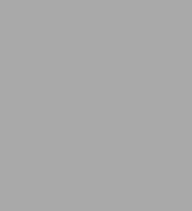
Star Ware: The Amateur Astronomer's Guide to Choosing, Buying, and Using Telescopes and Accessories
432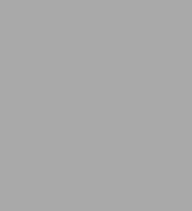
Star Ware: The Amateur Astronomer's Guide to Choosing, Buying, and Using Telescopes and Accessories
432eBook
Available on Compatible NOOK devices, the free NOOK App and in My Digital Library.
Related collections and offers
Overview
"Star Ware is still a tour de force that any experienced amateur will find invaluable, and which hardware-minded beginners will thoroughly enjoy."
- Robert Burnham, Sky&Telescope magazine
"Star Ware condenses between two covers what would normally take a telescope buyer many months to accumulate."
- John Shibley, Astronomy magazine
Whether you're shopping for your first telescope or your fifth, don't be surprised if you feel overwhelmed by the dazzling array of product choices, bells and whistles, and the literature that describes them all. That's why you need Star Ware.
In this revised and updated Fourth Edition of the essential guide to comparing and selecting sky-watching equipment, award-winning astronomy writer Philip Harrington takes you telescope shopping the easy way. He analyzes and explains today's astronomy market and compares brands and models point by point. Star Ware gives you the confidence you need to buy the telescope and accessories that are right for you and the knowledge to get the most out of your new purchase, with:
*
Extensive, expanded reviews of leading models and accessories-including dozens of new products
*
A clear, step-by-step guide to every aspect of selecting telescopes, binoculars, filters, mounts, lenses, cameras, film, star charts, guides and references, and much more
*
Ten new do-it-yourself projects for building your own astronomical equipment
*
Easy tips on setting up, using, and caring for telescopes and other astronomical equipment
*
Lists of where to find everything astronomical, including Web sites and resources; distributors, dealers, and conventions; and corporate listings for products and services
Product Details
ISBN-13: | 9781118046333 |
---|---|
Publisher: | Trade Paper Press |
Publication date: | 02/25/2011 |
Sold by: | Barnes & Noble |
Format: | eBook |
Pages: | 432 |
File size: | 10 MB |
About the Author
Read an Excerpt
Star Ware
The Amateur Astronomer's Guide to Choosing, Buying, and Using Telescopes and AccessoriesBy Philip S. Harrington
John Wiley & Sons
ISBN: 0-471-41806-4Chapter One
Parlez-Vous "Telescope"?Before the telescope, ours was a mysterious universe. Events occurred nightly that struck both awe and dread into the hearts and minds of early stargazers. Was the firmament populated with powerful gods who looked down upon the pitiful Earth? Would the world be destroyed if one of these deities became displeased? Eons passed without an answer.
The invention of the telescope was the key that unlocked the vault of the cosmos. Though it is still rich with intrigue, the universe of today is no longer one to be feared. Instead, we sense that it is our destiny to study, explore, and embrace the heavens. From our backyards we are now able to spot incredibly distant phenomena that could not have been imagined just a generation ago. Such is the marvel of the modern telescope.
Today's amateur astronomers have a wide and varied selection of equipment from which to choose. To the novice stargazer, it all appears very enticing but very complicated. One of the most confusing aspects of amateur astronomy is telescope vernacular-terms whose meanings seem shrouded in mystery. "Do astronomers speak a language all their own?" is the cry frequently echoed by newcomers to the hobby. The answer is yes, but it is a language that, unlike some foreign tongues, iseasy to learn. Here is your first lesson.
Many different kinds of telescopes have been developed over the years. Even though their variations in design are great, all fall into one of three broad categories according to how they gather and focus light. Refractors, shown in Figure 1.1a, have a large lens (the objective) mounted in the front of the tube to perform this task, whereas reflectors, shown in Figure 1.1b, use a large mirror (the primary mirror) at the tube's bottom. The third class of telescope, called catadioptrics (Figure 1.1c), places a lens (here called a corrector plate) in front of the primary mirror. In each instance, the telescope's prime optic (objective lens or primary mirror) brings the incoming light to a focus and then directs that light through an eyepiece to the observer's waiting eye. Although chapter 2 addresses the history and development of these grand instruments, we will begin here by exploring the many facets and terms that all telescopes share. As you read through the following discussion, be sure to pause and refer to the diagrams found in chapter 2. This way, you can see how individual terms relate to the various types of telescopes.
Aperture
Let's begin with the basics. When we refer to the size of a telescope, we speak of its aperture. The aperture is simply the diameter (usually expressed in inches, centimeters, or millimeters) of the instrument's prime optic. In the case of a refractor, the diameter of the objective lens is cited, whereas in reflectors and catadioptric instruments, the diameters of their primary mirrors are specified. For instance, the objective lens in Galileo's first refractor was about 1.5 inches in diameter; it is therefore designated a 1.5-inch refractor. Sir Isaac Newton's first reflecting telescope employed a 1.33-inch mirror and would be referred to today as a 1.33-inch Newtonian reflector.
Many amateur astronomers consider aperture to be the most important criterion when selecting a telescope. In general (and there are exceptions to this, as pointed out in chapter 3), the larger a telescope's aperture, the brighter and clearer the image it will produce. And that is the name of the game: sharp, vivid views of the universe.
Focal Length
The focal length is the distance from the objective lens or primary mirror to the focal point or prime focus, which is where the light rays converge. In reflectors and catadioptrics, this distance depends on the curvature of the telescope's mirrors, with a deeper curve resulting in a shorter focal length. The focal length of a refractor is dictated by the curves of the objective lens as well as by the type of glass used to manufacture the lens.
As with aperture, focal length is commonly expressed in either inches, centimeters, or millimeters.
Focal Ratio
Looking through astronomical books and magazines, it's not unusual to see a telescope specified as, say, an 8-inch f/10 or a 14-inch f/4.5. This f-number is the instrument's focal ratio, which is simply the focal length divided by the aperture. Therefore, an 8-inch telescope with a focal length of 56 inches would have a focal ratio (f-ratio) of f/7, because 56 / 8 = 7. Likewise, by turning the expression around, we know that a 6-inch f/8 telescope has a focal length of 48 inches, because 6 x 8 = 48.
Readers familiar with photography may already be used to referring to lenses by their focal ratios. In the case of cameras, a lens with a faster focal ratio (that is, a smaller f-number) will produce brighter images on film, thereby allowing shorter exposures when shooting dimly lit subjects. The same is true for telescopes. Instruments with faster focal ratios will produce brighter images on film, reducing the exposure times needed to record faint objects. However, a telescope with a fast focal ratio will not produce brighter images when used visually. The view of a particular object through, say, an 8-inch f/5 and an 8-inch f/10 will be identical when both are used at the same magnification. How bright an object appears to the eye depends only on telescope aperture and magnification.
Magnification
Many people, especially those new to telescopes, are under the false impression that the higher the magnification, the better the telescope. How wrong they are! It's true that as the power of a telescope increases, the apparent size of whatever is in view grows larger, but what most people fail to realize is that at the same time, the images become fainter and fuzzier. Finally, as the magnification climbs even higher, image quality becomes so poor that less detail will be seen than at lower powers.
It's easy to figure out the magnification of a telescope. If you look at the barrel of any eyepiece, you will notice a number followed by mm. It might be 26 mm, 12 mm, or 7 mm, among others; this is the focal length of that particular eyepiece expressed in millimeters. Magnification is calculated by dividing the telescope's focal length by the eyepiece's focal length. Remember to first convert the two focal lengths into the same units of measure-that is, both in inches or both in millimeters. A helpful hint: There are 25.4 millimeters in an inch.
For example, let's figure out the magnification of an 8-inch f/10 telescope with a 26-mm eyepiece. The telescope's 80-inch focal length equals 2,032 millimeters (80 x 25.4 = 2,032). Dividing 2,032 by the eyepiece's 26-mm focal length tells us that this telescope/eyepiece combination yields a magnification of 78x (read 78 power), because 2,032 / 26 = 78.
Most books and articles state that magnification should not exceed 60 x per inch of aperture. This is true only under ideal conditions, something most observers rarely enjoy. Due to atmospheric turbulence (what astronomers call poor seeing), interference from artificial lighting, and other sources, many experienced observers seldom exceed 40x per inch. Some add a caveat to this: Never exceed 300x even if the telescope's aperture permits it. Others insist there is nothing wrong with using more than 60per inch as long as the sky conditions and optics are good enough. As you can see, the issue of magnification is always a hot topic of debate. My advice for the moment is to use the lowest magnification required to see what you want to see, but we are not done with the subject just yet. Magnification will be spoken of again in chapter 5.
Light-Gathering Ability
The human eye is a wondrous optical device, but its usefulness is severely limited in dim lighting conditions. When fully dilated under the darkest circumstances, the pupils of our eyes expand to about a quarter of an inch, or 7 mm, although this varies from person to person-the older you get, the less your pupils will dilate. In effect, we are born with a pair of quarter-inch refractors.
Telescopes effectively expand our pupils from fractions of an inch to many inches in diameter. The heavens now unfold before us with unexpected glory. A telescope's ability to reveal faint objects depends primarily on the diameter of either its objective lens or primary mirror (in other words, its aperture), not on magnification; quite simply, the larger the aperture, the more light gathered. Doubling a telescope's diameter increases light-gathering power by a factor of four, tripling its aperture expands it by nine times, and so on.
A telescope's limiting magnitude is a measure of how faint a star the instrument will show. Table 1.1 lists the faintest stars that can be seen through some popular telescope sizes. Trying to quantify limiting magnitude is anything but precise due to a large number of variables. Apart from aperture, other factors affecting this value include the quality of the telescope's optics, meteorological conditions, light pollution, excessive magnification, apparent size of the target, and the observer's vision and experience. These numbers are conservative estimates; experienced observers under dark, crystalline skies can better these by perhaps half a magnitude or more.
Resolving Power
A telescope's resolving power is its ability to reveal fine detail in whatever it is aimed at. Though resolving power plays a big part in everything we look at, it is especially important when viewing subtle planetary features, small surface markings on the Moon, or searching for close-set double stars.
A telescope's ability to resolve fine detail is always expressed in arc-seconds. You may remember this term from high-school geometry. Recall that in the sky there are 90° from horizon to the overhead point, or zenith, and 360° around the horizon. Each one of those degrees may be broken into 60 equal parts called arc-minutes. For example, the apparent diameter of the Moon in our sky may be referred to as either 0.5° or 30 arc-minutes, each one of which may be further broken down into 60 arc-seconds. Therefore, the Moon may also be sized as 1,800 arc-seconds.
Regardless of the size, quality, or location of a telescope, stars will never appear as perfectly sharp points. This is partially due to atmospheric interference and partially due to the fact that light consists of slightly fuzzy waves rather than mathematically straight lines. Even with perfect atmospheric conditions, what we see is a blob, technically called the Airy disk (named in honor of its discoverer, Sir George Airy, Britain's Astronomer Royal from 1835 to 1892). Because light is composed of waves, rays from different parts of a telescope's prime optic (be it a mirror or lens) alternately interfere with and enhance each other, producing a series of dark and bright concentric rings around the Airy disk (Figure 1.2a). The whole display is known as a diffraction pattern. Ideally, through a telescope without a central obstruction (that is, without a secondary mirror), 84% of the starlight remains concentrated in the central disk, 7% in the first bright ring, and 3% in the second bright ring, with the rest distributed among progressively fainter rings.
Figure 1.2b graphically presents a typical diffraction pattern. The central peak represents the bright central disk, and the smaller humps show the successively fainter rings.
The apparent diameter of the Airy disk plays a direct role in determining an instrument's resolving power. This becomes especially critical for observations of close-set double stars. How large an Airy disk will a given telescope produce? Table 1.2 summarizes the results for most common amateur-size telescopes.
Although these values would appear to indicate the resolving power of the given apertures, some telescopes can actually exceed these bounds. The nineteenth-century English astronomer William Dawes found experimentally that the closest a pair of 6th-magnitude yellow stars can be to each other and still be distinguishable as two points can be estimated by dividing 4.56 by the telescope's aperture. This is called Dawes' Limit (Figure 1.3). Table 1.3 lists Dawes' Limit for some common telescope sizes.
When using telescopes of less than 6-inch aperture, some amateurs can readily exceed Dawes' Limit, while others will never reach it. Does this mean that they are doomed to be failures as observers? Not at all! Remember that Dawes' Limit was developed under very precise conditions that may have been far different than your own. Just as with limiting magnitude, reaching Dawes' Limit can be adversely affected by many factors, such as turbulence in our atmosphere, a great disparity in the test stars' colors and/or magnitudes, misaligned or poor-quality optics, and the observer's visual acuity.
Rarely will a large-aperture telescope-that is, one greater than about ten inches-resolve to its Dawes' Limit. Even the largest backyard instruments can almost never show detail finer than between 0.5 arc-seconds (abbreviated 0.5") and 1 arc-second (1"). In other words, a 16- to 18-inch telescope will offer little additional detail compared to an 8- to 10-inch one when used under most observing conditions. Interpret Dawes' Limit as a telescope's equivalent to the projected gas mileage of an automobile: "These are test results only-your actual numbers may vary."
We have just begun to digest a few of the multitude of telescope terms that are out there. Others will be introduced in the succeeding chapters as they come along, but for now, the ones we have learned will provide enough of a foundation for us to begin our journey.
(Continues...)
Excerpted from Star Ware by Philip S. Harrington Excerpted by permission.
All rights reserved. No part of this excerpt may be reproduced or reprinted without permission in writing from the publisher.
Excerpts are provided by Dial-A-Book Inc. solely for the personal use of visitors to this web site.
Table of Contents
Preface to the Fourth Edition.Acknowledgments.
1. Parlez Vous "Telescope?"
2. In the Beginning.
3. So You Want to Buy a Telescope.
4. Two Eyes are Better than One.
5. Attention Shoppers.
6. The "Eyes" Have It.
7. The Right Stuff.
8. The Homemade Astronomer.
9. Till Death Do You Part.
10. A Few Tricks of the Trade.
Appendices.
A. Specs at a Glance.
B. Eyepiece Marketplace.
C. The Astronomical Yellow Pages.
D. An Astronomer's Survival Guide.
E. Astronomical Resources.
F. English/Metric Conversion.
G. Star Ware Reader Survey.