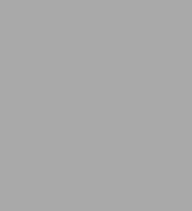
The Moment of Creation: Big Bang Physics from Before the First Millisecond to the Present Universe
240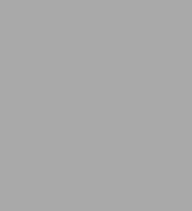
The Moment of Creation: Big Bang Physics from Before the First Millisecond to the Present Universe
240eBook
Available on Compatible NOOK devices, the free NOOK App and in My Digital Library.
Related collections and offers
Overview
"Fascinating reading. A mind-stretching book." — John Barkham Reviews
One of the founders of modern quark theory traverses the eons to bring readers within the first millisecond of the Big Bang. Trefil employs minimal mathematics in this compelling and lucid narrative, which not only offers a remarkable view of the universe's beginnings, but also speculates about its end. Compelling and lucid, this reader-friendly narrative travels billions of years back in time to depict the events that culminated in the Big Bang: the colossal explosion that initiated the existence of the universe. James Trefil is one of the founders of modern quark theory, and he specializes in explaining complex scientific matters to nonspecialists. 1983 edition.
Product Details
ISBN-13: | 9780486152189 |
---|---|
Publisher: | Dover Publications |
Publication date: | 03/27/2013 |
Sold by: | Barnes & Noble |
Format: | eBook |
Pages: | 240 |
File size: | 7 MB |
Read an Excerpt
The Moment of Creation
Big Bang Physics from Before the First Millisecond to the Present Universe
By James S. Trefil, Gloria Walters
Dover Publications, Inc.
Copyright © 1983 James S. TrefilAll rights reserved.
ISBN: 978-0-486-15218-9
CHAPTER 1
The Big Bang
One thing about the past. It's likely to last. OGDEN NASH
It took us several thousand years to discover that the solid earth on which we live is not the center of the universe, the unmoved core around which the rest of the heavens revolve. But after Copernicus and Kepler taught us that the earth moved and the sun stood still, it did not take us long to realize that the stars were bodies much like the sun. The fact that they appeared fixed in the heavens was taken (rightly) to indicate that they lie very far from our solar system. By 1750, only a little more than a century after Galileo had been forced to recant the Copernican doctrine at his trial for heresy in 1633, the English astronomer Thomas Wright was putting forward the idea that the appearance of the Milky Way was evidence that the stars near the solar system are arranged in a flat, disklike structure. We now call this structure the Milky Way galaxy (galacticos actually means "milky" in Greek). It is a collection of some 10 billion stars, of which our own sun is a rather ordinary member. How many other planetary systems there may be remains unknown.
If the sun is part of a collection of stars arranged in a certain way, it is natural to ask whether other such collections exist in the universe. Debate on this question went on throughout the late eighteenth and nineteenth centuries. It centered on faint patches of light in the sky known as nebulae, of which there are many. One, in the constellation Andromeda, had been known to Arab and Persian astronomers since the tenth century. The famous astronomer Sir William Herschel suggested that some of these nebulae might be galaxies like the Milky Way, as did Immanuel Kant. Yet there are many nebulae in the sky that are not galaxies. There are, for example, clouds of gas illuminated by stars in their interior, presenting the same appearance as galaxies. Such nebulae are clearly in our own galaxy, and strong arguments were made against assigning huge distances to any other nebulae in the sky. One argument centered on the fact that flashes of light were occasionally seen originating in some nebulae—flashes of such brightness that no energy source known to nineteenth-century astronomers could have produced them unless the distance to the origin of the flash was relatively small. Today we know that these flashes were supernovae and that the source of energy is the conversion of huge amounts of matter into energy. In the nineteenth century, however, supernovae were not understood and the conversion of mass to energy was unknown, so the question of whether some nebulae were indeed distant galaxies or merely clouds within the Milky Way remained very much open.
It was not until 1923, after the 100-inch telescope at the Mount Wilson Observatory in the mountains above Los Angeles was completed, that Edwin Hubble was able to resolve this puzzle. Because of the power of the new instrument, it was possible to pick out individual stars in the Andromeda nebula. The types of stars Hubble saw were similar to those in our own galaxy; in particular, he was able to isolate several stars of the type known as Cepheid variables. These are stars whose brightness varies periodically on a time scale of weeks or months, and it was known that the period of the variation was related to the total amount of light emitted by the star. This fact can be used to measure distances in our own galaxy, because if we know how much light a star is emitting and how much we actually receive on the earth, some simple arithmetic will tell us how far away the star must be. Using this well-known technique on the stars observed in the Andromeda nebula, Hubble was able to show that the stars (and hence the nebula) must be almost a million light-years away. Since the Milky Way is only 100,000 light-years across, the only possible conclusion from Hubble's work was that the Milky Way must be only one of many galaxies in the universe. Since Hubble's time, the number of known galaxies has passed the million mark, and the growth of this number shows no sign of slowing down.
Just as those who followed Copernicus had to surrender the concept of the earth and the solar system as the center of the universe, scientists in the twentieth century have had to abandon the concept of the uniqueness of the Milky Way. It turns out that we live in a rather typical galaxy, with nothing to distinguish it from others except for the fact that it is our home.
The Universal Expansion
Once the existence of other galaxies was firmly established, Hubble's continued studies revealed another important (and, at the time, surprising) thing. It appeared that the distance between the Milky Way and other galaxies was increasing—that other galaxies were moving away from us. Furthermore, it appeared that the farther away from us the galaxy in question was, the faster was the speed of its recession. So crucial is this observation to the development of modern cosmology that it is worth spending some time understanding how Hubble arrived at this result.
Anyone who has stood next to a railroad track and listened to the whistle of a passing train knows that the pitch changes as the train approaches and then passes into the distance. As the train approaches us, the pitch of the whistle grows higher, and as the train moves away, the pitch drops. This is a specific example of a rather general phenomenon associated with waves of all kinds, a phenomenon known as the Doppler effect. Figures 1 and 2 can help us to understand the effect.
The source of the sound wave (the train whistle in our example) creates a repeating pattern of pressure in the air. When these pressure patterns reach the ear, they vibrate the eardrum and we hear a sound. The more high-pressure ridges that strike the ear each second, the faster the eardrum vibrates and the higher the pitch of the sound we perceive. If the source is stationary, as in Figure 1, then the waves move out in uniformly spaced concentric spheres. Any observer will hear the same pitch, no matter where he stands.
If, on the other hand, the source of sound is moving (as with our train), then the situation is different. Each wave will go out as a sphere, but that sphere will be centered on the position of the source at the time the wave was emitted. This means that the spheres will no longer be concentric but will bunch up, as shown in Figure 2. An observer standing in front of the source at A will find the crests of the wave more closely spaced than normal, and he will hear a higher-pitched sound. Conversely, someone standing in back of the source at B will find the crests more widely separated and will hear a lower sound. This explains the train whistle phenomenon.
This effect was first suggested by mathematician Christian Johann Doppler at Prague in 1842 and was verified experimentally three years later by the Dutch scientist Christoph Buys Ballot. His experiment was as direct as it was picturesque. He assembled a group of musicians who had perfect pitch, and had a group of trumpeters pass by on an open railway car. Compared to this technique, our modern testing methods that employ sensitive microphones and oscilloscopes seem hopelessly prosaic.
Light and sound are both wave phenomena, and it is clear that Doppler's argument will apply to both, even though the nature of the waves is quite different in the two cases. What this means is that if we look at light emitted by a particular atom in a distant galaxy and then look at light emitted by that same species of atom in our laboratory, a comparison of the perceived wavelengths will tell us whether that galaxy is moving toward us or away from us. In the case of light, the bunching up of the wave crests seen at point A in Figure 2 will be perceived as a shift toward the blue, or high-frequency, end of the spectrum, while the spreading out at point B will be perceived as a shift toward the red, or low-frequency, end.
What Hubble found was that every galaxy whose distance he could determine with his instruments showed a red shift, indicating that every galaxy is moving away from us. Furthermore, Hubble concluded from his data that the farther away the galaxy was (with distance measured by the Cepheid variable data), the higher was its red shift.
The picture that emerged from Hubble's work, therefore, was of a universal expansion of the universe, with all galaxies receding from each other. Knowing the separation of the galaxies at the present time and the speed at which they are receding, it is a simple exercise to imagine "running the film in reverse" and letting the galaxies get closer and closer together. In principle, then, we could infer from the present condition of the galaxies the amount of time that has elapsed since all the matter in the universe existed in a highly compact-state. The number we get in this way is called the Hubble time or the Hubble age and represents a rough guess at the age of the universe. This particular number has a long and somewhat checkered history.
Measuring the Hubble age requires that we have a very good understanding of the Cepheid variable stars and that we have very accurate measurements of the red shift of other galaxies. By the nature of things, those galaxies that are farthest away will have the largest red shift, and it is for these galaxies that we will expect the most accurate measurements of the Doppler effect. On the other hand, it is precisely for these galaxies that the Cepheid variables will be hardest to distinguish. The inverse situation holds for nearby galaxies. In other words, it is not a trivial matter to measure both the red shift and the distance with sufficient accuracy to make a precise determination of the Hubble age. When Hubble made the first measurement in 1931, he set the age of the universe at only a billion years or so—about ten times smaller than the presently accepted number. The problem with Hubble's billion years was that the age of the earth as determined by geologists was thought to be several billion years, and the earth could hardly be older than the universe. Improved techniques and a recalibration of the Cepheid-variable scale have led to repeated changes in the best estimate of the Hubble age, and even today astronomers can make headlines by announcing that the universe is only half as old (or twice as old) as had been previously thought. Creationists, as might be expected, enjoy pouncing on such statements as evidence against the entire evolutionary picture of the universe. A much more reasonable attitude is that of one astronomer whose judgment I have learned to trust: "The Hubble age is 15 billion years, plus or minus 50 percent." In other words, the best guess for the Hubble age is 15 billion years, a number we will adopt for the rest of this book. We cannot, however, rule out ages as low as 7 billion or as high as 20 billion years. Since this uncertainty is not terribly important to most modern cosmology, a better determination of the Hubble age is not regarded as an important goal in astronomy.
The only force that now acts on an outward-moving galaxy is the gravitational attraction of the rest of the galaxies. We know enough about the way matter behaves under the influence of gravity to be able to trace the expansion backward in time. We cannot believe, however, that we can use this sort of simple extrapolation all the way back to the Big Bang. At the early stages of the expansion, the density of matter would have been high enough so that electrical and even nuclear forces could be expected to play a role in the development of the universe. This is not a trivial statement, since our theories tell us that the present uniform expansion need not be typical of earlier epochs.
The Big Bang
Despite these uncertainties in the exact age of the universe, it has become accepted that the expansion that we see now began about 15 billion years ago, from a situation in which all matter was in a highly condensed state. The term Big Bang is applied to this event, and the picture this term conveys of a universe consisting of fragments hurled out from an exploding center is essentially accurate. The only problem with this simple view of things is that it seems to imply that the Big Bang was analogous to the explosion of an artillery shell, with fragments flying out through space. Actually, modern cosmologists have a much more complex view of the process.
The theory of relativity teaches us that there is a delicate interconnection between matter and space. If we think of free space as a kind of grid marked out in the void, then matter has the effect of distorting the grid. In this view, the expansion of matter that we call the Big Bang would carry the grid with it, stretching it out as if it were a rubber sheet. A way of visualizing the expansion as it is seen by a modern cosmologist is to think of blowing up a balloon that has dots scattered over its surface, with each dot representing a galaxy. To someone on one of the dots, it is not so much a question of the balloon expanding through space as it is an expansion of space itself (as represented by the balloon's surface).
There is another important point about the universal expansion that can be understood in terms of the balloon. If you stood on one dot and looked around you, it would appear to you as if you were standing still and every other dot were receding from you. In other words, it would appear to you as if you were the center of the universal expansion, occupying a privileged place in the universe. This would not be true, however, because it is clear that an observer on any other dot would see exactly the same thing. Everyone sees himself as being stationary and everyone else receding, so no observer's view of the universe is any more privileged than that of anyone else.
This is a special case of the principle of relativity. In its most general form, this principle states that the laws of nature must be such that every observer will find them to be identical—that there is no privileged frame from which to observe the universe. The principle, so compatible with the working of the twentieth-century mind, is built into contemporary physics from the foundation up. In a sense, it plays a role in modern science similar to the geocentrism of ancient and medieval sciences. We build it into our perceptions of the universe because we feel it ought to be true, and, as yet, we have found no experimental evidence to convince us that it is not. But then, geocentrism worked beautifully for several millennia, too.
Echoes
Although the idea that the universe may have originated in a cataclysmic explosion billions of years ago is implicit in Hubble's discoveries, these discoveries do not force us to accept the Big Bang. Cosmologists are marvelously imaginative people, able to produce alternative explanations for any fact at the drop of a hat. For a long time, the chief rival to the Big Bang picture was a theory in which, as the galaxies receded from each other, new matter was created in the voids, creating new galaxies that would, in turn, recede and be replaced by still newer ones. The simple existence of the red shift data in and of themselves could not distinguish between the Big Bang and this alternative. Before the Big Bang could be accepted, some more direct confirmation would have to be found.
We can understand what this direct evidence turned out to be if we concentrate on the fact that in its earlier stages of development the universe must have been very hot. Physicists know that any object whose temperature exceeds absolute zero radiates energy and that the type of energy radiated depends on the object's temperature. Anyone who has watched the heating element on an electric stove turn from dull red to bright orange has firsthand experience to corroborate this statement. The element is giving off that particular type of radiation we call visible light, radiation to which our eyes are sensitive and which can therefore be seen directly.
Even after the heating element has been turned off and its color has returned to normal, it is still radiating. You can verify this by putting your hand near the stove. You will feel heat coming out, even though there is no visible radiation. What has happened is that as the temperature of the heating element has dropped, the radiation given off has moved out of the visible range and into the infrared. Our eyes are not sensitive to this radiation (although it can readily be detected by instruments and special photographic film).
Nevertheless, we can perceive the radiation with our hand. The colder the object being observed, the farther away from being visible is the radiation, and the more elaborate will our detector have to be for us to sense it.
The universe was very hot a long time ago, and it has been expanding and cooling off ever since. Like the heating element on the stove, the radiation given off during this cooling process has shifted from very energetic to less energetic and has long since passed out of the range associated with visible light. In fact, as long ago as 1948, theoretical physicists, taking into account the rate of expansion of the universe, had predicted that 15 billion years after the Big Bang the universe should have cooled off to a temperature just 3° above absolute zero, a number usually referred to as 3° on the Kelvin scale, or 3°K. Even at such an extremely low temperature, the laws of physics tell us that a body gives off radiation. Furthermore, they tell us that the radiation will be in the microwave range. Microwave radiation is intermediate between radio waves and infrared radiation in energy; it is the same radiation that cooks food in a microwave oven and is emitted by aircraft radar systems. This radiation should be bathing the entire volume of the universe by now, and we should see it coming to the earth, no matter in which direction we look. In this respect, we are in a position analogous to an observer surrounded by a fire: he feels heat coming toward him from every direction.
(Continues...)
Excerpted from The Moment of Creation by James S. Trefil, Gloria Walters. Copyright © 1983 James S. Trefil. Excerpted by permission of Dover Publications, Inc..
All rights reserved. No part of this excerpt may be reproduced or reprinted without permission in writing from the publisher.
Excerpts are provided by Dial-A-Book Inc. solely for the personal use of visitors to this web site.
Table of Contents
Contents
Title Page,Copyright Page,
Dedication,
Introduction,
PART ONE,
Chapter 1 - The Big Bang,
Chapter 2 - The Ages of the Universe,
Chapter 3 - Problems with the Big Bang Theory,
PART TWO,
Chapter 4 - Elementary Particles and Quarks,
Chapter 5 - The Fundamental Forces,
Chapter 6 - The First Modern Unification,
Chapter 7 - The Grand Unification,
Chapter 8 - The Decay of the Proton,
PART THREE,
Chapter 9 - The Moment of Creation,
Chapter 10 - Inflation,
Chapter 11 - Solving the Problems of the Big Bang,
Chapter 12 - The Search for Relic Particles,
Chapter 13 - Beyond the Planck Time: Asking the Ultimate Questions,
Chapter 14 - The Fate of the Universe,
Epilogue,
Glossary,
Index,