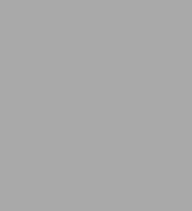
The Number Sense: How the Mind Creates Mathematics
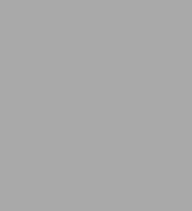
The Number Sense: How the Mind Creates Mathematics
eBook
Available on Compatible NOOK devices, the free NOOK App and in My Digital Library.
Related collections and offers
Overview
Product Details
ISBN-13: | 9780199881055 |
---|---|
Publisher: | Oxford University Press |
Publication date: | 10/30/1999 |
Sold by: | Barnes & Noble |
Format: | eBook |
File size: | 4 MB |
About the Author
Stanislas Dehaene is Research Affiliate at the Institute de la Sante et de la Recherche Medicale, in Paris, France.
Read an Excerpt
CHAPTER ONE
Talented and Gifted Animals
One stone,
two houses
three ruins
four gravediggers,
one garden,
some flowersOne raccoon.
Jacques Prevert, Inventaire
Books on natural history have recounted the following anecdote since the eighteenth century:
A nobleman wanted to shoot down a crow that had built its nest atop a tower on his domain. However, whenever he approached the tower, the bird flew out of gun range, and waited until the man departed. As soon as he left, it returned to its nest. The man decided to ask a neighbor for help. The two hunters entered the tower together, and later only one of them came out. But the crow did not fall into this trap and carefully waited for the second man to come out before returning. Neither did three, then four, then five men fool the clever bird. Each time, the crow would wait until all the hunters had departed. Eventually, the hunters came as a party of six. When five of them had left the tower, the bird, not so numerate after all, confidently came back, and was shot down by the sixth hunter.
Is this anecdote authentic? Nobody knows. It is not even clear that it has anything to do with numerical competence: For all we know, the bird could have memorized the visual appearance of each hunter rather than their number. Nevertheless, I decided to highlight it because it provides a splendid illustration of many aspects of animal arithmetic that are the subject of this chapter. First, in many tightly controlled experiments, birds and many other animal species appear to be able to perceive numerical quantities without requiring special training. Second, this perception is not perfectly accurate, and its accuracy decreases with increasingly larger numbers; hence the bird confounding 5 and 6. Finally, and more facetiously, the anecdote shows how the forces of Darwinian selection also apply to the arithmetical domain. If the bird had been able to count up to 6, it would perhaps never have been shot! In numerous species, estimating the number and ferocity of predators, quantifying and comparing the return of two sources of food, are matters of life and death. Such evolutionary arguments should help make sense of the many scientific experiments that have revealed sophisticated procedures for numerical calculation in animals.
A Horse Named Hans
At the beginning of this century, a horse named Hans made it to the headlines of German newspapers. His master, Wilhelm von Osten, was no ordinary circus animal trainer. Rather, he was a passionate man who, under the influence of Darwin's ideas, had set out to demonstrate the extent of animal intelligence. He wound up spending more than a decade teaching his horse arithmetic, reading, and music. Although the results were slow to come, they eventually exceeded all his expectations. The horse seemed gifted with a superior intelligence. It could apparently solve arithmetical problems and even spell out words!
Demonstrations of Clever Hans's abilities often took place in von Osten's yard. The public would form a half-circle around the animal and suggest an arithmetical question to the trainer--for instance, "How much is 5 plus 3?" Von Osten would then present the animal with five objects aligned on a table, and with three other objects on another. After examining the "problem," the horse responded by knocking on the ground with its hoof the number of times equal to the total of the addition. However, Hans's mathematical abilities far exceeded this simple feat. Some arithmetical problems were spoken aloud by the public, or were written in digital notation on a blackboard, and Hans could solve them just as easily (Figure 1.1). The horse could also add two fractions such as 2/5 and 1/2 and give the answer 9/10 by striking nine times, then ten times with its hoof. It was even said that to the question of determining the divisors of 28, Hans came out very appropriately with the answers 2, 4, 7, 14, and 28. Obviously, Hans's number knowledge surpassed by far what a school teacher would expect today of a reasonably bright pupil!
In September 1904, a committee of experts, among whom figured the eminent German psychologist Carl Stumpf, concluded after an extensive investigation that Hans's feats were real and not a result of cheating. This generous conclusion, however, did not satisfy Oskar Pfungst, one of Stumpf's own students. With von Osten's help--the master was fully convinced of his prodigy's superior intelligence--he began a systematic study of the horse's abilities. Pfungst's experiments, even by today's standards, remain a model of rigor and inventiveness. His working hypothesis was that the horse could not but be totally inept in mathematics. Therefore, it had to be the master himself, or someone in the public, who knew the answer and sent the animal a hidden signal when the target number of strokes had been reached, thus commanding the animal to stop knocking with its hoof.
To prove this, Pfungst invented a way of dissociating Hans's knowledge of a problem from what its master knew. He used a procedure that differed only slightly from the one described above. The master watched carefully as a simple addition was written in large printed characters on a panel. The panel was then oriented toward the horse in such a way that only it could see the problem and answer it. However, on some trials, Pfungst surreptitiously modified the addition before showing it to the horse. For instance, the master could see 6+2 whereas in fact the horse was trying to solve 6+3.
The results of this experiment, and of a series of follow-up controls, were clear-cut. Whenever the master knew the correct response, Hans got the right answer. When, on the contrary, the master was not aware of the solution, the horse failed. Moreover, it often produced an error that matched the numerical result expected by its master. Obviously, it was von Osten himself, rather than Hans, who was finding the solution to the various arithmetical problems. But how then did the horse know how to respond? Pfungst eventually deduced that Hans's truly amazing ability lay in detecting minuscule movements of its master's head or eyebrows that invariably announced the time to stop the series of knocks. In fact, Pfungst never doubted that the trainer was sincere. He believed that the signals were completely unconscious and involuntary. Even when von Osten was absent, the horse continued to respond correctly: Apparently, it detected the buildup of tension in the public as the expected number of hoof strokes was attained. Pfungst himself could never eliminate all forms of involuntary communication with the animal, even after he discovered the exact nature of the body clues it used.
Pfungst's experiments largely discredited demonstrations of "animal intelligence" and the competence of self-proclaimed experts such as Stumpf who had blindly subscribed to them. Indeed the Clever Hans phenomenon is still taught in psychology classes today. It remains a symbol of the pernicious influence that experimenter expectations and interventions, however small, may have on the outcome of any psychological experiment, with humans or with animals. Historically, Hans's story has played a crucial role in shaping the critical minds of psychologists and ethologists. It has drawn attention to the necessity for a rigorous experimental design. Since an essentially invisible stimulation as brief as the blink of an eye can influence the performance of animals, a well-designed experiment has to be devoid, from the start, of any possible source of errors. This lesson was particularly well-received by behaviorists, such as B. F. Skinner, who dedicated a large amount of work to the development of rigorous experimental paradigms for the study of animal behavior.
Unfortunately, Hans's exemplary case has also had more negative consequences on the development of psychological science. It has imposed an aura of suspicion onto the whole area of research on the representation of numbers in animals. Ironically, scientists now meet every single demonstration of numerical competence in animals with the same raised eyebrows that served as a cue to Hans! Such experiments are immediately associated, consciously or not, with Hans's story and are therefore suspected of a basic flaw in design, if not of downright forgery. This is an irrational prejudice, however. Pfungst's experiments showed only that Hans's numerical abilities were a fluke. By no means did they prove that it is impossible for an animal to understand some aspects of arithmetic. For a long time, however, the scientist's attitude was to systematically look for some experimental bias that might explain animal behavior without resorting to the hypothesis that animals have even an embryonic knowledge of calculation. For a while, even the most convincing results failed to convince anyone. Some researchers even preferred to attribute to animals mysterious abilities such as "rhythm discrimination" faculty, for instance, rather than admit that they could enumerate a collection of objects. In brief, the scientific community tended to throw out the baby with the bath water.
Before turning to some of the experiments that finally convinced all but the most skeptical of researchers, I would like to conclude Hans's story with a modern anecdote. Even today, the training of circus animals rests on methods rather similar to Hans's trick. If you ever see a show in which an animal adds numbers, spells words, or some surprising deed of this kind, you may safely bet that its behavior rests, like Hans's, on a hidden communication with its human trainer. Let me stress again that such communication need not be intentional. The trainer is often sincerely convinced of his pupil's gifts. A few years ago, I came upon an amusing article in a local Swiss newspaper. A journalist had visited the home of Gilles and Caroline P., whose poodle, named Poupette, seemed extraordinarily gifted in mathematics. Figure 1.2 shows Poupette's proud owner presenting his faithful and brilliant companion with a series of written digits that it was supposed to add. Poupette responded without ever making an error by tapping on its master's hand with its paw the exact number of times required, and then licking the hand after the correct count had been reached. According to its master, the canine prodigy had required only a brief training period, which led him to believe in reincarnation or some similar paranormal phenomenon. The journalist, however, wisely noted that the dog could react to subtle cues from the master's eyelids, or to some tiny motions of his hand when the correct count was reached. So this was indeed a case of reincarnation after all: the reincarnation of Clever Hans's stratagem, of which Poupette's story constituted, a century later, an astonishing replication.
Rat Accountants
Following the Hans episode, several renowned American laboratories developed research programs on animal mathematical abilities. Many such projects failed. A famous German ethologist named Otto Koehler, however, was more successful. One of his trained crows, Jacob, apparently learned to choose, among several containers, the one whose lid bore a fixed number of five points. Because the size, the shape, and the location of the points varied randomly from trial to trial, only an accurate perception of the number 5 could account for this performance. Nevertheless, the results achieved by Koehler's team had little impact, partly because most of their results were published only in German, and partly because Koehler failed to convince his colleagues that all possible sources of error, such as unintentional experimenter communication, olfactory cues or the like, had been excluded.
In the 1950s and 1960s, Francis Mechner, an animal psychologist at Columbia University, followed by John Platt and David Johnson at the University of Iowa, introduced a very convincing experimental paradigm that I shall schematically describe here. A rat that had been temporarily deprived of food was placed in a closed box with two levers, A and B. Lever B was connected to a mechanical device that delivered a small amount of food. However, this reward system did not work at once. The rat first had to repeatedly press lever A. Only after it had pressed for a fixed number of times n on lever A could it switch to lever B and get its deserved treat. If the rat switched too early to lever B, not only did it fail to get any food, but it received a penalty. On different experiments, the light could go off for a few seconds, or the counter was reset so that the rat had to start all over again with a new series of n presses on lever A.
How did rats behave in this rather unusual environment? They initially discovered, by trial and error, that food would appear when they pressed several times on lever A, and then once on lever B. Progressively, the number of times that they had to press was estimated more and more accurately. Eventually, at the end of the learning period, the rats behaved very rationally in relation to the number n that had been selected by the experimenter. The rats that had to press four times on lever A before lever B would deliver food, did press it about four times. Those that were placed in the situation where eight presses were required, waited until they had produced about eight squeezes, and so on (see Figure 1.3). Even when the requisite number was as high as twelve or sixteen, those clever rat accountants continued to keep their registers up to date!
Two details are worth mentioning. First, the rats often squeezed lever A a little more than the minimum required--five times instead of four, for instance. Again, this was an eminently rational strategy. Since they received a penalty for switching prematurely to lever B, the rats preferred to play it safe and press lever A once more rather than once less. Second, even after considerable training, the rats' behavior remained rather imprecise. Where the optimal strategy would have been to press lever A exactly four times, the rats often pressed it four, five, or six times, and on some trials they even squeezed it three or seven times. Their behavior was definitely not "digital," and variation was considerable from trial to trial. Indeed, this variability increased in direct proportion to the target number that the rats estimated. When the target number of presses was four, the rats' responses ranged from three to seven presses, but when the target was sixteen, the responses went from twelve to twenty-four, thus covering a much larger interval. The rats appeared to be equipped with a rather imprecise estimation mechanism, quite different from our digital calculators.
At this stage, many of you are probably wondering whether I am not too liberal in attributing numerical competence to rats, and whether a simpler explanation of their behavior might not be found. Let me first remark that the Clever Hans effect cannot have any influence on this type of experiment because the rats are isolated in their cages and because all experimental events are controlled by an automated mechanical apparatus. However, is the rat really sensitive to the number of times the lever is pressed, or does it estimate the time elapsed since the beginning of a trial, or some other nonnumerical parameter? If the rat pressed at a regular rate, for instance once per second, then the above behavior might be fully explained by temporal rather than numerical estimation. While pressing on lever A, the rat would wait four, eight, twelve, or sixteen seconds, depending on the imposed schedule, before switching to lever B. This explanation might be considered simpler than the hypothesis that rats can count their movements--although, in fact, estimating duration and numbers are equally complex operations.
To refute such a temporal explanation, Francis Mechner and Laurence Guevrekian used a very simple control: They varied the degree of food deprivation imposed on the rats. When the rats are really hungry, and therefore eager to obtain their food reward as fast as possible, they press the levers much faster. Nevertheless, this increase in rate has absolutely no effect on the number of times they press the lever. The rats that are trained with a target number of four presses continue to produce between three and seven presses, while the rats trained to squeeze eight times continue to squeeze about eight times, and so on. Neither the average number of presses, nor the dispersion of the results, are modified with higher rates. Obviously, a numerical rather than a temporal parameter drives the rats 'behavior.
A more recent experiment by Russell Church and Warren Meck, at Brown University, demonstrates that rats spontaneously pay as much attention to the number of events as to their duration. In Church and Meck's experiment, a loudspeaker placed in the rats' cage presented a sequence of tones. There were two possible sequences. Sequence A was made up of two tones and lasted a total of two seconds, whereas sequence B was made up of eight tones and lasted eight seconds. The rats had to discriminate between the two melodies. After each tune, two levers were inserted in the cage. To receive a food reward, the rats had to press the left lever if they had heard sequence A, and the right if they had heard sequence B (see Figure 1.4).
Several preliminary experiments had shown that rats placed in this situation rapidly learned to press the correct lever. Obviously, they could use two distinct parameters to distinguish A from B: the total duration of the sequence (two versus eight seconds) or the number of tones (two versus eight). Did rats pay attention to duration, number, or both? In order to find out, the experimenters presented some test sequences in which duration was fixed while number was varied, and others in which number was fixed while duration was varied. In the first case, all sequences lasted four seconds, but were made up of from two to eight tones. In the second case, all sequences were made up of four tones, but duration extended from two to eight seconds. On all such test sequences, the rats always received a food reward, regardless of the lever they picked. In anthropocentric terms, the researchers were simply asking what these new stimuli sounded like to the rats, without letting the reward interfere with their decision. The experiment therefore measured the rats' ability to generalize previously learned behaviors to a novel situation.
The results are clear-cut. Rats generalized just as easily on duration as on number. When duration was fixed, they continued to press the left lever when they heard two tones, and the right lever when they heard eight tones. Conversely, when number was fixed, they pressed left for two-second sequences, and right for eight-second sequences. But what about intermediate values? Rats apparently reduced them to the closest stimulus that they had learned. Thus, the new three-tone sequence elicited the same response as the two-tone sequence used for training, while sequences with five or six tones were classified just as the original sequence of eight tones had been. Curiously, when the sequence comprised just four tones, the rats could not decide whether they should press left or right. For a rat, four appears to be the subjective midpoint between the numbers two and eight!
Keep in mind that the rats did not know during training that they would be tested subsequently with sequences that varied in duration or in number of tones. Hence, this experiment shows that when a rat listens to a melody, its brain simultaneously and spontaneously registers both the duration and the number of tones. It would be a serious mistake to think that because these experiments use conditioning, they somehow teach the rats how to count. On the contrary, rats appear on the scene with state-of-the-art hardware for visual, auditory, tactile, and numerical perception. Conditioning merely teaches the animal to associate perceptions that it has always experienced, such as representations of stimulus duration, color, or number, with novel actions such as pressing a lever. There is no reason to think that number is a complex parameter of the external world, one that is more abstract than other so-called objective or physical parameters such as color, position in space, or temporal duration. In fact, provided that an animal is equipped with the appropriate cerebral modules, computing the approximate number of objects in a set is probably no more difficult than perceiving their colors or their positions.
Indeed, we now know that rats and many other species spontaneously pay attention to numerical quantities of all kinds--actions, sounds, light flashes, food morsels. For instance researchers have proved that raccoons, when presented with several transparent boxes with grapes inside, can learn to systematically select those that contain three grapes and to neglect those that contain two or four. Likewise, rats have been conditioned to systematically take the fourth tunnel on the left in a maze, regardless of the spacing between consecutive tunnels. Other researchers have taught birds to pick the fifth seed that they find when visiting several interconnected cages. And pigeons can, under some circumstances, estimate the number of times they have pecked at a target and can discriminate, for instance, between forty-five and fifty pecks. As a final example, several animals, including rats, appear to remember the number of rewards and punishments that they have received in a given situation. An elegant experiment by E. J. Capaldi and Daniel Miller at Purdue University has even shown that when rats receive food rewards of two different kinds--say, raisins and cereals--they keep in mind three pieces of information at the same time: the number of raisins that they have eaten, the number of pieces of cereals, and the total number of food items. In brief, far from being an exceptional ability, arithmetic is quite common in the animal world. The advantages that it confers for survival are obvious. The rat that remembers that its hideout is the fourth to the left will move faster in the dark maze of tunnels that it calls home. The squirrel that notices that a branch bears two nuts, and neglects it for another one that bears three, will have more chances of making it safely through the winter.
How Abstract Are Animal Calculations?
When a rat presses a lever twice, hears two sounds, and eats two seeds, does it recognize that these events are all instances of the number "2"? Or can't it see the link between numbers that are perceived through different sensory modalities? The ability to generalize across different modalities of perception or action is an important component of what we call the number concept. Let us suppose, as an admittedly extreme case, that a child systematically utters the word "four" whenever he or she sees four objects, but randomly picks the words "three," "four," or "nine" when he or she hears four sounds or makes four jumps. Although performance is no doubt excellent with visual stimuli, we would be reluctant to grant the child knowledge of the concept of "4", because we consider possession of this concept to entail being able to apply it to many different multimodal situations. As a matter of fact, as soon as children have learned a number word, they can immediately use it to count their toy cars, the meows of their cat, or the misdemeanors of their little brother. What about rats? Is their numerical competence confined to certain sensory modalities, or is it abstract?
Unfortunately, any answer must remain tentative because few successful experiments have been done on multimodal generalization in animals. However, Russell Church and Warren Meck have shown that rats represent number as an abstract parameter that is not tied to a specific sensory modality, be it auditory or visual. They again placed rats in a cage with two levers, but this time stimulated them with visual as well as with auditory sequences. Initially, the rats were conditioned to press the left lever when they heard two tones, and the right lever when they heard four tones. Separately, they were also taught to associate two light flashes with the left lever, and four light flashes with the right lever. The issue was, how were these two learning experiences coded in the rat brain? Were they stored as two unrelated pieces of knowledge? Or had the rats learned an abstract rule such as "2 is left, and 4 is right"? To find out, the two researchers presented mixtures of sounds and light flashes on some trials. They were amazed to observe that when they presented a single tone synchronized with a flash, a total of two events, the rats immediately pressed the left lever. Conversely, when they presented a sequence of two tones synchronized with two light flashes, for a total of four events, the rats systematically pressed the right lever. The animals generalized their knowledge to an entirely novel situation. Their concepts of the numbers "2" and "4" were not linked to a low level of visual or auditory perception.
Consider how peculiar the rats' behavior was on trials with two tones synchronized with two light flashes. Remember that in the course of their training, the rats were always rewarded for pressing the left lever after hearing two tones, and likewise after seeing two flashes of light. Thus, both the auditory "two tones" stimulus and the visual "two flashes" stimulus were associated with pressing the left lever. Nevertheless, when these two stimuli were presented together, the rats pressed the lever that had been associated with the number 4! To better grasp the significance of this finding, compare it with a putative experiment in which rats are trained to press the left lever whenever they see a square (as opposed to a circle), and to respond left whenever they see the color red (as opposed to green). If the rats were presented with a red square--the combination of both stimuli--I bet that they would press even more decidedly on the left lever. Why are the numbers of tones and flashes grasped differently from shapes and colors? The experiment demonstrates that rats "know," to some extent, that numbers do not add up in the same way as shapes and colors. A square plus the color red makes a red square, but two tones plus two flashes do not evoke an even greater sensation of twoness. Rather, 2 plus 2 makes 4, and the rat brain seems to appreciate this fundamental law of arithmetic.
Perhaps the best example of abstract addition abilities in an animal comes from work done by Guy Woodruff and David Premack at the University of Pennsylvania. They set out to prove that a chimpanzee could do arithmetic with simple fractions. In their first experiment, the chimpanzee's task was simple: It was rewarded for selecting, among two objects, the one that was physically identical to a third one. For instance, when presented with a glass half-filled with a blue liquid, the animal had to point toward the identical glass when presented next to another glass that was filled up to three-quarters of its volume. The chimp immediately mastered this simple physical matching task. Then the decision was progressively made more abstract. The chimp might be shown a half-full glass again, but now the options were either half an apple or three-quarters of an apple. Physically speaking, both alternatives differed widely from the sample stimulus; yet the chimpanzee consistently selected the half apple, apparently basing its responses on the conceptual similarity between half a glass and half an apple. Fractions of one-quarter, one-half, and three-quarters were tested with similar success: The animal knew that one-quarter of a pie is to a whole pie as one-quarter of a glass of milk is to a full glass of milk.
In their last experiment, Woodruff and Premack showed that chimpanzees could even mentally combine two such fractions: When the sample stimulus was made of one-quarter apple and one-half glass, and the choice was between one full disc or three-quarters disc, the animals chose the latter more often than chance alone would predict. They were obviously performing an internal computation not unlike the addition of two fractions 1/4 + 1/2 = 3/4. Presumably, they did not use sophisticated symbolic calculation algorithms as we would. But they clearly had an intuitive grasp of how these proportions should combine.
A final anecdote concerning Woodruff and Premack's work Though the manuscript reporting their work was initially titled "Primitive mathematical concepts in the chimpanzee: proportionality and numerosity," an editorial error made it appear in the pages of the scientific journal Nature under the heading "Primative mathematical concepts ... "! Involuntary as it was, this alteration was not so improper. For primitive, indeed, the animal's ability was not. And if "primative" was taken to mean "specific to primates," then the neologism seemed very appropriate here because such an abstract ability to add fractions has not been observed in any other species so far.
Table of Contents
Preface to the Revised and Expanded EditionPreface to the First Edition
Introduction
Part I: Our Numerical Heritage
Chapter 1: Talented and Gifted Animals
Chapter 2: Babies Who Count
Chapter 3: The Adult Number Line
Part II: Beyond Approximation
Chapter 4: The Language of Numbers
Chapter 5: Small Heads for Big Calculations
Chapter 6: Geniuses and Prodigies
Part III: Of Neurons and Numbers
Chapter 7: Losing Number Sense
Chapter 8: The Computing Brain
Chapter 9: What Is a Number?
Epilogue. The Contemporary Science of Number and Brain
Appendix
Notes and References
Bibliography
Main books consulted
Useful web resources
Detailed bibliography
Index