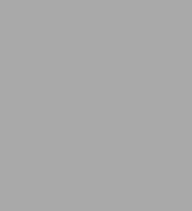
Self-Organization in Biological Systems
562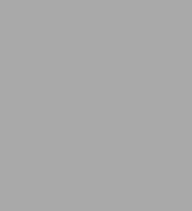
Self-Organization in Biological Systems
562eBook
Available on Compatible NOOK devices, the free NOOK App and in My Digital Library.
Related collections and offers
Overview
The synchronized flashing of fireflies at night. The spiraling patterns of an aggregating slime mold. The anastomosing network of army-ant trails. The coordinated movements of a school of fish. Researchers are finding in such patterns--phenomena that have fascinated naturalists for centuries--a fertile new approach to understanding biological systems: the study of self-organization. This book, a primer on self-organization in biological systems for students and other enthusiasts, introduces readers to the basic concepts and tools for studying self-organization and then examines numerous examples of self-organization in the natural world.
Self-organization refers to diverse pattern formation processes in the physical and biological world, from sand grains assembling into rippled dunes to cells combining to create highly structured tissues to individual insects working to create sophisticated societies. What these diverse systems hold in common is the proximate means by which they acquire order and structure. In self-organizing systems, pattern at the global level emerges solely from interactions among lower-level components. Remarkably, even very complex structures result from the iteration of surprisingly simple behaviors performed by individuals relying on only local information. This striking conclusion suggests important lines of inquiry: To what degree is environmental rather than individual complexity responsible for group complexity? To what extent have widely differing organisms adopted similar, convergent strategies of pattern formation? How, specifically, has natural selection determined the rules governing interactions within biological systems?
Broad in scope, thorough yet accessible, this book is a self-contained introduction to self-organization and complexity in biology--a field of study at the forefront of life sciences research.
Product Details
ISBN-13: | 9780691212920 |
---|---|
Publisher: | Princeton University Press |
Publication date: | 05/05/2020 |
Series: | Princeton Studies in Complexity , #7 |
Sold by: | Barnes & Noble |
Format: | eBook |
Pages: | 562 |
File size: | 49 MB |
Note: | This product may take a few minutes to download. |
About the Author
Read an Excerpt
COPYRIGHT NOTICE: Published by Princeton University Press and copyrighted, © 2001, by Princeton University Press. All rights reserved. No part of this book may be reproduced in any form by any electronic or mechanical means (including photocopying, recording, or information storage and retrieval) without permission in writing from the publisher, except for reading and browsing via the World Wide Web. Users are not permitted to mount this file on any network servers.
What Is Self-Organization?
Technological systems become organized by commands from outside, as when human intentions lead to the building of structures or machines. But many natural systems become structured by their own internal processes: these are the self-organizing systems, and the emergence of order within them is a complex phenomenon that intrigues scientists from all disciplines.F. E. Yates et al., Self-Organizing Systems: The Emergence of OrderSelf-Organization Defined
Self-organization refers to a broad range of pattern-formation processes in both physical and biological systems, such as sand grains assembling into rippled dunes (Figure 1.1), chemical reactants forming swirling spirals (Figure 1.3a), cells making up highly structured tissues, and fish joining together in schools. A basic feature of these diverse systems is the means by which they acquire their order and structure. In self-organizing systems, pattern formation occurs through interactions internal to the system, without intervention by external directing influences. Haken (1977, p. 191) illustrated thiscrucial distinction with an example based on human activity: “Consider, for example, a group of workers. We then speak of organization or, more exactly, of organized behavior if each worker acts in a well-defined way on given external orders, i.e., by the boss. We would call the same process as being self-organized if there are no external orders given but the workers work together by some kind of mutual understanding.” (Because the “boss” does not contribute directly to the pattern formation, it is considered external to the system that actually builds the pattern.)
Systems lacking self-organization can have order imposed on them in many different ways, not only through instructions from a supervisory leader but also through various directives such as blueprints or recipes, or through pre-existing patterns in the environment (templates).
To express as clearly as possible what we mean by self-organization in the context of pattern formation in biological systems, we provide the following definition: Self-organization is a process in which pattern at the global level of a system emerges solely from numerous interactions among the lower-level components of the system. Moreover, the rules specifying interactions among the system’s components are executed using only local information, without reference to the global pattern. In short, the pattern is an emergent property of the system, rather than a property imposed on the system by an external ordering influence. Emergent properties will be defined in later chapters, but for now suffice to say that emergent properties are features of a system that arise unexpectedly from interactions among the system’s components. An emergent property cannot be understood simply by examining in isolation the properties of the system’s components, but requires a consideration of the interactions among the system’s components. It is important to point out that system components do not necessarily have to interact directly. As described in Chapter 2, and Figure 2.4, individuals may interact indirectly if the behavior of one individual modifies the environment and thus affects the behavior of other individuals.
Pattern in Group Activities
Critical to understanding our definition of self-organization is the meaning of the term pattern. As used here, pattern is a particular, organized arrangement of objects in space or time. Examples of biological pattern include a school of fish, a raiding column of army ants, the synchronous flashing of fireflies, and the complex architecture of a termite mound. Examples of other biological patterns include lichen growth (Figure 1.2a), pigmentation patterns on shells, fish and mammals (Murray 1988, Meinhardt 1995), (Figures 1.2b,c,d) and the ocular dominance stripes in the visual cortex of the macaque monkey brain (Hubel and Wiesel 1977) (Figure 1.2e).
To understand how such patterns are built, it is important to note that in some cases the building blocks are living units — fish, ants, nerve cells, etc. — and in others they are inanimate objects such as bits of dirt and fecal cement that make up the termite mound. In each case, however, a system of living cells or organisms builds a pattern and succeeds in doing so with no external directing influence, such as a template in the environment or directions from a leader. Instead, the system’s components interact to produce the pattern, and these interactions are based on local, not global, information. In a school of fish, for instance, each individual bases its behavior on its perception of the position and velocity of its nearest neighbors, rather than knowledge of the global behavior of the whole school. Similarly, an army ant within a raiding column bases its activity on local concentrations of pheromone laid down by other ants rather than on a global overview of the pattern of the raid.
The literature on nonlinear systems often mentions self-organization, emergent properties, and complexity as well as dissipative structures and chaos (Prigogine and Glansdorf 1971; Nicolis and Prigogine 1989). The terms chaos and dissipative structures have precise scientific meanings that may differ from popularized definitions, so it is important to discuss these terms at this point. To begin with, the term complex is a relative one. Individual organisms may use relatively simple behavioral rules to generate structures and patterns at the collective level that are relatively more complex than the components and processes from which they emerge. As discussed in Chapter 6 (see Box 1), systems are complex not because they involve many behavioral rules and large numbers of different components but because of the nature of the system’s global response. Complexity and complex systems, on the other hand, generally refer to a system of interacting units that displays global properties not present at the lower level. These systems may show diverse responses that are often sensitively dependent on both the initial state of the system and nonlinear interactions among its components. Since these nonlinear interactions involve amplification or cooperativity, complex behaviors may emerge even though the system components may be similar and follow simple rules.
Complexity in a system does not require complicated components or numerous complicated rules of interaction.
Self-Organization in Biology
The concept of self-organization in biological systems can be conveyed through counterexamples. A marching band forming immense letters on a foot-ball field provides one such example. Here the band’s members are guided in their behavior by a set of externally imposed instructions for the movements of each band member that specify in fine detail the final configuration of the whole band. A particular member of the band may know that the instructions are to march to the 50-yard line, turn left 90 degrees and march 10 paces. To the extent that the band member follows this recipe for contributing to the pat-tern and ignores local information, such as position relative to neighbors, this pattern formation would not be considered self-organized.
Similarly, a team of carpenters building a house is a pattern-formation process that functions without self-organization. Here members of the construction crew are guided in their collective behavior by predetermined externally imposed instructions expressed as blueprints, that precisely specify the final structure of the house. Letter formation by a marching band and house construction by a construction crew both involve pattern building in space.
Let us also consider two counterexamples to self-organization that involve pattern building over time. One such example is oarsmen in a rowing team pulling on their oars in perfect synchrony with one another and with appropriate adjustments of their stroke frequency. This pattern arises when each oars-man responds to the coxswain’s shouted instructions indicating when to begin each stroke. Clearly, this is an example of a group generating a pattern by following explicit orders from a leader based on the overall state of the group members. The rhythmic contractions of muscle fibers in the heart are also a counterexample to self-organization. Here the pattern arises as the component building blocks (the muscle fibers), follow instructions from special excitable cells that act as an external pacemaker and send a rhythmic electrical signal to the fibers.1
We can easily see how a system can form a precise pattern if it receives instructions from outside—such as a blueprint, recipe, or signals from a pacemaker—but it is less obvious how a definite pattern can be produced in the absence of such instructions. A general answer to this puzzle is provided in the next chapter, while specific answers for particular biological patterns constitute the main body of this book. For now, it is merely asserted that pattern formation often is achieved by systems without external guidance.
The mechanisms of self-organization in biological systems differ from those in physical systems in two basic ways. The first is the greater complexity of the subunits in biological systems. The interacting subunits in physical systems are inanimate objects such as grains of sand or chemical reactants. In biological systems there is greater inherent complexity when the subunits are living organisms such as fish or ants or neurons.
The second difference concerns the nature of the rules governing interactions among system components. In chemical and physical systems, pattern is created through interactions based solely on physical laws. For example, heat applied evenly to the bottom of a tray filled with a thin sheet of viscous oil transforms the smooth surface of the oil into an array of hexagonal cells of moving fluid called Bénard convection cells (Figure 1.3) (Velarde and Normand 1980). The molecules of oil obey physical laws related to surface tension, viscosity, and other forces governing the motion of molecules in a heated fluid. Likewise, when wind blows over a uniform expanse of sand a pattern of regularly spaced ridges is formed (Figure 1.1) through a set of forces attributable to gravity and wind acting on the sand particles (Anderson 1990; Forrest and Haff 1992).
Of course, biological systems obey the laws of physics, but in addition to these laws the physiological and behavioral interactions among the living components are influenced by the genetically controlled properties of the components. In particular, the subunits in biological systems acquire information about the local properties of the system and behave according to particular genetic programs that have been subjected to natural selection. This adds an extra dimension to self-organization in biological systems, because in these systems selection can finely tune the rules of interaction. By tuning the rules, selection shapes the patterns that are formed and thus the products of group activity can be adaptive. What is also intriguing about pattern formation in biological systems and lends excitement to studies of self-organization in animal groups is the recent realization that interactions among system components can be surprisingly simple, even when extremely sophisticated patterns are built, such as the labyrinthine nests of termites, the spatial patterns of army ant raids, and the coordinated movements of fish in a school.
Table of Contents
Explanation of Color Plates viiPrologue: Aims and Scope of the Book 2
Part I. Introduction to Biological Self-Organization 5
Chapter 1. What Is Self-Organization? 7
Chapter 2. How Self-Organization Works 15
Chapter 3. Characteristics of Self-Organizing Systems 29
Chapter 4. Alternatives to Self-Organization 47
Chapter 5. Why Self-Organization? 63
Chapter 6. Investigation of Self-Organization 69
Chapter 7. Misconceptions about Self-Organization 88
Part II. Case Studies 93
Chapter 8. Pattern Formation in Slime Molds and Bacteria 95
Chapter 9. Feeding Aggregations of Bark Beetles 121
Chapter 10. Synchronized Flashing among Fireflies 143
Chapter 11. Fish Schooling 167
Chapter 12. Nectar Source Selection by Honey Bees 189
Chapter 13. Trail Formation in Ants 217
Chapter 14. The Swarm Raids of Army Ants 257
Chapter 15. Colony Thermoregulation in Honey Bees 285
Chapter 16. Comb Patterns in Honey Bee Colonies 309
Chapter 17. Wall Building by Ants 341
Chapter 18. Termite Mound Building 377
Chapter 19. Construction Algorithms in Wasps 405
Chapter 20. Dominance Hierarchies in Paper Wasps 443
Part III. Conclusions 483 Chapter 21. Lessons, Speculations, and the Future of
Self-Organization 485
Notes 495
References 497
Index 525
What People are Saying About This
The authors' lively yet scholarly account of the rapidly emerging new science of group behavior should captivate anyone who has an inquiring mind and a curiosity about what new directions biology is taking,including undergraduate and graduate students looking for an exciting new way to do biology. This book is a major contribution,not only to biology,but well beyond.
This ambitious volume has the potential to be a pivotal (even breakthrough) contribution to the biology of complex systems. It aims to facilitate both conceptual understanding and also correct application of the principles of self-organization in a biological context.
"The authors' lively yet scholarly account of the rapidly emerging new science of group behavior should captivate anyone who has an inquiring mind and a curiosity about what new directions biology is taking, including undergraduate and graduate students looking for an exciting new way to do biology. This book is a major contribution, not only to biology, but well beyond."—Robert Jeanne, University of Wisconsin-Madison"This ambitious volume has the potential to be a pivotal (even breakthrough) contribution to the biology of complex systems. It aims to facilitate both conceptual understanding and also correct application of the principles of self-organization in a biological context."—Kern Reeve, Cornell University