Selenium: Chemistry, Analysis, Function and Effects / Edition 1 available in Hardcover
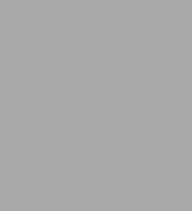
Selenium: Chemistry, Analysis, Function and Effects / Edition 1
- ISBN-10:
- 1849738912
- ISBN-13:
- 9781849738910
- Pub. Date:
- 08/25/2015
- Publisher:
- RSC
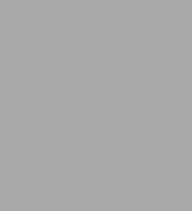
Selenium: Chemistry, Analysis, Function and Effects / Edition 1
Hardcover
Buy New
$279.00-
SHIP THIS ITEM— Not Eligible for Free Shipping
Overview
Part of The Food and Nutritional Components in Focus series, this edited volume pools knowledge across scientific disciplines in a way that increases its applicability to a wide range of audiences. Victor Preedy’s own distinguished career in nutritional science has made him a prolific author of research articles and books in this area, and this project fills a gap in providing comprehensive synopses of food substances.
Chemists, analytical scientists, forensic scientists, food scientists, as well as course lecturers will all benefit from this interdisciplinary title written by international experts in this area.
About the Author
Read an Excerpt
Selenium
Chemistry, Analysis, Function and Effects
By Victor R. Preedy
The Royal Society of Chemistry
Copyright © 2015 The Royal Society of ChemistryAll rights reserved.
ISBN: 978-1-84973-891-0
CHAPTER 1
The Chemistry of Selenium
DANIEL PERRONE, MARIANA MONTEIRO, AND JULIANA CÔRTES NUNES
1.1 Chemical Properties of Selenium
Selenium was discovered in 1818 by Jöns Jacob Berzelius, a Swedish chemist who also isolated and described the elements cerium (1803), zirconium (1824), silicon (1824) and thorium (1828). The name selenium derives from the Greek word for "moon", selene, in opposition to the Latin word for "earth", tellus. Selenium is a nonmetal with symbol Se and atomic number 34, located in the forth period of the periodic table in the chalcogen group, along with oxygen, sulfur and tellurium. Its atomic and physical properties are listed in Table 1.1.
In nature, selenium is found in ore minerals, partially substituting sulfur. Selenium can exist as multiple allotropes (Butterman and Brown, 2004) that is, it can exist in essentially different molecular forms with varying physical properties. Both amorphous (without crystalline shape) and crystalline varieties of selenium occur. Amorphous selenium is best known as the "vitreous" and the finely divided brick red forms, which are frequently described as two distinct allotropes. These forms are, however, identical at the microscopic level and their distinct appearance is only due to different routes of production. The crystalline allotropes include several monoclinic varieties, red to brown in color, as well as the so-called "metallic" gray or black selenium. The most thermodynamically stable allotrope is the hexagonal, crystalline form to which both major monoclinic forms convert at temperatures above 110 °C and to which amorphous selenium converts spontaneously at 70–120 °C.
Elemental selenium is relatively nontoxic and is considered to be an essential trace element. Commonly, it can be reduced to the -2 (selenide, Se-2) oxidation state or oxidized to the +4 (selenite, SeO32-) or +6 (selenate, SeO42-) oxidation states. Hydrogen selenide (H2Se) and other selenium compounds are extremely toxic (LD50 6 ppm for guinea pigs), and resemble arsenic in their physiological reactions. Selenium in the -2 oxidation state is the dominant form in organoselenium chemistry. Selenide is an important metabolite in animals and some micro-organisms, since it is the precursor of selenocysteine at the active centers of many selenoenzymes (Rayman, 2004). Going down in the chalcogen group, bond strength becomes increasingly weaker (341 kJ mol-1, 272 kJ mol-1 and 234 kJ mol-1 for the C–O, C–S and C–Se bonds, respectively) and longer (141 pm, 181 pm and 198 pm for the C–O, C–S and C–Se bonds, respectively). Selenium compounds are more nucleophilic and acidic than the corresponding sulfur compounds.
The selenium standard atomic mass is 78.963 Da and shows six naturally occurring isotopes, five of which are stable: 74Se, 76Se, 77Se, 78Se, 80Se (Rosman and Taylor, 1998; Jörg et al., 2010). The other naturally occurring isotope, 82Se, is considered to be stable for practical reasons since it has a very long half-life (approximately 1020 years), yielding 82Kr via a double beta decay. Data regarding selenium naturally occurring isotopes are listed in Table 1.2. Although many studies have used repletion of tissue selenium or glutathione peroxidase activity as criteria for determination of bioavailability, most of the selenium stable isotopes have been employed for the study of its role as an essential nutrient. These isotopes may be produced as highly enriched materials at an affordable cost (Young et al., 1982) and may be analyzed by mass spectrometry or neutron activation analysis. More recently, stable 77Se and 82Se were employed to estimate selenium bioavailability to men (Fox et al., 2004). This study was notable for the use of foods intrinsically labeled with 77Se stable isotope, as well as for the double-label method (82Se as selenate) that allowed each individual to serve as their own control.
So far, twenty-four unstable selenium isotopes have been described (Audi et al., 2003), showing half-lives ranging from approximately 20 ms to 295 000 years (Table 1.3). Selenium radioactive isotopes are mainly used for the production of medical and industrial bromine radioisotopes. Since some selenium radioactive isotopes show a very long half-life, their study is of great relevance for dose calculations performed in the frame of geological disposal of long-lives radioactive waste.
The selenium valence electronic configuration ([Ar] 3d10 4s2 4p4) is equivalent to that of sulfur ([Ne] 3s2 3p4), which explains their similar atomic and physicochemical properties such as atomic sizes (both in covalent and ionic states), bond energies, ionization potentials and electronegativities. Despite these similarities, selenium compounds differ from sulfur compounds in two important chemical features, which are pivotal to differentiate their role in biological systems: their redox behavior and their acidity. Selenium compounds tend to be metabolized to more reduced states while sulfur compounds show an opposite behavior, tending to be metabolized to more oxidized states. Moreover, although their oxyacids show similar acid strengths (pKa 2.6 and pKa 1.9 for species with Se+4 and S+4; pKa 3.0 for both species with Se+6 and S+6), H2Se is much more acidic (pKa 3.8) than H2S (pKa 7.0). This difference in hydrides acidity is reflected in the dissociation behaviors of the selenohydryl group (–SeH) of selenocysteine (pKa 5.24) and the sulfhydryl group (–SH) of cysteine (pKa 8.25). Consequently, selenocysteine and other selenols are mainly dissociated at physiological pH, while cysteine and other thiols are mainly nondissociated or protonated under the same conditions (Jacob et al., 2003).
1.2 Organoselenium Compounds
Selenium occurs in different chemical forms, which determine its bioavailability, function and toxicity to human health. While inorganic selenium can be found in different minerals in the form of selenites and selenates, main organic forms are selenoaminoacids, selenopeptides and selenoproteins (Maseko et al., 2013). Thus, the physiology or the biochemistry of organic selenium is mediated basically by its incorporation into selenoproteins. Indeed, the majority of selenium in plants is found in selenoaminoacids, such as selenomethionine and methylselenocysteine (Dumont et al., 2006; Figure 1.1).
Organoselenium compounds can be defined as chemical compounds that contain both selenium and carbon. Other elements, such as halogens, oxygen, sulfur, or nitrogen are also frequently present in their structure. In general, organoselenium compounds have greater bioavailability than that of inorganic selenium and also are usually found to be less toxic than the inorganic forms (Amoako et al., 2009). In addition to their low toxicity, organoselenium compounds play important roles in biochemical processes such as antioxidants, anticancer and antiviral agent (Mugesh et al., 2001; Soriano-Garcia, 2004). For this reason, in the last few years, a number of novel synthetic organoselenium compounds have been synthesized. Experimental evidence suggests that modification of organoselenium compounds can have a profound effect on their chemistry and consequently on their biological activity (Plano et al., 2010).
The main classes of organoselenium compounds include selenols, diselenides, selenoxides, selenones, selenium acids, selenides, selenium halides and selenaheterocyclic compounds (Table 1.4). As mentioned before, the chemical properties of organoselenium compounds resemble their sulfur analogs and may be classified similarly. Therefore, selenols, selenides, and selenoxides correspond to thiols, sulfides, and sulfoxides. Despite their structural similarities, sulfur and selenium compounds are often different with respect to their stability, chemical properties and route of synthesis. Owing to the stronger metallic properties of selenium as compared to those of sulfur, the C–Se bond is more easily broken by halogens and oxidizing agents than is the C–S bond. For instance, Briviba et al. (1996) demonstrated that organoselenium compounds protected against peroxynitrite and that this protection was more efficient than that exerted by the corresponding sulfur analogs. This selectivity is attributed to a more easily oxidation of selenium in comparison to sulfur.
Selenols are one of the main classes of organoselenium compounds, and the most important selenol is the amino acid selenocysteine. Various enzymes found in mammals such as glutathione peroxidase, iodothyronine deiodinase and thioredoxin reductase contain selenols at their active sites (Pappas et al., 2008). Selenols are strong nucleophiles that react with alkyl halides and epoxides yielding selenides (Back, 2011). They are also strong reducing agents that can convert N–O and N–N compounds into amines, dihalides into alkenes, and are also readily oxidized to diselenides by exposure to oxygen. Diselenides, such as diphenyl diselenide, have received significant attention, particularly with the discovery that these compounds possess a mode of antioxidant action similar to that of glutathione peroxidase (Rosa et al., 2007). Diselenides are usually prepared by either of two types of reaction: one in which the diselenide group is directly introduced into the molecule, or another in which a selenium-containing compound is oxidized or reduced to diselenide (Campbell et al., 1952). More recently, Freudendahl et al.(2009) reported the synthesis of diselenides by reduction of aldehydes or imines in the presence of carbon monoxide. Diselenides can be also further reduced to selenols, oxidized to selenenic, seleninic, or selenonic acids. Finally, halogenation of diselenides leads to selenenyl halides.
Selenenic acids are usually unstable and disproportionate into mixtures of diselenides and seleninic acids. Seleninic acids are typically more stable, and the corresponding aryl derivatives serve as useful oxidizing agents toward a variety of organic functional groups (Back, 2011).
When selenium forms covalent bonds with both a hydrocarbon substituent and a carbonitrile group, selenocyanates are obtained. Selenocyanates have been synthesized by reaction of substrates with potassium selenocyanate, a product commercially available on large scale. The most usual reactions involving this reagent are the nucleophilic substitutions of halogen atoms or other leaving groups as tosylates, the reaction with diazonium salts and the addition on carbon double or triple bond (Jean-Claude, 2011). Selenocyanates can also be synthesized by the reaction of diselenides with trialkylsilylcyanide or metallic cyanides (Jean-Claude, 2011). Among the synthetic selenium derivatives, selenocyanates are also an important class that acts as bioactive compounds, showing antioxidative, antimutagenic and anticarcinogenic properties (Das et al., 2007).
Selenaheterocyclic compounds demonstrates potential effects against various diseases acting as antioxidants, enzyme inhibitors, anti-inflammatory and anti-infective agents (Mugesh et al., 2001). These compounds are key intermediates employed in the synthesis of alkenes, alkynes and selenium-free heterocycles (Mlochowski et al., 2007). The structures of selenaheterocyclic compounds are closely related to those of analogous sulfur compounds, but their properties are often distinct. In most selenaheterocyclic rings selenium is bonded to carbon or to another heteroatom, such as Se, O, N or S. Some selenaheterocyclic systems containing Se–Te, Se–P, Se–B, Se–Zn or Se–Sn bonds have also been reported (Mlochowski et al., 2007).
Selenides are the most prevalent organoselenium compounds. They may be obtained by synthetic methods or occur as byproducts of other reactions. Selenides are generally stable compounds that can be alkylated to afford selenonium salts or oxidized to either the corresponding selenoxides or selenones.
1.3 Selenium in Soil and Foods
The content of selenium in foods varies according to geographical origin both within and between countries (Navarro-Alarcon and Cabrera-Vique, 2008). Selenium levels in plants are directly affected by selenium content in the soil. In animal products, these levels are directly related to their diet (Navarro-Alarcon and Cabrera-Vique, 2008; Rayman et al., 2008).
The distribution of selenium is uneven over the Earth's surface. Seleniferous and selenium deficient geo-ecosystems can be formed within limited geographic zones (Tan et al., 2002). Several areas, such as the Enshi province (China), the great plains of the USA and Canada and portions of Ireland, Colombia and Venezuela are known seleniferous areas and have high soil concentrations of selenium (>5 ppm) (Tan et al., 2002; Temmerman et al., 2014; Zhang et al., 2014). However, areas of low selenium (<0.05 ppm) or selenium-deficient soil are more common than areas of selenium-rich soil and according to the World Health Organization includes over 40 countries, such as China (Keshan area), Denmark, Finland, New Zealand and Russia (eastern and central Siberia) (Li et al., 2007).
Selenium levels in soil are not the only factor that determines the contents of selenium in foods. Different physicochemical conditions of the soils such as pH and redox potential are also important (Rayman, 2004; Spadoni et al., 2007; Zhao et al., 2007). The two major forms of selenium in soil are selenate and selenite. While selenite is the major species in-well aerated and neutral to alkaline soils, selenite is found in higher levels in well-drained mineral soils with pH from acid to neutral (Rayman, 2008). These characteristics are important because transport of selenite is both lower and slower than that of selenite (Spadoni et al., 2007; Zhu et al., 2009).
In soils, selenite is less bioavailable than selenate because it is strongly absorbed by iron oxides and/or hydroxides (Spadoni et al., 2007). In acid soils selenium is usually bound as a basic ferric selenite of extremely low solubility and it is essentially unavailable to plants. In alkaline soils selenium may be oxidized to selenate ions and become water soluble and readily available to plants (Rayman, 2008). However, as it is not easy to increase the pH of sandy soils extensively, fertilizers enriched with selenium could be an option to increase crop selenium concentrations (Temmerman et al., 2014).
A further important factor is that plant species differ in their ability to assimilate and accumulate selenium and can be divided into three groups: nonaccumulators, secondary selenium accumulators and selenium accumulators (Broadley et al., 2006; Rayman, 2008). The only selenium-accumulator plant that is used as a food source is Bertholletia excelsa, a tree that produces Brazil nuts, considered the richest selenium food source (800 to 8300 µg 100 g-1) (Thomson et al., 2008). Brassica (Indian mustard, rapeseed, broccoli, and cabbage), Allium (garlic, onions, leeks and wild leeks) and some mushrooms species of commercial importance can be described as secondary selenium accumulators (Broadley et al., 2006; Navarro-Alarcon and Cabrera-Vique, 2008). Cereal crops such as wheat, oats, rye and barley are nonaccumulators (Rayman, 2008) and therefore are not sources of selenium.
According to the literature, the typical selenium contents in foods varies as follows: organ meats, meats, eggs and seafood, 12.5 to 311.5 µg per 100 g; dairy products, less than 0.1 to 30 µg per 100 g; cereals, 1.0 to 55.0 µg per 100 g, whereas selenium in bread is generally found at levels of 6.0 to 16.0 µg per 100 g; fruits and vegetables, 0.1 to 2.2 µg per 100 g (Dumont et al., 2006; Navarro-Alarcon and Cabrera-Vique, 2008; Rayman, 2008). The main forms of selenium in plants and animal foods are selenomethionine, selenocysteine, methylselenocysteine and selenate. Selenomethionine is predominantly in cereals, methylselenocysteine in Brazil nuts and Brassica and Allium species and selenomethionine and selenate in animal foods (Dumont et al., 2006; Navarro-Alarcon and Cabrera-Vique, 2008; Rayman et al., 2008).
(Continues...)
Excerpted from Selenium by Victor R. Preedy. Copyright © 2015 The Royal Society of Chemistry. Excerpted by permission of The Royal Society of Chemistry.
All rights reserved. No part of this excerpt may be reproduced or reprinted without permission in writing from the publisher.
Excerpts are provided by Dial-A-Book Inc. solely for the personal use of visitors to this web site.