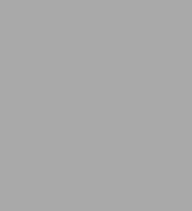
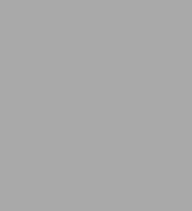
eBook
Available on Compatible NOOK devices, the free NOOK App and in My Digital Library.
Related collections and offers
Overview
Soil contamination...public lands...surface and groundwater pollution...coastal erosion...global warming. Have we reached the limits of this planet's ability to provide for us? If so, what can we do about it?
These vital questions are addressed by Jill Schneiderman in The Earth Around Us, a unique collection of thirty-one essays by a diverse array of today's foremost scientist-writers. Sharing an ability to communicate science in a clear and engaging fashion, the contributors explore Earth's history and processes--especially in relation to today's environmental issues--and show how we, as members of a global community, can help maintain a livable planet. The narratives in this collection are organized into seven parts that describe:
- Earth's time and history and the place of people in it
- Views of nature and the ethics behind our conduct on Earth
- Resources for the twenty-first century, such as public lands, healthy forests and soils, clean ground and surface waters, and fluctuating coastlines
- Ill-informed local manipulations of landscapes across the United States
- Innovative solutions to environmental problems that arise from knowledge of the interactions between living things and the Earth's air, water, and soil
- Natural and human-induced global scale perturbations to the earth system
- Our responsibility to people and all other organisms that live on Earth
Never before has such a widely experienced group of prominent earth scientists been brought together to help readers understand how earth systems function to produce our physical and biological environment. Driven by the belief that earth science is, and should be, an integral part of everyday life, The Earth Around Us empowers all of us to play a more educated and active part in the search for a sustainable future for people and other living things on our planet.
Product Details
ISBN-13: | 9781466814431 |
---|---|
Publisher: | Holt, Henry & Company, Inc. |
Publication date: | 03/27/2000 |
Sold by: | Macmillan |
Format: | eBook |
Pages: | 250 |
File size: | 3 MB |
About the Author
Jill S. Schneiderman is an associate professor of geology at Vassar Collge, where she teaches courses in geology, environmental studies, and women's studies. She is the author of numerous scientific papers on subjects ranging from the formation of mountain ranges to the geological history of the Nile Delta. A congressional science fellow during the 104th Congress, she worked on environmental issues as a science advisor to the Democratic leader of the U.S. Senate.
Read an Excerpt
Earth Around Us, The
Maintaining A Livable Planet
By Jill Schneiderman
Henry Holt and Co.
Copyright © 2000 Jill SchneidermanAll rights reserved.
ISBN: 978-1-4668-1443-1
The Earth Around Us
Part IRECORDS OF TIME AND HISTORYLife must be lived amidst that which was made before. Every landscape is an accumulation. The past endures.--Donald W. Meinig
The Earth has a vast history, of which humans are a part. Traces of events that characterize Earth's history lurk amidst landscapes--they convey a context for human existence, they mark our absence or presence. Astute observers can read the record of past events but also must proceed with caution, for human historical context affects human perceptions. The essays that follow build one on the other to give the reader a sense of Earth's time and history as well as the place of people in it.In the first essay, Susan Kieffer reflects on the rare and the routine among geological events--meteorite impacts, floods, volcanic eruptions, and earthquakes--that affect individuals and civilizations. Then, John McPhee unearths the "abyss of time" in the intellectual rovings of eighteenth- and nineteenth-century naturalists. Lauret Savoy explores the effects of human perceptions and actions on North American terranes from the desert Southwest to midcontinent prairies and grasslands. And finally, Paul Bierman paints a history of natural and human-induced change on a Vermont landscape.In the aggregate, these essays reveal the simultaneously fleeting yet substantial nature of human participation in Earth's history.1Geology: The Bifocal ScienceSusan Werner Kieffer
Rare events seem to be fodder for Hollywood megamovies such as Volcano, Twister, Dante's Peak, Deep Impact, and Armageddon. However, consideration of the possibility and consequences of such events occupies almost no time in our personal thinking, nor much of a fraction of most community budgets. Major infrastructure sectors, such as power generation and waste disposal facilities, also fail to have resources set aside for such events. But the occurrence of even one rare event can have catastrophic consequences for the planet, for living species, for economies, and for technology.Bifocal lenses allow people with vision problems to see both near and far objects. In geology, a view of rare events far away in space and time gives us the ability and perspective to focus on their possible occurrence and impact in our nearby space and in current and future times. We will use the bifocal capacity of geology to look into the distant past and deep Earth to focus on rare, but possible, events that could affect human civilization. As we go through examples of such events, we should keep asking: How can we bring rare, nonintuitive events into our collective consciousness and into our planning for future generations? Even in cases where we have 7,000 years of human records--such as the history of the Nile River system--supplemented by a body of geological knowledge that spans an even longer time, do we dare think that we know all the possible behaviors of a geological system or the consequences of our perturbations on it? The bifocal view of geology clearly tells us not to be so arrogant.WHAT IS RARE?The word rare can be used in the context of space ("spatially rare") or time ("temporally rare"). In the context of space, rare means "thinly distributed over an area," or "widely spaced," often with the implication of having unusual valuebecause of the uncommonness. In the context of time, rare means "occurring infrequently," or "uncommon." Both space and time definitions have implications that depend on the perception of the person describing an event and on the circumstances of lives, economies, cultures, and communication. We also find that there is an interconnectedness between events that are rare in space and those that are rare in time, as I will show in a number of examples.Consider first the nature of rareness in space. People who have few opportunities to travel will discover with delight new geography and will tend to think that some new sight is rare because it has seldom, or never, occurred in their own experiences. For example, when I moved from Pennsylvania to the Rocky Mountains and then to the Southwest, I was totally unprepared for the high rugged mountains or for the beautiful cacti that I saw. I was overwhelmed by these "rare" mountains and flora, for I had no context for judging the commonness or rareness of what I was seeing. After living in the Southwest for many years, cacti were common and hardwood forests, wetlands, or coastal estuaries seemed rare.A world traveler will have a perspective on the rareness of various spatial features on the Earth different from that of a local dweller. The traveler will be inclined to say, "Oh, this feature in Asia reminds me of that one in the United States," or "This is amazingly like that." Thus, when the world traveler says that something in our natural world is rare, the meaning can be quite different from that of exactly the same words spoken by the local traveler.Geologists tend to be world travelers because of the nature of our science. Thus, our perspective on spatially rare features should carry different weight than the perspective of someone who has not traveled so widely. For example, the travels of geologists tend to make them deeply and personally aware of the mushrooming population of the planet--every major city that we visit is growing faster than its infrastructure. Television may carry this message into individual homes, but people who do not travel and personally experience the population changes may think that the situations portrayed are rare. The world traveler, however, will argue that the city-population-infrastructure problem is common, and may even go further and advocate that the problem is so common that it represents a crisis to be solved.The same comparison holds with respect to time. A young person will relish new experiences as unique and rare. An elder, however, may appear jaded, having lived through many of those new experiences several times. It takes something truly unique to surprise an elder.How do these perspectives on space and time extend to geology? Geologists are both the world travelers and the elders of the planet. We see the planet in three spatial dimensions and also across the fourth dimension of time. Just as the person who looks through bifocal lenses can see both far and near objects, our science allows us to look at the evolution of our planet at many scales of space and throughout all of geologic and astronomical time. We can think of geology as looking at the world through both a magnifying glass and amicroscope. Because of the nature of our science, we can define the terms common and rare on many different scales of space and time.For example, what is common and what is rare on the time scale of a billion years, which is the relevant scale for the formation of planets? What is common and what is rare on the time scale of a million years, which is the time scale for the formation of many of the fuel resources that we humans care about? What is common and what is rare on the time scale of a thousand years, which is the time scale we consider when dealing with natural hazards to human civilization and culture? What is common and what is rare on the time scale of a century, which represents three human generations, parent, child, and grandchild? What is common and what is rare on the time scale of a few decades, the life of an individual? What is common and what is rare on the time scale of a few weeks or years--the time scale of teenagers?On the time scale of a billion years, formation of stars and galaxies is common. However, according to our knowledge at this time, formation of whole universes is rare. Currently, we also think that the formation of planets and other life forms is rare, but our data on this subject are changing rapidly because of improvements in observational techniques. Spatially, these phenomena may also be rare because of the special circumstances required for their formation. Thus, rarity is interconnected spatially and temporally in these processes.On the time scale of a million years, formation of sedimentary, igneous, and metamorphic rocks is common. Formation of oil, gas, and coal deposits may be rare on this time scale because of particular requirements of large deposits of organic matter combined with highly specific pressure and temperature conditions. Formation of precious metal deposits is probably a common and ongoing process at this time scale, but these deposits are spatially rare.On the time scale of thousands of years, small volcanic eruptions may be common, but big events that destroy a whole volcanic mountain may be rare because a long time is required to replenish the heat and mass that cause such an event. Likewise, small and medium earthquakes may be common, but large ones that destroy whole cities, nations, or civilizations may be rare because of the long time needed to accumulate strain that is released by the big earthquakes. Spatially, earthquakes and volcanic eruptions are restricted to special parts of the planet because of processes now well explained by the unifying theory of plate tectonics, and their commonness or rarity is determined by the circumstances of supply of strain or of energy and heat at specific locations.On the time scale of centuries, shooting stars are common, but big meteorite impacts are rare. And, whereas the effects of a big meteorite impact may be global, the craters formed even by large impacts are small compared with the scale of the Earth.On the time scale of weeks and years, small or medium changes in river flow may be common, but floods that drain an entire watershed during an exceptionally strong El Niño or La Niña event may be rare.Clearly, conclusions like these depend on our state of knowledge. Not only scientific techniques but also time, communication, and culture play roles in how we perceive "rarity."In the time domain, the nature of human experience is to consider as common, not rare, those events that occur in one's lifetime. Beyond this, we have the capacity to absorb anecdotal knowledge from our parents, our grandparents, and perhaps our great-grandparents. But, in societies with written rather than strictly oral traditions, knowledge from more remote times becomes intellectual rather than intuitive.Assuming that the lives of an individual, her parents, and grandparents span a century, events that occur once per century seem to be common because of the family memories. Events that occur on certain time scales are given the names that reflect their statistical likelihoods: "the annual flood," "the hundred-year drought," or "the thousand-year forest fire." Up to intervals of about a century, these events are part of familiar, intuitive experience. In many places, government plans for zoning and response to hazards exist for these time scales. Nevertheless, financial and political factors often result in poor plans. Every year, floods devastate homes built on well-known floodplains, hurricanes destroy fragile houses in areas that are known to experience frequent hurricanes, and waves destroy structures improperly constructed on coastal beaches. These losses arise despite our certain knowledge that these events will occur. The "annual spring flood" may be small for a few years, or big for a few years, but it will occur in the spring. The more serious problem is that there will be larger spring floods over time scales of centuries or millennia, and these rare events are neither intuitive nor easily predicted.Although rare events are rare, they do happen. Our lives are short; the term of influence of elected politicians is even shorter. Nevertheless, one or more of those "rare" events may happen during our lives, or during some politician's "watch." Obviously, individuals and communities might benefit from a satisfactory, and practical, definition of rare. So might groups charged with protecting the public, for these agencies need to be able to predict and to define the hazards and their consequences. Scientific research and monitoring groups, such as the U.S. Geological Survey and the National Center for Atmospheric Research, study and try to understand these events. Even nongeological agencies, such as the Centers for Disease Control in the United States, will benefit from geological evidence about rare events, such as climate change, because of the response of living organisms to these changes. Service agencies, such as the Red Cross and the Federal Emergency Management Agency, that respond to crises brought on by natural phenomena need to know what to expect from and how to respond to crises. Likewise, insurance companies care about a suitable definition of rare because they mediate the cost between insurance premiums and payouts.With this introduction and perspective, let's focus on the concept of "rare" at the time scale of human civilization--thousands to tens of thousands ofyears. What is common and what is rare? Four examples, viewed at different scales of space and time, bring into focus the issue of rarity over thousands to tens of thousands of years and its relevance to our present and future periods. As we consider these scenarios, we should ask: How can we bring nonintuitive events into people's individual and collective consciousness?Meteorite ImpactsMost humans probably see a few "shooting stars" in a lifetime. The observation may be in the context of a romantic tryst in the mountains, a small observatory built by a friend or institution, or a nightly newscast. We assimilate shooting stars as part of our individual human experience, in the sense that we "expect" to see them. Therefore, although not common, shooting stars are not rare to most of us.However, consider the few percent of the human population that do not see shooting stars--perhaps because of perennially bad weather or because of city light pollution. To people living under these conditions, shooting stars are not merely uncommon, they are "rare" or even "unknown." The little and common events do not reside in the individual's consciousness, so there is no basis for extrapolating them into the larger collective consciousness. Superstitions may be rooted in such a condition, that is, a lack of knowledge of common events on which to extrapolate to predictions about larger and rarer events.If we see shooting stars and understand what causes them, then we have some basis for understanding that bigger shooting stars may exist. With scientific measurements, we find that the shooting stars are caused by meteorites that are the size of a sand grain or a small pea and enter the Earth's atmosphere. We might wonder what would happen if they did not burn up in the atmosphere but landed on Earth, which they would do if they were bigger. In fact, some meteorites do land here; and some of them end up in laboratories, either because some rock collector recognizes an unusual rock on the ground or because these rocks actually disrupt someone's life by falling through roofs, landing in a herd of cattle while a farmer watches, or dropping onto cars.When meteorites land on the Earth, they make depressions called impact craters. We can go into the field--geology slang for going out into the world and making measurements--and look at various young and old craters, measure their ages, make estimates of the size of the meteorite that caused a particular crater, and infer the frequency with which meteorites of various sizes hit the Earth.1 A famous small crater less than a half-mile across is Meteor Crater, Arizona, formed by the impact of an iron meteorite about 50,000 years ago. This impact probably caused local effects over northern Arizona. An important large crater about 62 miles in diameter is Chicxulub, on the Yucatan Peninsula in Mexico. This crater was formed about 65 million years ago.2 The impact at Chicxulub affected the whole planet, causing the extinction of many species, including dinosaurs. By studying the numbers, sizes, and ages of craters on theEarth and other planets, geologists in the field of planetary sciences are able to make measurements and models of how often meteoroids of different sizes impact the Earth.We also can go to observatories and measure the numbers of comets and Earth-crossing asteroids that are currently in the vicinity of our solar system.3 These measurements give us other reference points for our statistics on the frequency of impacts of various sizes.For example, several meteoroids the size of grapefruits will hit us in an average year. One meteoroid the size of half of a football field will impact every 50,000 years and make a crater about the size of Meteor Crater. A meteoroid a mile in diameter will impact us every few hundred thousand years; it will cause civilization-threatening consequences. A meteoroid with a six-mile diameter will hit us every hundred million years, making a crater the size of Chicxulub and producing global consequences.We can use these statistics to predict the probability of an impact over any given time frame--for example, we can say that the chance that you will die as a consequence of a meteoroid impact is almost the same as the chance that you will meet your end in the crash of a passenger aircraft: 1 in 20,000. However, we cannot predict exactly when or where an impact might occur until we actually see an object heading on a trajectory toward us. As more measurements on the trajectory and the size of the object are obtained, our ability to predict the consequences of an impact would improve. We would certainly experience different consequences if a crater like Meteor Crater were formed in New York City, or in the Arizona desert, or in the ocean.Weighing the probability and the possible consequences of impact is a challenge that earth and planetary scientists face. For this problem, the lenses we use are literally as large as the telescopes that aid our search for meteoroids and as small as the microscopes that allow us to examine them in detail.FloodsWhether about the Mississippi River in the United States, the Red River that crosses the border between the United States and Canada, or the Yangtze River in China, stories of flooding rivers are much in the news. Prior to the time when massive dam projects were initiated in the twentieth century, people in these regions and even desert dwellers in the southwestern United States or along the Nile in Egypt could observe seasonal fluctuations in the discharge of their nearby river. Spring floods, gully washes, flash floods, winter freezes, and ice jams were part of community knowledge.Even if you lived near a very small creek, as I did when I was a child, you saw the variations seasonally and annually. Much to my parents' annoyance, the Fisher Creek, normally a small trickle that I could easily hop over, typically overflowed its banks about two blocks upstream from our house in the spring and wound its way downstream by taking a right angle bend through ourbasement. This small creek discharged into the larger Conewango Creek. When the Conewango flooded in the spring--which, of course, it did every year--we students inevitably delighted in a few extra vacation days because the county had unwisely placed the junior high school on the floodplain. Even with a good collective conscience about the seasonal and annual behavior of this small river system, city zoning laws did not, and typically do not, prevent considerable expense and damage due to the floods. Political and economic pressures prevail over geological evidence and collective wisdom.The massive dam projects that began in the early twentieth century on nearly every river in the United States, and in many other countries, have greatly modified our individual and collective consciousness about flooding. Many people who live downstream of dams see no annual variations in water discharge because the reservoirs behind the dams buffer the seasonal, and even decade-scale, variations. These large dams have had a massive impact in places like Egypt, where a chain of dams culminating in the High Aswan Dam changed the behavior of the Nile; and in the United States, where a series of dams has altered the behavior of the Colorado River system. When the Three Gorges Dam is completed along the Yangtze River in China, there will inevitably be similar great effects. What does history tell us of the effects? And, what does history not tell us?Probably more than any other factor, the Nile was responsible for the great civilization of ancient Egypt. It's history is well documented both historically and geologically.4 The longest river on the African continent, the Nile flows north into the Mediterranean Sea. Where it meets the sea, the Nile has deposited sediment in the shape of a Greek letter delta. The beginning of Egyptian civilization coincided with the first emergence of the Nile Delta. The annual cycles of discharge of the Nile produced fertile lands along the river floodplains and on the delta. These conditions supported the great Egyptian civilization that began 7,000 to 8,000 years ago.However, natural annual fluctuations in discharge produced unpredictable flood or famine conditions, so, even during early cultural phases, humans tried to control the fluctuations. The Dynastic period, starting approximately 5,050 years before the present, began at a time when the climate in Egypt became extremely arid and the discharge on the Nile was sufficiently reduced that simple irrigation could be developed to allow year-round cultivation of crops. For 5,000 years, the Nile was slowly modified by human influence; but this modification greatly accelerated in the nineteenth century with the building of the Aswan dams--a series of increasingly high dams started in 1889 and finished when the High Dam was completed in 1970.5 Lake Nasser formed by the ponding of the Nile behind this last dam.The Aswan dams control the annual floods that used to cause damage along the floodplain, increase areas that can be cultivated for perennial crops, allow more industrialization by providing about half of Egypt's power supply, and improve navigation conditions on the Nile because they keep water levelsfairly constant. Because of the growing population of Egypt, these advantages cannot be ignored.However, serious disadvantages are also associated with the dams: The cultural disturbance to create the Aswan High Dam was large--almost 90,000 people had to be relocated and many artifacts were lost or had to be moved. The formation of Lake Nasser has induced earthquakes in a previously aseismic region.6 Seepage and evaporation cause a loss of about 15 percent of the water in the Nile system. And sediments have been filling the reservoir and decreasing its storage capacity, although the High Dam is still expected to have a life of 500 years.In turn, storage of sediments behind the dam deprives the river of those sediments downstream. So, instead of depositing sediments, the river erodes its banks. Even though the river carries this eroded sediment further downstream toward the delta, it deposits very little material on the delta relative to the amounts deposited in the past. The balance between deposition of sediments and erosion of the delta by coastal processes and subsidence has been upset, and the delta has changed from a constructive regime to a destructive one. Wetlands in the northern part of the delta are being destroyed or reduced, thereby causing ecosystems to be degraded.7Human influence is magnified by these altered conditions along the Nile. Farmers have been forced to use artificial fertilizers because natural fertilizers are no longer replenished by annual floods. Lands that are newly irrigated by the stored and diverted waters do not drain well. Minerals from the fertilizers and salt are accumulating because of evaporation, and these enter the food chain in potentially toxic levels.Waste of both nonhuman and human origins is concentrated because of the lack of drainage. The accumulation of human waste is an especially serious problem where villages are closely spaced. These conditions have led to the resurgence of diseases. For example, the parasitic disease schistosomiasis, a condition associated with snails that live in the stagnant waters of the new canal and lagoon system, is affecting a greater number of individuals than in the past. These disease threats could become a serious problem because about 95 percent of Egypt's population lives within a dozen miles of the Nile.These changes in the Nile have influenced even the large Mediterranean Sea. Loss of silt and algae from the Nile caused the disappearance of a major sardine resource from the Mediterranean, and the change in discharge has affected the Mediterranean shrimp population.The history of the modification of the Nile spans 7,000 years and gives us a perspective that we can apply to other rivers and dam projects. At the present time, the largest hydroelectric project in history is the construction of the Three Gorges Dam on the Yangtze River in China. At the time of the writing of this book, a diversion channel has already been constructed to route the Yangtze around the dam construction site. The dam is scheduled to be completed between 2009 and 2013. When filled, the lake behind the dam will floodabout 1,200 square miles. For comparison, Lake Powell behind Glen Canyon Dam covers about 250 square miles, and Lake Nasser behind the Aswan Dam covers nearly 2,000 square miles. By the time the reservoir behind the Three Gorges Dam is filled, over 1,000,000 people will have been relocated and 13 cities, 140 towns, more than 1,300 villages, 650 factories, and hundreds of the world's most ancient archeological ruins--some more than 6,000 years old--will be covered with water.Internal and international controversy over this dam is strong. The disastrous floods of 1998 in China that killed more than 2,500 people, flooded 22,000,000 acres of farmland, and destroyed nearly 3,000,000 homes did not lessen this controversy. Proponents of the dam claim that the project will stop similar flood disasters in the future. Opponents claim the opposite, largely because much of the flooding comes from rivers below the dam and because money for flood control levies on these rivers has been diverted to the Three Gorges project.The advantages and problems of the Aswan and Three Gorges dams occur in various forms with large dams all over the world. Logically and rationally, we should be able to anticipate similar effects and should not associate with them the word rare when we consider any other large dam. Do we think that, just because we have 7,000 years of records, we know all the possible consequences and there will be no "rare" events to surprise us? Geology tells us very clearly not to be so arrogant--there is much that we may not know.And so we come to the story of a wave--an unusual wave--in the Colorado River system, a waterway that originates in the Rocky Mountains.8 From nearly its source to its end, the Colorado River is dammed by the Davis, Parker, Glen Canyon, and Hoover dams (to name the most well known). Probably the most controversial among them has been the Glen Canyon Dam, located near the Arizona-Utah border. Glen Canyon Dam retains a large reservoir, Lake Powell, which releases water into the part of the Colorado River that flows through the Grand Canyon. Originally built for two main purposes--to store and distribute water to seven western states and Mexico and to generate electricity for peak power requirements on the western power grid system--it now must be managed to have minimal impact on the environment. The incompatible nature of these multiple objectives has made management of the dam very complicated. For example, the reservoir must be kept as full as possible to conserve water for distribution according to treaty agreements; yet the water must be released at rates demanded by peak power requirements in faraway cities such as Phoenix and Los Angeles. These required storage and release patterns often do help to preserve and regenerate species of aquatic flora and fauna and to replenish soil nutrients.Prior to the completion of the dam, the discharge of the Colorado River varied between about 4,000 cubic feet per second (cfs) and approximately 100,000 cfs annually, with century-scale floods known to have reached about 400,000 cfs. After the dam was completed in 1966, the discharge was controlledby the engineers and varied between about 4,000 cfs and about 30,000 cfs. Hence, the peak discharges were only about one-third the volume of typical annual floods and were much smaller than decade-, century-, or millennial-scale floods. Until 1983, Lake Powell retained all the water that would have gone into the large annual floods through the Grand Canyon because it had not filled to its operational level.The Colorado River system was essentially unexplored until 100 years ago; and even when the Glen Canyon Dam was built, relatively few people had been through the river canyons. The sport of river-running through the 200 miles of spectacular terrain in the Grand Canyon developed rapidly into a tourist industry during the years when the discharges were controlled by the dam; and probably it was enhanced by the relative predictability of the discharges. The intuitions of the river guides, their passengers, and the scientists were formed by phenomena observed at these relatively modest discharges. We knew that the famous "rapids of the Colorado" were always at locations where there were side-canyon tributaries and that floods down these tributaries episodically delivered large boulders from the tributaries into the bed of the Colorado River. Waves formed around these rocks in the riverbed at low discharges, and the rocky regions with their waves were the rapids. These waves were the major challenges, as well as part of the fun, of rafting navigation. River guides, who accumulated the most experience and knowledge of the river, observed that the waves disappeared as discharges increased because the water became deep relative to the height of the obstacles that formed the waves. The river terminology was that the waves "washed out." At the highest discharges characteristic of the operation of Glen Canyon Dam during those years, the river became fast and turbulent but relatively free of problem waves.The "rare" event occurred in 1983 about 90 miles downstream from the Glen Canyon Dam, with great human and financial cost. In 1983, just when Lake Powell was filled "to the brim," an unusually rapid snowmelt occurred in the Rocky Mountains. That no volume was left in Lake Powell to accommodate this water posed a serious danger downstream because the Glen Canyon Dam might fail to contain it. Sheets of plywood four feet by eight feet were added on top of Glen Canyon Dam to accommodate some of the extra water by raising the level of Lake Powell temporarily. Nevertheless, it also became necessary to open the emergency bypass systems and spillways. Within a period of several days, discharges below the dam increased to over 90,000 cfs.For the 20 years that Lake Powell had been filling, river guides and scientists had never seen this type of discharge. The guides assumed that they would be rafting on a turbulent, but relatively waveless, river. This assumption held true for about the first 90 miles of river below the dam, but not at a place called Crystal Rapids. Here, the waves did not wash out; instead, an unforeseen and new type of wave formed. This wave reached 20 feet in height and spanned the entire navigable part of the river. Numerous people were injured before the National Park Service was able to temporarily stop all river-running.The formation of the wave was caused by another "rare" event that had happened after completion of the dam but before any high discharges were needed. Large flash floods down the tributaries of the Colorado occur roughly once per century. And in 1968, just two years after completion of the dam, one of these century-scale floods roared down Crystal Creek, carrying boulders and debris that constricted the Colorado River to about one-quarter of its typical width. Overnight, Crystal Rapids, which had been a mere piffle not even mentioned in the exploration journals of the geologist-explorer John Wesley Powell, became one of the most formidable rapids on the Colorado.There are many tributaries draining into the Colorado; and over hundreds of thousands of years, they all have had multiple flash floods that formed debris fans.9 But over the same time scale, these debris fans have been eroded back by the large floods on the Colorado. Small annual floods have eroded the debris fans back a small distance, medium size century-scale floods have cut the fans back further, and the peak floods--perhaps every few millennia--have pushed them back even further. Typically, most debris fans are old enough to have been reworked by the largest floods typical of the Colorado, and they are eroded back so that the river keeps about half its normal width where it passes by them.Between the time of its formation in 1968 and the flood of 1983, the debris fan at Crystal Creek had never been exposed to flooding, not even an annual flood, to say nothing of a hundred-year or a thousand-year flood. So, the river was unusually constricted there. We could say that this condition was both spatially and temporally rare within this stretch of the river. A "rare" wave formed during the 1983 flood because the river was so unusually constricted by this new debris fan. As a qualitative analogy, think about the problem of running water through a hose, which normally only gets stepped on by people or dogs but suddenly gets stepped on by an elephant. At Crystal Rapids, the debris constriction was akin to the elephant problem. The water could only get through the constriction by dramatically changing its flow conditions, and a standing wave was the result of this change in conditions. (Technically, the transition was from supercritical to subcritical flow; and the far more appropriate analogy is supersonic to subsonic deceleration of an aircraft, rather than the elephant on a hose.) This wave was not the typical wave formed by a single rock or a large submerged obstacle; it was a wave formed by the overall geometric shape of the river at that location. That single flood of 1983 eroded back the Crystal Creek debris fan considerably, but the river is still more constricted there than at most other debris fans, and Crystal Rapids remains a formidable boating obstacle to river rafters because of this constriction. It will never become a "normal" river rapid as long as Glen Canyon Dam operates at its current capacity because it will never experience a peak flood.The rafting community, engineers who managed the dam, administrators of the Grand Canyon National Park, and scientists had a collective view of the river that was based on a few decades of observation--extended to a centuryfor those who studied the journals of the explorers of the Colorado. However, there was no immediate understanding, prediction capability, or planned response to conditions that exceeded those which were usual for that short period of time. Now that we have seen and experienced this previously rare event, it does not seem surprising to the scientists who understand it. Thus, we can add this wave behavior to our 7,000-year-old body of knowledge about the effect of large dams on river systems.Our view of the effect of dams on natural systems is actually longer than 7,000 years because we can study the effect that natural blockages, such as landslides or lava flows, have on river systems. However, even though we humans can view all this knowledge from the distant past through our bifocal lenses, we have not brought that understanding clearly into focus on our near and current problems. It is not clear how the "memory" of rare events is or will be passed on institutionally--we typically do not plan adequately, even for more common events. In the case of the Colorado River, guides and boating companies come and go and the individual experience of 1983 will be lost, or retained only by diminished storytelling. Scientific papers are published and get lost in volumes of information. Reports are filed in institutions and disappear onto shelves. Managers and even institutional structures change. This story is repeated on other river systems. How do we preserve and expand the memory and management recommendations for these events, that is, how do we implement action based on a bifocal view?VolcanoesThe way we live with and react to volcanic eruptions also illustrates well the problem of understanding the probability and consequences of rare events. People who live near active volcanoes develop an understanding and intuition about their normal behavior. Hawaiians are used to frequent but relatively mild eruptions of Kilauea and Mauna Loa, eruptions in which one can generally walk, or at least jog, away from the slowly advancing viscous liquid magma. Yet, in 1790, there was a rare explosion and volcanic blast from Kilauea in which soldiers of Keoua crossing a desert region with their families and cattle were suffocated and burned, and Keoua capitulated to King Kamehameha. Footprints of these doomed people can still be seen in the deposits from this eruption. A smaller, but similar eruption in May 1924 killed a person at the Hawaii Volcano Observatory.10 These were rare events by Hawaiian standards.Unlike Hawaiians, people living in the Japan archipelago are used to frequent and much more violent volcanic eruptions. Their "common" eruptions resemble the Hawaiian eruptions of 1790 and 1924. Some schoolchildren routinely wear hard hats to go to and from home and school because of the rain of falling ash and rocks! Yet these daily conditions are mild compared with events that have occurred in the past few thousand years. Mount Fuji, although presently quiescent, erupted 13 times between AD 781 and 1707; and the lasteruption in 1707 was violent enough to cover the area of present-day Tokyo, which is 60 miles from Fuji, with an inch of ash. On July 15, 1888, with almost no warning, Bandai-san blasted out its northern flank in a rapid series of violent explosions, sending steam and rock debris down steep slopes at about 50 miles per hour, burying seven villages, and killing 461 people.11 Twenty-two thousand years ago, an ash flow, called the Ito flow, traveled"over hill and dale" for 45 miles, climbing over topographic obstacles as high as 2,000 feet.12People in the American Northwest were used to living with their beautiful, but not particularly active volcanoes, until Mount St. Helens erupted violently on the eighteenth of May in 1980. Although that eruption was the largest in the Northwest since its settlement by Europeans, we now know that it was a very small eruption compared with those from similar sized volcanoes.13 Crater Lake, in Oregon, was formed by the decimation of Mount Mazama--a Mount Rainier-type of volcano--only 7,000 years ago, when civilization was emerging along the Nile. As recently as 800 years ago, there was still active volcanic activity under the lake. Seattle, at the base of Mount Rainier, would be devastated by such eruptions.An eruption of a very similar type of volcano at Novarupta in Alaska on June 6, 1912 spewed almost five cubic miles of magma out of the volcano and transported a total of nine to ten cubic miles of magma and rock down into a valley, forming the still fuming "Valley of Ten Thousand Smokes." This event took only 60 hours and was the largest eruption in the twentieth century. The ash deposits in the Valley of Ten Thousand Smokes--called ignimbrites--are the most extensive historical deposits of that kind, but the geological record shows that ignimbrites can cover thousands of square miles of land. Such eruptions are rare, but we certainly have active volcanoes that have the potential to produce them. Volcanologists are challenged to try to understand what the precursors to such rare and large events might be and how we should respond to them.Certainly, the eruption of Mount St. Helens elevated the collective consciousness of the residents of the Pacific Northwest to the potential for much more violent eruptions. But how deep is that awareness? Twenty-three thousand people--three-quarters of the people in the single village of Armero--were killed in one horrific night on November 13, 1985 by mud and debris flows down Nevado del Ruiz volcano in Colombia. How real does that fact seem to those of us living at the base of such volcanoes all around the Pacific Ocean? How many of us are aware that that eruption was well predicted by scientists and that the deaths resulted solely from political choices? Decisions to respond to rare events are extremely difficult: Evacuation of a population under the threat of a volcanic eruption that subsequently does not occur causes human dislocations and economic problems. Yet decisions not to evacuate under a threat that develops into an event cost lives, land, and property.These volcano stories show the connection between rarity in space and time. Volcanoes are spatially rare, occurring only in specific settings that wenow understand in terms of the framework of plate tectonics. They occur at lithospheric plate boundaries--the cracks that separate the Earth's crust into a few large pieces--where one plate of the crust descends under another plate; they occur at plate boundaries where plates are being pulled apart; and they occur within plates under certain anomalous conditions. However, they cover a relatively small portion of the Earth's surface and are therefore spatially rare. Large eruptions are rare in time because volcanoes need to recharge heat in order to melt rock and form magma that will erupt. Some volcanoes recharge and erupt in a relatively balanced way, producing the Hawaiian type of volcanism. Others store their recharging magma for a very long time and release it in one or a few huge eruptions.Our understanding of volcanic behavior has grown enormously over the past few decades for several reasons. Growing populations, and modern satellites, witness eruptions of volcanoes that were previously in uninhabited areas. Modern communication allows this information to be spread globally. Our bifocal lenses now range from billions of human eyes to the strongest of satellite lenses and these certainly help us solve the problems associated with understanding spatial rarity. The post-1960s view of the Earth in terms of plate tectonics theory gives us a framework to understand the locations as well as chemistry, volatility, and explosivity of certain types of volcanoes. And, modern sophisticated supercomputer models of volcanic processes, as well as rapidly changing ideas of how to make predictions in complex systems, allow us to enhance our understanding of physical processes and our prediction capabilities, as well as our knowledge of the limitations of those capabilities.EarthquakesEarthquakes are rare spatially but not always restricted to plate boundaries. In hindsight, I'm not surprised that the very first night I was in Pasadena, California, near the boundary between the Pacific and North American plates, I woke up, not because my alarm went off, but because it slid off the table onto the floor. However, I was quite astonished to be rocked in my office chair--near Toronto, Canada--in 1998 by an earthquake near the Ohio-Pennsylvania border--definitely not near a plate boundary.Californians are used to earthquakes of various magnitudes, and they have a good institutional memory of the 1906 San Francisco earthquake, as well as the more recent earthquakes at Loma Preta (1989), Landers (1992), and Northridge (1994). They also are alert to earthquakes of various magnitudes around the world, including secondary effects such as fires, mudslides, and tsunamis.However, do Californians have a concept of what could really happen in truly "rare" but possible events? Does knowledge of either the Armenian earthquake of December 7, 1988, in which 25,000 people were killed, or the major earthquake that struck near Izmit, Turkey on August 17, 1999 affect our individual or institutional actions, or is it simply not imaginable that such devastationis possible, even in highly engineered cities? Furthermore, geoscientists now recognize that the strain on large faults may not be released in a single earthquake alone or in a single earthquake followed by minor aftershocks; instead, release may cause a series of relatively large earthquakes as a fault is "unzipped." Events such as these are called earthquake "sequences" or "storms."Compelling evidence indicates that major civilizations have been severely affected by earthquakes and earthquake storms.14 One such civilization was that of the Aegean and eastern Mediterranean areas circa 1225-1175 BC, the end of the Late Bronze Age. Why did the Bronze Age end so abruptly--over a period of only about 50 years?Extremely complex plate tectonics characterize the eastern Mediterranean region--major fault lines have created the topography that has governed the evolution of civilizations through trade routes and earthquakes on those faults have destroyed those very same civilizations. Some earthquake sequences have occurred in this region in historical times. A sequence along the Anatolian Fault occurred during the 30-year period from 1939 to 1967 as strain that had accumulated over 200 quiet years was progressively released. A storm of more than 80 years duration was reported as having occurred along the same fault from AD 967 to 1050, during which time more than 20 earthquakes of damaging to destructive magnitude occurred.15 A 30-year period of earthquakes between AD 350 and 380, following a quiet period of about 300 years, resulted in damage to sites in Israel, Cyprus, Turkey, Crete, Corinth, Reggio Calabria, Sicily, and northern Libya.16Robert Drews, professor of history and classics at Vanderbilt University, compiled a map of 47 Aegean and eastern Mediterranean sites destroyed in a 50-year period at the end of the Late Bronze Age, including historically famous sites such as Mycennae, Tiryns, Midea, Thebes, Pylos, Kynos, Troy, and Megiddo.17 According to biblical history., much of this destruction was due to battles, but for some of these sites, there is no independent evidence of battles. When superposed on a map of earthquake intensities in this region, these sites fall along the zones of high-intensity shaking. Drews recognized the possibility of earthquakes as a cause. In fact, earthquake storms disrupted many of these cities over a prolonged, but short, period of time. The destruction of buildings and people affected trade, induced economic hardship, and weakened defenses, situations leading to attacks and uprisings. Clearly, physical events led inexorably to political, social, and economic "systems collapse" in the Aegean and eastern Mediterranean at the end of the Late Bronze Age.Over four millennia, these storms might be considered "rare." But geology's view through a bifocal lens aimed at ancient archeology suggests that such events do occur--they have occurred often enough that we should expect them in current times. In our modern interconnected world, there are many ways in which a system collapse might be triggered. As we observe the global financial chaos of 1998 caused by the "economic Asian flu," we can onlyponder what could happen if Tokyo were devastated in the near future by either a volcanic eruption or an earthquake? What if one or more of the many volcanoes in populous Indonesia has one of its millennial-scale eruptions? Or, what if Mount Ranier took out Seattle and Microsoft just before the millennium?
These four examples illustrate the bifocal perspective that a geological gaze can provide--parallel views of distant and near spaces and times. With it, we can observe the Earth's surface and its depths and look back in time. We can bring that vision to bear on present problems around the Earth and those in the foreseeable future. With this way of considering the world, geological knowledge can elucidate the problems of our physical environment that impact people and other living things inhabiting it.Although most people may never gain an intuitive familiarity with rare events, we can bring rare events into our collective consciousness by recognizing the nature of the world immediately surrounding us. Once we achieve general consciousness about the possibility of rare events, we can discuss their social, legal, political, scientific, technological, and economic implications. But people must appreciate that some predictions may be relatively good, or in some instances even very good, whereas others may have very large uncertainties.Nonetheless, rare events will happen--the great focal length of geology's bifocal lens tells us so. Rare occurrences will continue to shape human and natural history. We will help ourselves and others if we grasp this perspective.page 18: Excerpt from Annals of the Former World by John McPhee. © 1998 by John McPhee. page 112: Excerpt from Eight Little Piggies by Stephen Jay Gould. © 1993 by Stephen Jay Gould.
(Continues...)
Excerpted from Earth Around Us, The by Jill Schneiderman. Copyright © 2000 Jill Schneiderman. Excerpted by permission of Henry Holt and Co..
All rights reserved. No part of this excerpt may be reproduced or reprinted without permission in writing from the publisher.
Excerpts are provided by Dial-A-Book Inc. solely for the personal use of visitors to this web site.