I grabbed his mug from the Internet because it is also a face you do not see every day. He was born in Indiana, studied at the University of Indiana, and became famous during the second decade of the 20th century. Well, not that famous, but in my book he should be. He was studying the spectra of light from distant galaxies and found something curious. The spectra looked like known elements, but the lines were a little off. Look at the hydrogen spectrum again. The red line corresponds to a wavelength of precisely 656 nanometers (a billion nanometers is a meter). Slipher saw a wavelength just slightly bigger than 656 nm. The other lines were shifted in the same manner, each to a longer wavelength. Figure 1.3 shows another example of a spectrum that is shifted. Note that the lines are dark, which means we are looking at an absorption spectrum (see the technical notes at end of the chapter). The lines on the right are moved up toward the red, so we call this redshifted.
Something as extraordinary as this calls for a question, so here it is.
? Question:
What causes the spectrum to be redshifted?
[] 1. Dirt on the telescope lens.
[] 2. A mischievous God, like Zeus throwing lightning bolts.
[] 3. The ozone.
[] 4. The galaxies are moving away from us.
[] 5. Light gets tired, traveling zillions of miles and all.
When I taught astronomy, I usually gave easy tests, but every once in a while a few students were determined to undermine my facile approach. Refusing to come to class, they found trouble in the simplest questions, but often relied on "ozone" as the panacea to all issues. I do not know why this is, but that is why I included it — and yes, it is the wrong answer. Number 2 could be argued, but let's try science instead. This question shows how devious I can be — I already gave you the answer! Remember the angry motorist in the Berkshires? The pitch is lowered, or the wavelength of sound is increased, when he is moving away from us. It is called the Doppler effect, and it holds for light as well as sound. Once again, number 4 is the correct answer.
Because nothing exists in isolation except isolation itself, I must bring in another thread of the fabric of revolution: Mercury. I am not speaking about the speedy messenger long before FedEx; I am referring to the speedy planet, zooming around the sun once every 88 days. Too small to have an atmosphere, its ravaged surface exposes the callous ferocity of our solar system — but I wander. I want to mention its orbit, which is elliptical. The point of its orbit closest to the sun is called the perihelion. One of the greatest triumphs of the human genius, Isaac Newton, discovered the universal law of gravitation and the equation of motion, and proved that Mercury (as all planets) speeds around the sun in an elliptical orbit (nowadays, physics majors discover this in their sophomore or junior years). The theory also predicts that the perihelion is fixed in space and time.
All this amazing theory, corroborated by observations as plentiful as dandelions in my backyard, made many people very happy. But happiness, like a rainbow, never lasts, and soon Mercury proved to be a pesky problem. If I were writing this book in 1910, I would definitely include pesky Mercury as a chapter, for the perihelion of Mercury moves. It precesses around the sun 1,043 seconds of arc every century.
It was not too hard to realize that the other planets whirling around the sun produce a modest tug on Mercury. In fact, calculations show that this effect will produce a precession of the perihelion of 1,000 seconds of an arc per century — 1,000 seconds, but no more.
You see the quandary of the old-timers — how on earth to account for those 43 seconds — but this was not the first time astronomers were left in the dark. Years earlier Uranus was known to be an outlaw, breaking the gravitational law of Newton. Times like these are quite fertile for theoreticians like me. We unleash our imaginations, creating new theories and models from thin air, hoping we shed some light on nature's secrets. It was the same back then, and Newton's law of gravitation was quickly put on trial. George Airy, a British astronomer and mathematician with more medals than a four-star general, theorized that Newton's inverse square law did not hold at large distances. After all, just because a theory works on one scale does not mean it applies to all. In fact, when applied to the tiny atomic world, Newton's laws crash and burn like the Tunguska comet (see the technical notes). But there was another explanation for the errant behavior of Uranus: Perhaps there is something else out there yanking the great mass off course. Taking this idea and running with it, Urbain Jean Joseph Le Verrier (born in 1811 in Saint-Lô, France) and John Cough Adams (born in 1819 in Lidcott, England) actually used Newton's laws to determine where such a planet could be.
Armed with hard calculations, William Herschel and sister Caroline swung their telescope where the theoreticians predicted, and, before another perplexing mystery could pop up, it was found: The discovery of Georgium Sidus was made. What, you never heard of it? That's because no one could stand Herschel's putative name (except maybe King George), so Neptune took over, following the rule that no planet is allowed two words. The discovery is a fascinating story, but I must get on with my own little story.
Back to Mercury and its errant orbit. What could be a more natural explanation than the existence of another planet? It would be so close to the sun that it would be very hard to see, which explains why we had not. Our friend Le Verrier had such good luck in predicting Neptune, it prompted him to work diligently on the Mercury issue, pondering those 43 seconds of precession. Le Verrier was a terrific mathematician, and he was able to calculate that a planet 13 million miles from the sun (the earth is 93 million) that streaks around once every 20 days would fit the requirements. That same year, 1859, a French country doctor, Lescarbault, who had a modest telescope, saw the planet. By now it was clear that it needed a name. Le Verrier knew the fate of Georgium Sidus, and stuck to the rules this time: He came up with Vulcan, and by 1860 awards were flying faster than the new planet, and soon other sightings were published.
You did not know this? Let me pose a question.
? Question:
Why is Vulcan, the planet closest to the sun, unknown today?
[] 1. It is too small to be considered a bona fide planet.
[] 2. Le Verrerier needed cash, and created a hoax.
[] 3. It is classified Top Secret by the Air Force, who know all about it, as well as the little Vulcans that landed in Roswell.
[] 4. Its discoverers were seeing things.
The definitive answer did not come along until the next century, so let us go to the first decades of the 20th century, when two more famous names arise: Einstein and Hubble. Everyone knows about Einstein, and most people know about Hubble too, because the orbiting telescope is named in his honor. These guys were very active during this period, and Einstein came up with his general theory of relativity in 1915. This theory is a theory of gravity that turns out to be better than Newton's, and more accurate too. In fact, it predicts that the perihelion of planetary orbits should precess, and it even predicts the amount of precession. Would you like to guess what it predicts for Mercury? Yup, 43 seconds of an arc per century, right on the nose. And that was the end of Vulcan, and no one has seen it since.
Einstein's theory, GR, can not only be applied to planets, but it can also be used to model the entire universe in which we live. This is so cool I cannot understand why everybody does not become a physicist, but experience shows me they do not, so I will go on. During the mid-1920s it was shown that GR can describe a universe that is expanding or contracting (but not static). At the same time, Edwin Hubble was thinkingabout Vesto's red shifts. Actually, Hubble was doing a lot more than thinking. Working at Mount Wilson Observatory, he made measurements, collected data, and came across the most startling discovery of the century (actually, there are a few "most startling discoveries," but I take poetic license). Here is what he said: "The results establish a roughly linear relation between velocities and distances among nebulae for which velocities have been previously published, and the relation appears to dominate the distribution velocities."
I am no Hubble, but here is how I would say it: "Cosmic secrets have been unearthed, shattering our view of the universe. Observations show that the cosmos is exploding apart, as distant galaxies race away faster than a soccer mom in a van. Even more astounding: the further the galaxy, the faster it goes."
(Continues…)
Excerpted from "The Unknown Universe"
by .
Copyright © 2008 Richard Hammond.
Excerpted by permission of Red Wheel/Weiser, LLC.
All rights reserved. No part of this excerpt may be reproduced or reprinted without permission in writing from the publisher.
Excerpts are provided by Dial-A-Book Inc. solely for the personal use of visitors to this web site.
Table of Contents
Chapter One Cosmic Acceleration,
Chapter Two Dark Matter,
Chapter Three The Cosmic Ray Paradox,
Chapter Four Renormalization,
Chapter Five The Higgs Particle,
Chapter Six Quantum Gravity,
Chapter Seven Ashes to Ashes — Things We Know,
Chapter Eight String Theory,
Chapter Nine Origin of the Universe,
Chapter Ten Mysterinos,
Epilogue,
Notes,
Bibliography,
Suggested Reading,
Index,
About the Author,