The Dynamic Structure of the Deep Earth: An Interdisciplinary Approach / Edition 1 available in Hardcover
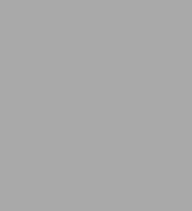
The Dynamic Structure of the Deep Earth: An Interdisciplinary Approach / Edition 1
- ISBN-10:
- 0691095116
- ISBN-13:
- 9780691095110
- Pub. Date:
- 05/26/2003
- Publisher:
- Princeton University Press
- ISBN-10:
- 0691095116
- ISBN-13:
- 9780691095110
- Pub. Date:
- 05/26/2003
- Publisher:
- Princeton University Press
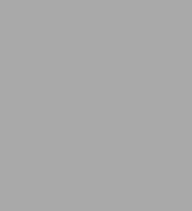
The Dynamic Structure of the Deep Earth: An Interdisciplinary Approach / Edition 1
Hardcover
Buy New
$88.00Buy Used
$52.77-
-
SHIP THIS ITEM
Temporarily Out of Stock Online
Please check back later for updated availability.
-
Overview
The richly varied dynamic processes in Earth's deep interior can be understood only through a better understanding of the properties of materials under the conditions prevalent there, Karato emphasizes. Materials properties change dramatically in the deep interior, and this has decisive consequences on mantle convection, which is the engine of plate tectonics. The importance of the hydrogen in water in determining the structure of the lithosphere-asthenosphere boundary is discussed, as is the role of phase transformation in controlling the density and plastic flow properties of Earth's materials. Models for enigmatic observations such as deep earthquakes and the anisotropic structures at the center of the Earth are covered in detail. The Dynamic Structure of the Deep Earth is an indispensable text for advanced-level undergraduate students and graduate students of Earth sciences, materials sciences, and physics.
Product Details
ISBN-13: | 9780691095110 |
---|---|
Publisher: | Princeton University Press |
Publication date: | 05/26/2003 |
Edition description: | First Edition |
Pages: | 256 |
Product dimensions: | 6.00(w) x 9.25(h) x (d) |
About the Author
Read an Excerpt
The Dynamic Structure of the Deep Earth
An Interdisciplinary ApproachBy Shun-ichiro Karato
Princeton University Press
Copyright © 2003 Princeton University PressAll right reserved.
ISBN: 0-691-09511-6
Chapter One
THE STRUCTURE OF EARTH AND ITS CONSTITUENTS1-1. EARTH'S INTERIOR: RADIAL STRUCTURE, CHEMICAL COMPOSITION, AND PHASE TRANSFORMATIONS
Inferring Earth's composition is a prerequisite to understanding its evolution and dynamics as well as those of planets like it. One might think that the composition of Earth can be easily inferred from the rocks that we can see on Earth's surface. However, it immediately becomes obvious that these rocks cannot be the major constituent of Earth's interior because the densities of typical rocks on Earth's surface, such as granite or basalt, are so small, even if the effects of compression on density are taken into account. Therefore, materials in the deep Earth (and most other planets) are different from those on the surface. What materials are there, and how do we infer the composition of the deep interior of Earth (and other planets)? You may want to drill into Earth, but the deepest drilled hole in the world is in the Kola peninsula in Russia, which is only ~ 12 km deep (remember that Earth's radius is 6,370 km). Although some kinds of volcanoes bring materials from the deep mantle, this sampling is usually limited to ~ 200 km deep. Therefore, our inference of Earth's internal structure must be based largely on indirect information. In this connection, both geochemical and geophysical observations are particularly relevant. In geochemistry, the scientist measures the chemical composition of various materials, then uses the chemical rules that govern the distribution of various chemical elements to infer the chemical composition of Earth. In geophysics, one measures physical properties such as seismic wave velocities and density, and infers the composition and structure of Earth's interior based on the physical principles that control the variation of physical properties with thermodynamic conditions (pressure and temperature). In this chapter, I will first summarize these basic observations, and then explain several models of Earth that have been proposed on the basis of geochemical and/or geophysical lines of inference. Although the inference of composition through such a process is indirect and not unique, some aspects of Earth's interior are now well understood. However, a number of problems remain controversial, including the nature of chemical heterogeneity in Earth's mantle.
1-1-1. Geochemical Models
An obvious starting point for inferring the chemical composition of Earth is the composition of rocks that we can collect on Earth's surface. We have a large data set of the composition of these rocks. Rocks from the shallow regions, the crust, are typically basalt or granite (rocks made mostly of quartz, pyroxenes, and plagioclase) or rocks that have been modified from these rocks by later chemical reactions (metamorphism). These rocks have a high silica content and small densities. Occasionally, we find denser rocks, peridotite or eclogite (rocks made mostly of olivine, pyroxenes, and spinels or garnets), which contain a smaller amount of silica and higher densities than basalt or granite. These rocks are obviously the candidates of mantle materials. However, petrological and geochemical studies show that the rocks that we can see on Earth's surface may not be representative of the bulk of Earth's mantle. It is important to note that Earth's shallow region has undergone extensive chemical differentiation through partial melting (melting of only some components) and the composition of each layer in the shallow regions is likely to reflect these differentiation processes. Consequently, the deeper portions of Earth's mantle likely have a composition different from that of rocks that we can collect on Earth's surface. One needs a theory for chemical differentiation in Earth to infer the chemical composition of Earth from the composition of rocks in near surface regions.
Ted Ringwood, at Australian National University (ANU) at Canberra, Australia, was a leader of the study of Earth's interior through a geochemical, petrological approach. He was perhaps the greatest Earth scientist from Australia. He made a number of fundamental contributions to knowledge of the structure and evolution of Earth and other terrestrial (Earth-like) planets. After obtaining a doctorate in geology at Melbourne in 1956, he studied with Francis Birch at Harvard. He returned to Australia in 1959 to take a position at the newly formed institution at ANU, and remained there until his premature death in 1993. Most of Ringwood's predictions or models of Earth's structures and evolution were based on simple principles, but he had an ingenious sense of synthesis, and most of his models have survived the test of time. Ringwood proposed a model of Earth's chemical composition based on a theory of chemical differentiation in Earth (1975). The starting point of his model is the notion that the formation of basalt through partial melting of mantle materials is the most important volcanic activity on Earth. The mantle materials must then be able to produce basalt (more precisely, mid-ocean ridge basalts [MORB]) by partial melting. From this line of argument, Ringwood proposed a hypothetical rock called pyrolite, which produces mid-ocean ridge basalt by partial melting, and he proposed that the majority of the mantle must be composed of pyrolite. Pyrolite is rich in magnesium and iron, similar to rocks brought from the mantle, but its silica concentration is slightly higher than typical mantle samples and it has more calcium, aluminum, and other elements. In this model, typical mantle samples on the surface are interpreted as residue from the partial melting of pyrolite. Ringwood suggested that the majority of the mantle (including the upper and lower mantle) is composed of materials with a chemical composition similar to pyrolite. In this hypothesis, the depth variation of density and the elastic properties in the mantle must be explained as a result of phase transitions, compression, and thermal expansion of the same material.
Another model is that Earth has the same chemical composition as the average composition of the solar system. It is generally believed that the Sun and the planets in the solar system were formed as a result of the collapse of a putative primitive solar nebula. Therefore, the composition of the sun and the other materials of the solar system should be approximately the same. The composition of the outer layer of the sun can be inferred from the analysis of its optical properties (indeed, the element helium, He, was discovered by the analysis of the optical spectrum of the Sun, hence its name [helios means "the Sun" in Greek]). Another source of information on the composition of the solar system comes from the composition of meteorites. Meteorites are considered to be fragments of materials that failed to become planets. Among various types of meteorites, carbonaceous chondrite is a unique type, which is made of a mixture of various materials including metallic iron, silicates, and organic materials. The age of this type of meteorite, inferred from the composition of radiogenic isotopes, is the oldest (~4.56 billion years) among the ages of various objects in the solar system. Therefore, this type of meteorite is considered to be a remnant of the primitive solar system. The chemical composition of carbonaceous chondrite agrees well with that of the Sun except for the volatile elements. Consequently, the composition of the carbonaceous chondrite is considered to be representative of the composition of the solar system. Some scientists consider that Earth has a chemical composition that is similar to that of (carbonaceous) chondrite (except for volatile components). This model is often referred to as the chondrite model.
Table 1-1 shows chemical compositions corresponding to the pyrolite model and the chondrite model. One significant difference between these models is the ratio of (Mg + Fe)/Si. In the chondrite model, the amount of silicon in the mantle is greater than that in the pyrolite model. Based on various sources of information, on the other hand, the chemical composition of the upper mantle is estimated to be very similar to pyrolite or have slightly less silicon than pyrolite. Therefore, if Earth has the chondritic chemical composition, the amount of silicon in the deep mantle must be greater than in the shallower mantle. One possibility is a silicon-rich lower-mantle model; some people consider that the lower mantle consists mostly of (Mg, Fe)Si[O.sub.3]. Thus, while the ratio of (Mg + Fe)/Si is constant throughout the entire mantle in the pyrolite model, the chondrite model has a smaller (Mg + Fe)/Si ratio in the lower mantle than in the upper mantle.
More complicated geochemical models have also been proposed. Don Anderson at Caltech (e.g., 1989), Eiji Ohtani at Tohoku University in Japan (1985), and Carl Agee, then at Harvard (1993), proposed mantle models containing several chemically different layers. Their thinking is based on a possible scenario for Earth's formation. Based on the results of Apollo missions, many Earth scientists believe that there was an extremely voluminous melting event due to high temperature caused by energy released via high-velocity collisions that occurred during the formation of the planets. This putative extensively molten region is called the magma ocean. They proposed that upon cooling of magma ocean, various minerals would have been solidified and would have sunk or floated to form chemical layering.
I must emphasize that these models have many uncertainties; their role is not to give some definitive picture of Earth structure, but to provide testable hypotheses. This point is particularly important in relation to geochemical models. Some rules of geochemistry are very well established and nearly independent of physical conditions. A good example is the rule of the radioactive decay of elements, which causes a temporal variation of isotope compositions. Also, the rule of partitioning some elements between liquids (magmas) and solids (minerals) is relatively well established. However, the physical processes that govern the distribution of chemically distinct materials in Earth are highly dependent on material properties that depend strongly on physical and chemical conditions, and these results should not be interpreted dogmatically to reach conclusions on chemical composition. For example, it is common to all models that the mantle basically consists of [(Mg, Fe).sub.2] Si[O.sub.4] (olivine and its high-pressure polymorphs) and (Mg, Fe) Si[O.sub.3] (pyroxene and its high-pressure polymorphs), and there is no doubt that (Mg, Fe)O is richer in the mantle than in the crust. For more detailed issues such as the depth variation of the (Mg + Fe)/Si ratio, however, we cannot draw conclusions only from these models. For example, Earth as a whole did not necessarily have the average chemical composition of the solar system. In the primitive solar system, its chemical composition was likely spatially variable, and it is unlikely that Earth and carbonaceous chondrites were formed from the same part of the primitive solar system. Furthermore, even if there was a magma ocean, it is not obvious that its solidification resulted in chemical layering. Brian Tonks and Jay Melosh (1990), at the University of Arizona at Tempe, have argued that crystallization from the magma ocean could result in a nearly homogeneous chemical composition because of stirring and mixing by vigorous convection during solidification.
So far we have discussed only major element compositions. The distribution of trace elements (elements that occur only in a minor content) is also important in relation to material circulation in Earth's interior. Because trace elements exist only in a small amount by definition, their influence on physical properties such as density is often small. However, since their distribution varies greatly by partial melting (because most of trace elements have different sizes or different electric charges than the major elements do), the distribution of trace elements provides an important clue as to the processes of partial melting and the resultant chemical evolution of Earth. Important trace elements are incompatible elements, such as rare earth elements, which concentrate in the melt phase when rocks partially melt. If we examine the concentration of trace elements in igneous rocks such as basalt, we can find the chemical composition of their parental rocks. From these studies, we now know that there are several source regions in the mantle for igneous rocks. In some regions, rocks there do not contain very much of these incompatible elements. We infer that these regions have undergone extensive chemical differentiation by partial melting that has removed most of the incompatible elements. In other regions, in contrast, rocks contain large amounts of incompatible elements. These regions are inferred to have undergone a lesser degree of partial melting, or a large amount of materials enriched in these elements (sediments, crustal rocks, etc.) have been added in these regions. The differences in chemical composition lead to different isotope ratios because different elements have different radioactive decay schemes. In this way, we can infer the chemical evolution of different regions in Earth. It is now agreed that there are at least two chemically distinct regions in Earth that have been isolated from each other more than one billion years (Hofmann 1997). The distribution of these source regions is controversial and is the subject of active research (see chap. 4 for detail).
Hydrogen occupies a unique position among the trace elements. In most of the geochemical literature, hydrogen is not treated as a trace element, but its quantity in normal minerals is low, and hydrogen is dissolved in melts more than in minerals. Therefore, hydrogen behaves like an incompatible element. Like other trace elements, its influence on density is small, but its influence on some physical properties is large. In particular, hydrogen affects melting behavior (melting temperature and the composition of molten materials) and significantly reduces the resistance of materials against plastic flow. Through its effects on plastic properties, hydrogen can also change the nature of seismic wave propagation. I will discuss these issues in some detail in chapter 2.
1-1-2. Geophysical Models
Any model for the interior of Earth (or other planets) must be consistent not only with geochemical observations, but also with geophysical observations. Important geophysical observations include density and elastic properties. The average density of Earth can be easily calculated from geodetic observations of its size and the total mass. The average density of Earth is estimated to be 5,515 kg/[m.sup.3].
Continues...
Excerpted from The Dynamic Structure of the Deep Earth by Shun-ichiro Karato Copyright © 2003 by Princeton University Press. Excerpted by permission.
All rights reserved. No part of this excerpt may be reproduced or reprinted without permission in writing from the publisher.
Excerpts are provided by Dial-A-Book Inc. solely for the personal use of visitors to this web site.
What People are Saying About This
This book is certainly an exciting contribution to the field. It fills the need for a solid Earth science textbook that can convey the excitement of the research frontier. Most previous work had relied on speculation. Professor Karato is very knowledgeable at interpreting seismology with mineral physics. And he is an independent thinker.
Toshiro Tanimoto, University of California, Santa Barbara
This is an exciting and eye-opening, well-written book that provides an updated overview of theoretical aspects of solid earth science. It does so quite successfully. The very sound scholarship reflects substantial original contributions by the author while also paying due attention to classic works.
Seiya Uyeda, Professor Emeritus, University of Tokyo
"This book is certainly an exciting contribution to the field. It fills the need for a solid Earth science textbook that can convey the excitement of the research frontier. Most previous work had relied on speculation. Professor Karato is very knowledgeable at interpreting seismology with mineral physics. And he is an independent thinker."—Toshiro Tanimoto, University of California, Santa Barbara"This is an exciting and eye-opening, well-written book that provides an updated overview of theoretical aspects of solid earth science. It does so quite successfully. The very sound scholarship reflects substantial original contributions by the author while also paying due attention to classic works."—Seiya Uyeda, Professor Emeritus, University of Tokyo
Recipe
"This book is certainly an exciting contribution to the field. It fills the need for a solid Earth science textbook that can convey the excitement of the research frontier. Most previous work had relied on speculation. Professor Karato is very knowledgeable at interpreting seismology with mineral physics. And he is an independent thinker."Toshiro Tanimoto, University of California, Santa Barbara
"This is an exciting and eye-opening, well-written book that provides an updated overview of theoretical aspects of solid earth science. It does so quite successfully. The very sound scholarship reflects substantial original contributions by the author while also paying due attention to classic works."Seiya Uyeda, Professor Emeritus, University of Tokyo