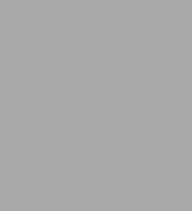
Searching for the Oldest Stars: Ancient Relics from the Early Universe
320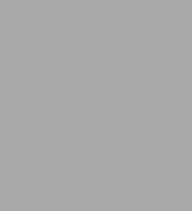
Searching for the Oldest Stars: Ancient Relics from the Early Universe
320eBook
Available on Compatible NOOK devices, the free NOOK App and in My Digital Library.
Related collections and offers
Overview
A leading astronomer takes readers behind the scenes of the thrilling science of stellar archaeology
Astronomers study the oldest observable stars in the universe in much the same way archaeologists study ancient artifacts on Earth. Anna Frebel takes readers into the far-flung depths of space and time to provide a gripping firsthand account of the cutting-edge science of stellar archaeology. Weaving the latest findings in astronomy with her own compelling insights as one of the world's leading researchers in the field, she explains how sections of the night sky are "excavated" in the hunt for these extremely rare, 13-billion-year-old relic stars and how this astonishing quest is revealing tantalizing new details about the origins and evolution of the cosmos. Along the way, Frebel recounts her own stories of discovery, offering an insider's perspective on this exciting frontier of science.
Product Details
ISBN-13: | 9781400874286 |
---|---|
Publisher: | Princeton University Press |
Publication date: | 11/17/2015 |
Sold by: | Barnes & Noble |
Format: | eBook |
Pages: | 320 |
File size: | 40 MB |
Note: | This product may take a few minutes to download. |
About the Author
Read an Excerpt
Searching for the Oldest Stars
Ancient Relics from the Early Universe
By Anna Frebel, Ann M. Hentschel
PRINCETON UNIVERSITY PRESS
Copyright © 2015 Princeton University PressAll rights reserved.
ISBN: 978-1-4008-7428-6
CHAPTER 1
WHAT IS STELLAR ARCHAEOLOGY?
To understand the many details and the prevalent chemical and physical processes in the Universe, we will embark on a cosmic journey through space and time. It starts directly with the Big Bang and will lead us from there to the present. As can be seen in Figure 1.1, we will first acquaint ourselves with the cosmic origin of an apple and from there also with that of the chemical elements. The most ancient stars from the time shortly after the Big Bang will assist us on this journey. They demonstrate that we humans are all children of the cosmos. Made mostly of star dust, we even carry small amounts of Big Bang material inside ourselves.
The American astronomer Carl Sagan once said, "If you wish to make an apple pie from scratch, you must first invent the Universe." The elements composing an apple are in fact the result of a cosmic production process that lasted billions of years. Astronomers call this the chemical evolution of the Universe. The atoms of an apple were first generated by processes of nuclear fusion in the hot cores of stars eons ago. By baking an apple pie we change the order of the atoms inside the apples' molecules, but the atoms themselves remain unchanged. To change one kind of atom into another, our kitchens would need to be equipped with nuclear reactors.
The elements hydrogen and helium were formed in the very early phases of the Big Bang and provide the basic material structure of the Universe. Soon afterward, the cosmic cooking of the other elements began. This is how all the elements were ultimately generated to form the basis for the emergence and evolution of life, and hence also of human beings. For humans and organic matter in general, carbon plays a crucial role, so our existence depends on the stars that synthesized that carbon. As humans, we thus have surprisingly close ties to the evolutionary history of the chemical elements.
By analyzing the different chemical and physical processes involved in this evolution, astronomers can inch their way closer to understanding the nature of the whole Universe. Plate 1.A outlines this evolution. But let us start at the beginning of the story.
1.1 The First Minutes after the Big Bang
We often use concepts like space and time, temperature and density without considering whether there ever existed a "before" this space or a "before" this time. Our physical understanding of the Universe begins just tiny fractions of a second after the Big Bang, which should be considered the beginning of space and time. What really existed before and right at the beginning remains a mystery. "Big Bang" simply represents this indescribable initial state.
We do know, though, that immediately after the Big Bang the Universe was extremely hot and consisted of a thick soup of various kinds of tiny particles. During the minutes that followed, protons, neutrons, and electrons — the building blocks of atoms — formed. The Universe then expanded rapidly and quickly cooled in the process. The only chemical element existing up to that point had been hydrogen (atomic number 1). To be more precise, only hydrogen nuclei, that is, protons, existed. After two to three minutes the temperature had dropped to one billion degrees. The first nuclei heavier than hydrogen, including deuterium, were formed. Deuterium is also called "heavy hydrogen" because it has the same atomic number as hydrogen, but it is composed of one proton and one neutron.
From deuterium, the first helium nuclei (atomic number 2) formed, consisting of two protons and two neutrons. During the first two minutes, when the temperature had been even higher, helium had also been forming directly from four protons. But those helium nuclei were immediately destroyed by highly energetic gamma radiation. The detour via deuterium at the cooler temperature of about one billion degrees then finally led to the formation of larger quantities of helium.
The collisions of several helium nuclei caused the third heaviest element, lithium (atomic number 3), to occasionally form, albeit in only extremely small amounts. The Universe was then composed of three elements: hydrogen, helium, and lithium. Roughly 75% of the total mass consisted of hydrogen, 25% helium, and merely 0.000000002% lithium. For comparison, expressing this distribution in percentages of hydrogen and helium atoms, there would be 92% hydrogen atoms and just 8% helium atoms because helium is four times heavier than hydrogen. Lithium, in turn, constitutes just a minuscule fraction.
The first phase of element synthesis was complete just three minutes after the Big Bang. The Universe had cooled down too far for continued nuclear fusion with hydrogen and helium. But for life to later evolve in the Universe and for humans to emerge, these three chemical elements were not enough. The elements needed to sustain life, including carbon, nitrogen, oxygen, and iron, as well as all other elements in the periodic table, were still missing. Those were later built up, nucleus by nucleus, inside stars over billions of years. Only the interiors of stars are hot enough for heavier elements to be successively synthesized from the available lighter elements, such as hydrogen and helium, and others, as time went on.
These stars, and later also galaxies, had to emerge first, however. For that, the positive, electron-less, atomic nuclei had to combine with the free electrons whizzing about the Universe to form neutral atoms. For quite some time after the Big Bang, these atomic nuclei, free electrons, and also photons were racing about in a cosmic jumble. The energy and direction of the photons were constantly being diverted — scattered — by free electrons. Hence, this soup of particles and rays was fairly opaque, similar to water droplets in the pouring rain or thick fog.
About 380,000 years after the Big Bang, the Universe had grown so much while cooling down that a fundamental change occurred when it reached about 3,000 K. The nuclei and electrons were moving slowly enough by then that the positively charged nuclei could capture the negatively charged electrons to bind them permanently. The photons that had been flying around since the Big Bang suddenly had much less chance of being scattered. Consequently, matter and radiation separated and the opaque Universe became transparent for the first time.
At last, the photons were liberated from the labyrinth of electrons and could traverse long distances unhindered. The photons from the early Universe are still flying around today — referred to as cosmic background radiation. They constitute the faint residual glow of the Big Bang from almost 14 billion years ago — the last glimmer of a gigantic cosmic firework.
Since becoming transparent, the Universe has grown 1,100 times larger. The energy density of the cosmic background radiation decreased as the Universe's volume increased. For that reason the temperature of the background radiation reaching us today is not 3,000 K anymore but just 2.7 K. Since the Big Bang, the Universe has come fairly close to absolute zero, 0 K, or –455 °F. As it continues to expand, someday in the very far future it will reach absolute zero temperature.
The Universe's background radiation was actually discovered by chance in 1964 by the American radio astronomers Arno Penzias and Robert Wilson, although others had previously predicted its existence. The two scientists received the Nobel Prize in 1978 for their work. Another Nobel Prize was awarded to the American astrophysicists George Smoot and John Mather in 2006. Together with their team, they obtained the first precise measurements of cosmic background radiation using the space satellite COBE (Cosmic Microwave Background Explorer) and were able to determine its structure and extension in space. These and other measurements taken with the Wilkinson Microwave Anisotropy Probe (WMAP) satellite (shown in Plate 1.B) provide confirmation of the Universe having gone through an extremely hot phase when it was occupying an immensely small space — in other words, the Big Bang. The team led by Smoot and Mather was able to prove the existence of a very slight clumping of matter 380,000 years after the Big Bang, the time when the cosmic background radiation originated. Those early lumps were the condensation seeds of all later cosmic structures, in particular those of galaxies.
A few hundred million years passed before the Universe completely changed its characteristics yet again. The "dark ages" that had persisted since the atomic nuclei had begun capturing electrons came to an end. The first stars in the Universe emerged from the giant and increasingly clumpy clouds of gas. They were composed of just the hydrogen, helium, and lithium of the primordial soup left behind after the Big Bang. This way the cosmos was lit up for the very first time. The UV light emitted by these stars led to the ionization of neutral atoms in the gas clouds. The intense stellar radiation had dislodged the electrons from their atoms. The very existence of the first stars had thus altered the conditions for the formation of subsequent stars. As a result, star formation continued more efficiently. Greater and greater numbers of stars formed, and, together with the gas, they arranged themselves in huge clouds of stars known as galaxies.
In their hot interiors, the first stars synthesized chemical elements heavier than hydrogen and helium. This production of additional elements led to significant changes in the Universe yet again. From that time on, countless stars began to chemically enrich the surrounding gas in their galaxies. After about nine billion years, enough of the elements had accumulated for the formation of our Sun along with its planets in a galaxy that we call the Milky Way. Our planet Earth was made from a substantial amount of iron and other elements that had to be first synthesized in stars.
At present, after 13.8 billion years of cosmic evolution, the mass fraction of the elements from lithium to uranium is roughly 2%. When the Sun was born about 4.6 billion years ago, it was about 1.5%. Apart from any lithium, all this material was produced in stars. For this reason, stars, especially the oldest ones, are the key to understanding exactly how the chemical diversity currently present in the cosmos developed over time.
The requirements for the existence of life were met by the time the Sun formed. A human being is mostly made of water, H2O, composed of oxygen made inside stars and hydrogen from the Big Bang. An oxygen atom is about 16 times heavier than a hydrogen atom, so in a water molecule the mass ratio of hydrogen to oxygen is 1 : 8. Since our body weight comprises 65% water, this means that 8% (i.e., one-twelfth) is hydrogen. Voilà. We ourselves are part of the Big Bang as the hydrogen inside us originated from within the first minutes of the Universe. A person weighing 75 kg is thus carrying around about 6 kg of Big Bang hydrogen. Babies' water content is even higher, almost 90%, so a baby weighing 3.5 kg contains 370 grams (11%) of Big Bang hydrogen, which roughly corresponds to the weight of a full can of soda. As illustrated in Figure 1.2, we consume these and other elements every time we drink, for instance, a tasty lemonade.
The chemical elements that constitute the molecules that compose our bodies are billions of years older than the few years that have elapsed since each of us was born. So how do astronomers explore this cosmic past?
1.2 Stellar Archaeology
In the same way that archaeologists search for relics of earlier civilizations and epochs, stellar archaeology explores the early cosmos by means of old stars. Of course it is not a matter of digging in the dust or dirt somewhere in a desert under the blazing sun but instead searching the night sky for stars dating to the time shortly after the Big Bang. The main requirement is a sky survey, which corresponds to the selection of an excavation site. Sky survey data list all objects observed with a dedicated telescope in a particular region of the sky, along with their positions, brightnesses, and other characteristics, such as color.
Then begins the laborious task of digging through all the entries in those huge star catalogs with the help of computer algorithms, the excavations so to speak. Figure 1.3 illustrates this approach. At some point the astronomer finds a potentially interesting object, which is set aside for a more detailed inspection in a subsequent step of the entire selection procedure. A small or medium-sized telescope with a mirror of 2 to 4 m in diameter is needed for this task.
Then the whole procedure has to be repeated. Most stars are not interesting enough for further observation. Only the best, most promising objects are observed subsequently, but then with one of the world's largest telescopes. Still, astronomers need a bit of luck as well. In the end, only few such objects turn out to be truly important contributors to the advancement of science. But this is precisely the goal, unearthing those ancient stars.
Comprehensive surveys of the Milky Way exist to provide astronomers with plenty of data and to help them reconstruct the long evolutionary history of the Universe almost all the way back to the beginning. Plate 1.C depicts the Andromeda galaxy, our slightly more massive sister galaxy, to show what the Milky Way might look like when viewed from far away. Every new finding about the structure and evolution of the Milky Way also leads to a more complete understanding of other galaxies such as Andromeda.
As stellar archaeologists, we primarily study the chemical composition of the oldest stars found in the Milky Way. This idea is illustrated in Figure 1.4. It means that our abundance measurements of the elements in those stars help us reconstruct how the chemical elements evolved throughout cosmic history, almost as far back as the Big Bang. This approach gives us a glimpse into our home galaxy's earliest epochs and lets us draw specific conclusions about how stars and even galaxies formed and evolved in the early Universe.
In this context, astronomers use old stars to answer a broad range of fundamental questions. This work resembles archaeologists excavating the remains of a Stone Age settlement to reconstruct how and in what environment these people used to live. Stellar archaeology does exactly the same thing. From observational data, it reconstructs the characteristic features of the first gigantic supernova explosions that expelled freshly synthesized elements into their surroundings like immense fountains. Which elements did they produce and in what quantities? Can the conditions for the emergence of the earliest stars and galaxies be deduced from these results?
We have not yet completely analyzed all of the sky surveys of the past decade to systematically track down ancient stars, and new projects look even more promising. The field of stellar archaeology is thus buzzing with excitement. The Australian SkyMapper telescope and other surveys are currently producing enormous amounts of data. Such large-scale observations of the Southern Hemisphere sky are bound to produce countless discoveries of ancient stars in the Milky Way's outer region, the so-called halo. New dwarf galaxies will likely be discovered and immense strung-out stellar streams, often taking up huge sections of the sky, will be found. Plate 1.D shows the Field of Streams with various stellar streams found in the Northern Hemisphere that wrap around the Milky Way. Many of the recently discovered faint dwarf galaxies are labeled as well. All these data will help us explore even better the chemical and dynamic processes that led to the formation of stars and galaxies.
Another complementary approach to studying the early history of the Universe besides using ancient Galactic stars is observing extremely distant galaxies and gas clouds. This approach is widely used and is pretty well known to the public, partly because of the spectacular images of the farthest galaxies that, for example, the Hubble Space Telescope has been delivering since 1990. The Hubble Space Telescope can be seen in Plate 1.B, along with some of the most impressive images it produced.
Those extremely faraway gaseous objects emitted their light as young galaxies in the early phases of the cosmos. Since the speed of light is finite, it took billions of years for their light to finally reach us. This method offers a way to directly examine the past. We thus know that at least some stars already existed about 700 million years after the Big Bang. However, unlike stellar archaeology, this technique can provide only a limited amount of detailed knowledge about the chemical composition of the earliest stars formed and about the production of the chemical elements in their interiors.
Before we devote ourselves further to chemical evolution and the history of our Milky Way, let us first glance at the historical course of research about stars, their luminosities, and their element synthesis.
(Continues...)
Excerpted from Searching for the Oldest Stars by Anna Frebel, Ann M. Hentschel. Copyright © 2015 Princeton University Press. Excerpted by permission of PRINCETON UNIVERSITY PRESS.
All rights reserved. No part of this excerpt may be reproduced or reprinted without permission in writing from the publisher.
Excerpts are provided by Dial-A-Book Inc. solely for the personal use of visitors to this web site.
Table of Contents
Preface xiAn Introductory Remark xv
1 What Is Stellar Archaeology? 1
1.1 The First Minutes after the Big Bang 2
1.2 Stellar Archaeology 7
2 Two Centuries of Pursuing Stars 10
2.1 First Glimpses of Stellar Rainbows 11
2.2 Decoding Starlight 16
2.3 A New Perspective of the Cosmos 22
2.4 Looking into the Hearts of Stars 29
2.5 Modern Alchemy 35
2.6 The Foundation of Cosmology 38
2.7 The Origin of the Elements 44
3 S tars, Stars, More Stars 51
3.1 The Cycle of Matter in the Universe 51
3.2 Astronomers and Their Metals 55
3.3 Element Nucleosynthesis in the Cosmic Kitchen 59
3.4 Stellar Diversity 73
4 Stellar Evolution—From Birth to Death 78
4.1 Sorting Stars 78
4.2 A Protostar Forms 85
4.3 The Evolution of a Low-Mass Star 88
4.4 The Evolution of a Massive Star 93
4.5 Supernovae and Supernova Remnants 95
4.6 Preliminary Thoughts about Working with Metal-Poor Stars 102
5 Neutron-Capture Processes and the Heaviest Elements 107
5.1 How Neon Lamps Relate to Giant Stars—Element Synthesis in the S-Process 109
5.2 Thorium, Uranium, and R-Process Element Synthesis 114
5.3 Cosmo-Chronometry: The Oldest Stars 120
5.4 Nuclear Astrophysics 128
6 Welcome to Our Milky Way 130
6.1 A Milky Way above Us 130
6.2 The Milky Way’s Structure 133
6.3 Dwarf Galaxies 139
6.4 Star Clusters 145
6.5 Naming Stars 151
7 Tales Told by Light 155
7.1 A Little Lexicon of Light 156
7.2 Spectroscopy—Deciphering Starlight 160
7.3 Element Abundance Analyses of Stars 166
7.4 The Largest Telescopes in the World 173
7.5 Three Steps toward Success 180
7.6 Observations with MIKE 186
8 Let’s Go Ob serve Some Stars! 192
8.1 Going Stargazing 192
8.2 Good-Weather Beer 197
8.3 A Sunset 198
8.4 The Observa-thon 201
8.5 One Hundred and Five Stars per Night 203
8.6 Computers, Computers . . . 205
8.7 Tested by Fire 207
9 The Chemical Evolution of the Early Universe 210
9.1 The First Stars in the Universe 210
9.2 The Family of Metal-Poor Stars 217
9.3 The Most Iron-Poor Stars 222
9.4 The Cosmic Chemical Evolution 227
10 Finding the Oldest Stars 241
10.1 Pursuing Metal-Poor Stars 241
10.2 Bright Metal-Poor Stars 248
10.3 Mt. Stromlo Succumbs to Bushfires 252
10.4 The Discovery of a Record-Breaking Most Iron-Poor Star 257
10.5 The Astronomical Community 264
11 A t the End of a Cosmic Journey 267
11.1 Cosmological Simulations 267
11.2 Where Do Metal-Poor Stars Come From? 273
11.3 Expectations of Future Surveys 279
11.4 The Next Generation of Giant Telescopes 282
11.5 Little Diamonds in the Sky 285
Further Reading 287
Index 289
What People are Saying About This
"Frebel accessibly describes the cutting-edge archaeological search in our cosmic backyard—the halo of the Milky Way galaxy—for relics from the first generation of stars born shortly after the big bang. This wonderfully written book captures the scientific excitement of research in this field by one of its pioneers."—Avi Loeb, Harvard University"Anna Frebel is the Indiana Jones of astronomy. In the search for the oldest stars, her cosmic fossils, she and her colleagues are revealing the very evolution of our universe. This book offers a thorough and detailed summary of those stellar explorations — how stars are born and die, how they generate the chemical elements — but also a fascinating portrait of how science gets done."—Marcia Bartusiak, author of The Day We Found the Universe and Black Hole"This authoritative and accessible book is a delightful read. Covering wide swathes of the subject, Frebel provides readers with insights into what it is like to be a research astronomer today. I am thrilled to see the recognition given to the work done by women astronomers."—Jocelyn Bell Burnell, University of Oxford"I loved reading these great stories by a master of the subject. Frebel tells them as simply as possible but no simpler—to paraphrase Einstein—and peppers her narrative with her own adventures and those of other pioneer women astronomers. There's plenty here to interest professional astronomers as well as nonspecialists."—John Mather, winner of the 2006 Nobel Prize in Physics"This is a beautiful book. Frebel describes, firsthand, the hunt for the first stars in the universe and the genuine thrill felt when working at the frontier of human knowledge. She weaves an engaging story of discovery."—Volker Bromm, University of Texas at Austin"Frebel provides an entertaining introduction to stellar astrophysics and the hunt for the oldest stars. Blending hard science with an account of her own experiences as one of the leading scientists in stellar archaeology, she manages to discuss complex phenomena in an intuitive way that nonspecialists can understand. Readers will enjoy this book without having an extended background in astrophysics."—Ralf S. Klessen, Heidelberg University