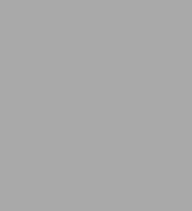
Revolutions in Differential Equations: Exploring ODEs with Modern Technology
100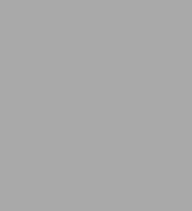
Revolutions in Differential Equations: Exploring ODEs with Modern Technology
100Paperback
-
SHIP THIS ITEMTemporarily Out of Stock Online
-
PICK UP IN STORECheck Availability at Nearby Stores
Available within 2 business hours
Related collections and offers
Overview
Product Details
ISBN-13: | 9780883851609 |
---|---|
Publisher: | Mathematical Association of America |
Publication date: | 08/01/1999 |
Series: | Notes Series , #50 |
Pages: | 100 |
Product dimensions: | 8.40(w) x 10.90(h) x 0.30(d) |
Read an Excerpt
Modeling and Visualization in the Introductory ODE Course
Robert L.
Borrelli
Courtney S. Coleman
Harvey Mudd College
Our increasingly technological society has welcomed computers with open arms.
No longer the domain of the esoteric few, powerful and (relatively) inexpensive
platforms are being marketed as a panacea for all that ails the civilized world.
Software packages are being churned out by armies of programmers whose genius
has produced code that not only addresses current societal needs, but also opens
doors for more technological change. There are good reasons for this: Most
science and engineering courses in the undergraduate curriculum have been around
so long that their content and method of approach are pretty well defined and
the supporting textbooks reflect this fact. It's in the nature of academia to be
slow to change- we aren't trying to find a niche in a competitive market.
Nevertheless, there are some very encouraging signs over the past decade that
academia is responding to the challenge of using computers in the curriculum in
an effective and creative way.
Reform movements in calculus, linear
algebra and differential equations are well under way, and all of them make good
use of hands-on projects in connection with the modeling and visualization
capabilities that technology provides. However these reform movements ultimately
turn out in the twenty-first century, one thing is already clear; modeling and
visualization will be in these courses in one way or another for a long time to
come.
What is modeling anyway? Scientists and engineers generally use
the term to describe the process of translating a natural system info a form
called a model that can be dealt with in a way that we have confidence in. the
models that interest us here are mathematical models which involve ordinary
differential equations.
Curricular reform these days seems to be
edging closer and closer to an interdisciplinary approach in which students take
a more active role in their own course work. In mathematics departments this
approach usually involves laboratory-based activities in which students play a
hands-on role in converting "word problems" into mathematical models and
"solving" them. We will describe modeling activities in which are suitable for a
course in differential equations.
1. The First Course in Differential Equations
Trends in the
undergraduate math curriculum have been influenced by a variety of factors.
First and foremost is the ready availability of powerful platforms and excellent
software for both numerical and symbolic computations. The reform movements in
all disciplines have been affected by these technology twins, but the
undergraduate mathematics curriculum has arguably been affected the most. The
primary use of technology in instruction is to visualize mathematical concepts,
solutions of equations, etc., in the context of a laboratory environment. The
first course in ODEs seems to be an ideal place to use computers for modeling
and visualization.
We have put in more years than we care to remember
in redesigning the first course in ODEs at Harvey Mudd College to bring in
modeling and visualization as an essential component of the course. When we
first embarked on this path in the late '70's, we couldn't find any solvers
which were both robust and easy-to-use, or any affordable platforms on which the
solvers could run. So our first task was to design our own solver package that
cold be used reliably on available platforms in a dynamical systems lab
environment. With the collaboration of four of our students (Ned Freed, Dan
Newman, Kevin Carosso and Tony Leneis), we finally after considerable effort,
produced an ODE solver package which was suitable for our needs, and the
National Science Foundation helped us to set up a lab dedicated to this course.
At last we were in business, or so we thought.
As is often the case at
universities and colleges with engineering programs, our first course in ODEs is
a required 3-credit-hour sophomore course with no time set aside for laboratory
instruction. The traditional syllabus for the course was designed to serve the
needs of our client disciplines. There was little flexibility in the course
syllabus and no chance the college would give us an additional credit-hour to
set up a companion laboratory course, so what to do? Well, first we made our
solver absolutely transparent to use (no mean feat) so that students could use
it on their own from the get-go with very little instruction required. Our
solver is network accessible, and so we included drivers for most graphics
platforms. That way students could access the solver from their dorm rooms (or
anywhere really) and use their favorite platforms as a front end; X-windows,
PCs, MACs, etc. Printing on an y academic laser printer could also be done over
the network. Graphs are automatically imprinted with the user name, the date,
and the time so that they can be retrieved at any time. Next, we created a
collection of computer experiments (later published as DE Lab Workbook) which
were more-or-less self-contained and designed to go along with a standard ODE
course. We assigned one (or more) experiments per week from this collection that
the students could do as "homework". In fact, each experiment replaces one
of the homework sets that otherwise would have been done that week. This slight
syllabus change was necessary because students would be quick to see that we
were cramming a four-credit hour course into three-credit
hours.
Students use any solver they like for laboratory assignments. At
the first lecture we distribute a hand-out describing all the currently
available ODE solvers with instructions on access from the various
colleges computer labs. This arrangement works well for a required course that
anyone in the department may be assigned to teach; individual instructions are
free to select any textbook/solver combination.
In contrast to the
reform calculus experience, there has been little doubt about the direction in
which the introductory ODE course should change; the only details to be worked
out concern the resources which support different modes of instruction. From
1992 to 1997 the NSF-funded Consortium for ODE Experiments (CODEE) has published
a newsletter with information about incorporating hands-on projects
("experiments") in an ODE course. Experiments always involve a modeling
component and are designed to address some questions in the modeling
environment.
2. ODE Solvers
There are a number of excellent ODE software packages
that support modeling and visualization in a first course in ODEs. Some are
commercially distributed, and some are shareware. Some are built to run as a
component of a large multi-purpose package, and some are stand-alone ODE
packages. The stand-alone ODE packages are usually designed to run on only one
platform, whereas the multi-purpose packages run on all platforms.
Here are brief descriptions of three solver packages that differ in their approach but are designed to provide a lab experience to go along with an ODE course.
Interactive Differential Equations (IDE): A collection of interactive tools
designed to explore a single concept or application in an ODE course, such as
the logistic equation, direction fields, oscillators, numerical methods, the
phase plane, eigenvectors. Laplace transforms, series solutions, chaos,
bifurcations, and many other topics, each with linked animations of the systems
being modeled. An important feature of the package is that the tools demonstrate
visually the connections between real world phenomena and the mathematical
models that describe them.
There are 97 interactive illustrations,
called tools, arranged into 31 lab collections. The intuitive point-and-click
interface allows the user to interact with the tools by setting initial
conditions and using sliders to very parameters for both linear and nonlinear
models. Each set of tools is accompanied by a workbook lab consisting of
background,, instructions, and experiments, with space for writing answers. An
instructor's manual is available with sample answers filled in.
These
tools were developed by a team of mathematics faculty including John Cantwell,
jean Marie McDill, Steven Strogatz, and Beverly West; the software designer, who
originated the package was Hubert Hohn. The package is now available for Windows
95 as well as for the Macintosh.
Internet Differential
Equations Activities (IDEA):
This product can be thought of as an
interactive virtual lab book for differential equations at the undergraduate
level. IDEA has the basic goal of developing and disseminating software for
numerical explorations of mathematical models using differential equations.
These materials are available over the Web and can be used by anyone with
connections to the Internet. The IDEA developers, Tom LoFaro and Kevin
cooper, have created tools to assist instructors in the development of
their own Web materials and/or contribute to the IDEA site. Currently, most
explorations are based on biology, chemistry, and ecology. The IDEA approach
provides resources that can give students an appreciate for research projects
involving differential equations not found in traditional texts.
ODE Architect: With NSF/DUE support, the CODEE consortium John Wiley &
Sons and the software house Intellipro are producing an interactive multimedia
ODE package which is built over a robust solver engine designed by Larry
Shampine. Scheduled for release in 1998 the CD-ROM runs on a PC under Windows
3.2 or better and is accompanied by a lab workbook or experiments. With video,
sound, animation, and dynamic graphics, this interactive package provides
motivation for modeling, analysis, visualization, discovery, and interpretation
in any ODE lab environment. In addition to the solver tool there are 13
interactive modeling modules on ODEs and dynamical systems, as well as a library
of ODEs with their dynamically generated solution curves and orbits. Each module
leads the user through a model building process via several exploration screens,
and ends up with questions. These questions take the user to the solver tools
and to the accompanying lab workbook where the user is asked to carry out
graphics-based experiments to explain what is going on. Users can enter their
own ODEs and explore dynamical systems with 2D or 3D graphics or numerical
tables by seeing what happens when data and parameters change. Graphs are
editable and aces can be scaled and labeled, equidistant-in-tiume orbital points
marked. Graphs of solutions and orbits can also be colorized, animated,
displayed in various line styles, overlayed with graphs of functions, and
graphed together with solution curves of other ODEs. All of this is possible
with no programming or special commands to remember. ODE Architect has a report
writing feature; graphs can be cut and pasted into reports.
3. Laboratory Experiments for an ODE Course
We can use
technology to examine dynamical processes and their ODE models that would have
been inaccessible only a few years ago. Hands-on experience in the setting of an
ODE laboratory is at the heart of this approach. The "laboratory" might be a
room with computers or individuals at home or in a dormitory working with their
own computer, or even lecture demos. Whatever the mode, here are some central
ideas that come up in the dynamical systems laboratory.
Modeling
* Derivative as a rate of change
* Balance law: net
rate of change=rate in-rate out
* Compartmental
models
* Newton's force laws
* Circuit laws for voltages and
currents
* Chemical law of Mass Action
Visualization
* Derivative as slope of a curve
* The art of
making graphs that tell a story
* Effective use of computer graphics;
choosing appropriate displays
* How to interpret graphs; extracting
information from graphs
* Change scales, time span, viewpoint to get the
most informative graph
Solution Behavior
* Do we use theory, formulas or computer simulation
to study solution behavior?
* Do solutions tend to an equilibrium state
or periodic solution with advancing time? Or do they become
chaotic?
* How sensitive are solutions to changes in data and system
parameters?
* Is analysis of the sign of a rate function useful to see
how solutions behave?
* What happens to long-term solution behavior as a
parameter changes?
* If the modeling system is nonlinear, can it be
approximated by a linear system, or is the behavior due to the
nonlinearities?
Computer Techniques
* Should the variable be scaled before
computing?
* Scale out system parameters not relevant to the sensitivity
study
* Does your solve handle on-off functions of engineering, or can
you work around it?
* Is the solution behavior generated by your
numerical solver really there, or is it an artifact of the numerics? Has your
solver overlooked an important aspect of solution behavior because its internal
settings are inappropriate?
Well that's a long list of things to keep in mind, but the numerical experiments that follow touch on many of these points. Don't look for the standard experiments and models that can be found in many places. Our aim is to present challenging models that can now be handled by students in an introductory ODE course if they have access to a decent numerical solver.