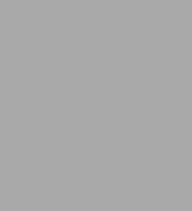
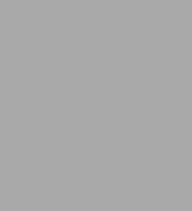
eBook
Available on Compatible NOOK devices, the free NOOK App and in My Digital Library.
Related collections and offers
Overview
Product Details
ISBN-13: | 9780190285425 |
---|---|
Publisher: | Oxford University Press |
Publication date: | 04/06/2000 |
Sold by: | Barnes & Noble |
Format: | eBook |
File size: | 3 MB |
About the Author
Read an Excerpt
Chapter One
Newton Gets It Completely Wrong
Matter, in the everyday world, comes in three forms: solid, liquid, and gas. Gas is the simplest form of matter, the form that we understand best. We live in a gas, in fact, a mixture of gases--air. The way that scientists see gases is not the way that our eyes see them. In fact our eyes don't see air at all, but it is typical of science that it starts off with the somewhat thin evidence of the senses and then proceeds, by experiment and hypothesis, to build a model that explains the properties of whatever system we are interested in. One of the most successful models is that constructed to explain the behavior of gases. The key to the understanding of gases is to believe in the microscopic motion of the bits of matter of which a gas is composed--the molecules. Newton got this wrong, and we can profit from seeing both why he thought that he was right and why we think that he wasn't.
I am going to ask the reader to accept certain facts on faith. This is not a disreputable cop-out. We all do it every day. Scientists certainly do it--we have no choice. I am a physical chemist. The only way that I can intelligently apprehend modern genetics, for example, is by taking certain things for granted and using my reason to build on them. This blind faith in what I read in reviews or selected research papers is not to be likened to an unquestioning belief in flying saucers. No one has demonstrated the existence of flying saucers in a manner that would satisfy either a sloppy scientist or a critical layman. In this book I will, unless specifically stated, use only facts that have been accepted as such by the international scientific community. Where a fact is in reality only a hypothesis I shall say so, and I will tell you when I think that I'm on shaky ground. It is quite possible that some of my so-called facts will sooner or later turn out to be fiction--I am not the pope. For the moment all I ask is unqualified acceptance. This sounds like the spiel of a secondhand car salesman, but if you don't believe something in this book, you can go to standard textbooks. If you question their validity, you can take a specialized course of lectures. If doubt remains, follow your doubt; you may be the next Galileo.
Molecules
In this chapter, and elsewhere in this book, the word molecule will make many appearances. What is a molecule? For the moment think of it this way: Take a glass of water and divide its contents into four cups; you will still call the substance in each cup "water." Now take one of the four cups and divide the water in it into a thousand droplets. Each drop is still recognizably water. Take one of these droplets and divide it into a million droplets, each one visible only under a microscope. All tests, physical or chemical, will verify that each drop is a sample of water. Can we go on with this game indefinitely? There have been those in the past who thought so, who believed that matter was a continuum, an infinitely divisible Jell-o. Today we know that the game stops somewhere. There comes a stage when the pieces of water, if split, divide into fragments that are very definitely not water. It's a little like splitting up a crowd of demonstrators; there comes a time when you get down to individuals who, if split up, are no longer people.
The smallest entity that still has the right to be called water is termed a molecule of water. Look at Figure 1.1. Most of you will recall that water is also known as [H.sub.2]O, which is just a shorthand way of saying that each molecule of water consists of two hydrogen atoms and one oxygen atom. (The word atom also needs more explanation, and we will return to it later on.) The smallest part of a sample of oxygen gas that is still recognizably the oxygen that we breathe is the oxygen molecule, which consists of two oxygen atoms linked together, and which is therefore written as [O.sub.2]. If you split an oxygen molecule in half, you will get two oxygen atoms whose physical, chemical, and biological properties are utterly different from those of the oxygen molecule. Ozone has the chemical formula [O.sub.3]. Each ozone molecule has three oxygen atoms joined together (Figure 1.1).
It is not surprising that we can chop up a sample of water apparently almost endlessly and still have water. Molecules tend to be very small entities. A teaspoonful of water contains about 200,000,000,000,000,000,000,000 water molecules. Textbooks like to suggest hypothetical counting games as an aid to the visualization of very large numbers. In accordance with this tradition, I can tell you that if the whole population of Earth set out to count the molecules in a teaspoon of water, each person counting at the rate of one molecule per second, it would take over a million years. But let's get back to gases.
The Invisible Sea
We live at the bottom of an invisible sea. Nature, working through gigantic astro-physical, geological, and biological processes, has generated for us a molecular atmosphere of gas within which we live and without which we cannot survive. Those few of us, such as divers or astronauts, who leave the gaseous sea have to take some of it with us, or we die. We need gases--and not just the air. Man uses gases to weld steel, to fill laser tubes and neon lights, to anesthetize patients, to give sparkle to soft drinks, beer, and champagne, to fly airships, float wrecks, cook hamburgers, inflate tires, and commit genocide. Deep under the ground, the decomposition of primeval forests by heat and pressure has given us coal and inflammable natural gas, which is an important, if minor, source of energy. In the meantime, we pollute the atmosphere with gases that at best erode buildings and change the color of oil paintings, and at worst poison life and perilously disturb the delicate heat balance of this planet. Gases can be indispensable to man or catastrophically destructive. As Willy Loman might have said, attention must be paid to such substances.
If you walk into a chemical supply company, you can purchase samples of many different gases. Some are familiar enough: oxygen, nitrogen, carbon dioxide, carbon monoxide, helium, chlorine. These are substances that appear weekly in the daily press. The chemical properties of these gases are so different as to suggest that there is no important basic property shared by all gases. Consider two well-known gases.
Helium exists in the stars, in a few underground cavities, and in very small quantities in the air. It was first discovered in the Sun, by examining the nature of the Sun's light--hence its name, from the Greek for Sun, Helios. Helium is a complete wimp of a gas, almost incapable of interacting with any other substance, which is why it avoided detection for so long. Chlorine, on the other hand, attacks almost everything and is reminiscent of the mythical chemist who discovered a universal solvent but failed to find a bottle capable of holding it. It kills painfully, as British and French soldiers found out in 1917. It is pale green, one of the few visible gases.
In general, chemical experience suggests that each gas is unique, which is true, and has very little, if anything, in common with most other gases, which is not true. That which is common to all gases is the way in which their molecules move. That is the real subject of this chapter, and it has implications that reach far beyond the nature of gases.
The Violent Crowd
Look at Figure 1.2. It shows an imaginary cubical box constructed in the air of the room in which you are sitting. The way that the molecules in it behave has been the subject of controversy for at least a century. The secret of time may reside in this box.
Note how small the box is. The letters nm stand for nanometer, which is one thousand millionth part of a meter, which can be written [10.sup.-9] meter. If everyone in China had one of these boxes and, by order of the Party, lay the boxes end to end, touching each other, the total length of the line would be only about five meters.
Descartes thought that the universe was continuous, like jelly, but he didn't do any experiments to back up his guess. Look at the box. The six objects represent six molecules drawn to scale. Oxygen and nitrogen molecules look very much the same, so we don't differentiate between them in our picture. It is clear that air is mainly empty space. The average distance between molecules in the air around you is about ten times their largest dimension. It is as though the average distance between canaries in a cage was about 1 meter.
So that you don't forget the kind of numbers we are dealing with, bear in mind that a teaspoonful of the air in your house contains somewhat more than [10.sup.20] molecules (1 with twenty zeros after it). This is about 2000 times less than the number of water molecules in a teaspoonful of water. Water molecules in liquid water are crowded together; the living conditions in liquids are slumlike compared with those in gases.
Two vital facts are not revealed by the drawing:
* Molecules in a gas are in constant motion.
* The average speed of a molecule in a gas increases with temperature.
Be very clear what this motion is and is not. We are not talking about drafts, winds, hurricanes, or convection currents. Even in a tightly closed room in the absence of breathing creatures, moving machinery, or sources of light or heat, the gas molecules are always moving. In the cylinder of oxygen standing in the hospital storeroom, the oxygen molecules are endlessly in motion. And they are moving fast. Very fast.
An Aside: Why Molecular Motion?
There is no such thing as a motionless atom or molecule, and there is hardly an aspect of the nonliving or living physical world which can be completely understood without taking into account molecular motion. The concept of temperature and the nature of heat both have a deep connection with molecular motion, and molecular motion has a strange connection with the arrow of time and the death of the universe.
Back to the Madding Crowd
Professors have a weakness for analogies. So here's one: A gas, any gas, is similar to a crowd of flies. The analogy is dangerous, but we can learn from the dangers. First of all, flies can see; they don't normally bump into each other. Molecules are "blind"; in a gas they are continually blundering into each other. Every collission changes the speed and direction of both molecules involved, so that a molecule in a gas resembles a flying dodgem car, continually getting jolted. Another difference between flies and molecules is that the molecules in our box are presumed to fly in straight lines unless they hit something. Flies practice their aeronautical skills. An improved fly analogy is a crowd of straight-flying, blind, deaf flies, but this is still misleading. Flies get tired. They often relax, and in the end they die and lie on the floor with their legs up. Molecules don't do this; the molecules in an oxygen cylinder never stop moving--until the end of time, as they say at MGM. Again improving our analogy, we liken the molecules in a gas to a collection of straight-flying, blind, deaf, radarless, tireless, immortal flies. We're getting there, but the problem, as we will soon see, is that flies have a sense of smell and molecules don't. First, however, let's look at the speeds of molecules.
How fast do molecules move in a gas? That depends on two things, the mass of the molecule (less correctly, its weight) and the temperature of the gas. The question needs sharpening. Since any given molecule is continually changing direction and speed due to collisions, it is more informative to speak not of its "speed" but of its average speed. If you drive from home to office, your speed is hardly constant; it drops to zero at red lights and rises a little above the speed limit on the freeway. However, you can work out your average speed from only two observations: just divide the distance from home to office by the time for the complete journey. Average speeds for molecules in a gas can be both measured experimentally and calculated theoretically. Here are some approximate average speeds, in miles per hour, for molecules in some still gases at room temperature:
Hydrogen in a steel cylinder 3800
Helium in an airship 2800
Oxygen, on a dreamy day in St. Tropez 1030
Carbon dioxide in a soda-syphon refill 830
The masses of these molecules increase along the series: hydrogen, helium, oxygen, carbon dioxide. Here we see the mass dependence of the average speed: at the same temperature a heavier molecule travels slower on the average than a lighter molecule. The average speed of the molecules in a sample of gas is a constant quantity, the same today as it was 500 years ago, provided the temperature is the same. If the temperature goes up, the average speed goes up. The average speed of an oxygen molecule rises by about 7% in going from 0[degrees]C (32[degrees]F) to 40[degrees]C (104[degrees]F). Not a stupendous increase, but it has practical implications. We will see that it is because particles get faster as they get hotter that the Sun is able to radiate energy.
You might care to compare molecular speeds in gases to the speeds of more familiar objects, again in miles per hour: Concorde in full flight 1450
Lamborghini, cruising 180
Ben Johnson, 1988 Olympics (assisted) 22.8
Carl Lewis, 1988 Olympics (unassisted) 22.6
Now consider the following problem, which is based on the fact that although solutions of alcohol, like beer, are liquid, the smell of alcohol can be easily detected. This is because in all liquids, molecules escape into the surrounding air. If they didn't, liquids would not evaporate. At room temperature the calculated average speed of an alcohol molecule is about 800 mph. Here's the problem:
A diligent, but worldly-wise, professor of physics returns from a late-night "seminar" and gingerly opens his front door. Why is it that his whisky-laden breath is not immediately detected by his loving, and equally worldly-wise, wife, who is waiting for him, 10 yards away, at the foot of the marble stairs? After all, molecules traveling at an average speed of 800 mph should be able to cover 10 yards in about one-fortieth of a second. In fact, if the air in the room were still, it would take months for the smell of alcohol to be detectable at that distance. Why? Why is the professor's secret safe until he plants a guilty kiss on his wife's skeptical cheek?
The answer lies in the astronomical number of collisions that a molecule makes as it blunders about in a gas. If you could follow the path of a single molecule of, say, oxygen in the air, you would find that in round numbers, it collided with other molecules about 6000 million times in one second. Molecules have a hectic social life, even by jet-set standards. An oxygen molecule in air changes direction at an incredible rate. It would take a very long time to fight its way across a room. The fact that you can quickly detect the smell of cooking all over the kitchen is due to the help given to the molecules by drafts or convection currents which move whole chunks of air rapidly from place to place. Similarly, if you put a drop of ink in a bath of motionless water, the color will spread out because of molecular motion, but it will spread very slowly. Drop the ink in a river, and it will travel downstream at a rate determined by the bulk flow of the water.
The difficulty that a molecule has in fighting its way across the room is analogous (here we go again) to a passenger trying to get to his platform during rush hour at Grand Central Station and being jostled by other passengers going in different directions. Again we can learn from the imperfect analogy. The passenger knows where he wants to go and directs his feet in that direction, when allowed to between pushes. In contrast, molecules in a static sample of gas have no predetermined destination: they are not heading anywhere, they just move. After every collision they travel in a direction determined by the details of the collision, like billiard balls, but in three dimensions, not two. Molecules have no will of their own. That is why the fly in our previous analogy had to have no sense of smell, since otherwise, even if blind and deaf, it might direct its flight toward food. The essence of molecular motion in gases is that it is completely determined by the last collision. We say that the motion is random. The flight of the fly is not random.
The professor's wife knows that the alcohol molecules leaving her husband's lips, once they have slowed down from the initial push given by his lungs, travel between two well-separated points at less than a snail's pace. Calculation shows that in six months the average, point-to-point distance traveled between starting point and final destination, by an alcohol molecule in still air, is about 10 yards. The total zigzag path length traveled is about 35 million miles!
War would be farcical if projectiles traveled through the air in the way that molecules do. Billy the Kid would have looked even stupider than he was if his bullets had performed a random dance after leaving his gun. However, a bullet weighs about [10.sup.23] times as much as an oxygen or nitrogen molecule and is far too heavy to be diverted by such puny objects. Linebackers aren't usually stopped in their tracks by gnats. They are, however, slowed down by air resistance, which is basically the cumulative effect a myriad of collisions between a moving macroscopic object and the molecules that it encounters. The same effect works if you try sprinting through a cornfield.
A Pause for Doubt
We have converted the air from a structureless, thin, invisible soup into a horde of frenetic particles--molecules--forever on the move, battering into each other at tremendous speeds and incredible frequency. The average speed of the molecules in the sample remains constant as long as the temperature remains constant. What we have found is genuine perpetual motion. Stubborn, deluded inventors are still seen sitting confidently in the corridors of patent offices, carrying the plans of ingenious contraptions that once set in motion are supposed to run forever, in the absence of any subsequent input of energy. They don't run and they won't run; only nature has taken out a patent on perpetual motion. At least that's the story that I've been telling. But is it true? I have given you the basic assumptions that go into the scientist's model of a gas. Could there be an entirely different and more correct model?
History shows that even the greatest minds can construct theories that work but that have one minor flaw--they are completely erroneous.
Newton Gets It Wrong
Thomas Willis (1621-1675), a professor of natural philosophy at Oxford, discovered the crippling disease myasthenia gravis. He was an iatrochemist, a physician who believed in the chemical basis of the body's workings, so it was natural for him to be aware of his famous contemporary, the chemist Robert Boyle. One of Willis's brighter students was Robert Hooke, and when Boyle was looking for an assistant, Willis recommended Hooke. Thus it was that in 1655, the twenty-year-old Hooke came to work with the twenty-eight-year-old Boyle.
The conjunction of Boyle and Hooke was heaven-sent. Hooke knew how to construct air pumps of the type that had been invented in 1650 by Otto von Guericke of Magdeburg. Hooke built a pump for Boyle, who, by creating a moderate vacuum in a glass vessel, showed that air was necessary for life (mice died), for combustion (fires went out), and for the passage of sound. (Von Guericke had already shown that light, but not sound, traveled through a vacuum.) In a famous experiment, Boyle trapped a volume of air inside a glass tube and varied the pressure on it. You can do the same by putting your finger over the nozzle of a bicycle pump and pushing the handle in. As the handle enters farther into the barrel of the pump, the volume of the trapped air decreases and you must push harder to keep the pump handle where it is. It is as though you are striving to compress a spring. Boyle's qualitative observation--that when the pressure on the air sample increases, the volume decreases--is not very surprising. But Boyle did something that was still a rarity in the seventeenth century; he made quantitative measurements. He recorded the pressure and the volume of the air during the experiments. He found that when he doubled the pressure on a sample of air, the volume halved. When the pressure went up three times, the volume went down to a third of the original volume. All his experiments were done at about the same temperature, that of his laboratory. He concluded that for a sample of gas kept at constant temperature, the pressure of the sample multiplied by its volume was unchangeable. As one got bigger, the other got smaller. We write this result as: pressure times volume = a constant, and call it Boyle's law.
Boyle's law, published in 1662, is one of the first examples of a physical law. Any theory about the nature of gases has to lead to Boyle's law; if it doesn't, it may be wrong. (In fact Boyle's law it is not an exact law, but it is not bad under normal circumstances.)
Boyle sought an explanation for the "spring" of the air and proposed that it consisted of "many slender and flexible hairs," like a sheep's fleece, "each of which may indeed, like a little spring be easily bent or rolled up." The problem with this idea is that as a theory it is useless. It qualitatively explains Boyle's observation, but you can't derive Boyle's law, or any other property of a gas, from Boyle's theory.
The Curse of the Fat Sumo
What happened next was an excellent example of a completely incorrect theory agreeing perfectly with experimental results. This has happened more than once in the history of science, and the present case contains a warning that applies to any kind of explanation, scientific or not. Thus the theory that sumo wrestling tends to makes you fat is beautifully confirmed by the fact that almost all sumo wrestlers are enormously bulky. In the absence of any other experimental data, the theory looks good. The curse of the fat sumo fell on Newton. He succeeded in elegantly explaining Boyle's law. Incorrectly.
Newton proposed that the particles of the air (we would call them molecules) were motionless in space and were held apart by repulsive forces between them, so that any attempt to reduce the volume of a sample of gas was analogous to the compression of springs. He assumed that the repulsive force was inversely proportional to the distance between the particles: double the distance and the force is halved He showed that, on the basis of this assumption, a collection of static particles in a box would behave exactly as Boyle had found. His model led straight to Boyle's law. Probably the greatest scientist ever, Newton managed to get the right answer from a model that was wrong in every possible way. Experiment shows that molecules in a gas are not motionless and, contrary to Newton's second assumption, that molecules almost always attract each other unless they are actually touching. Nevertheless, if all that we knew about the behavior of gases was Boyle's law, there would be no grounds for challenging Newton's theory. It gives the right answer. Unfortunately, Newton's model does not predict or explain any other property of gases. He had been struck by the curse of the fat sumo. Beware.
We believe that we have a better model than Newton's. It explains far more facts than his does, as we will see in Chapter 16, and the final test of a model is whether it explains the experimental facts. Nevertheless, we again ask: Why should we believe it to be true? Are molecules really moving continually, even in an undisturbed, closed container? Maybe there is a better theory waiting around the corner. Maybe we will also find that new facts about gases will be completely inconsistent with our present ideas.
Science is not carved on tablets of marble. Theories have arisen, have worked, have been challenged, and have been superseded. So why should you believe anything that I have written up to now? How skeptical should you be about the pronouncements of scientists?