Radioactive Releases in the Environment: Impact and Assessment / Edition 1 available in Hardcover
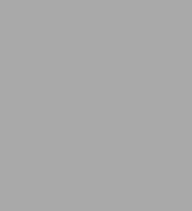
Radioactive Releases in the Environment: Impact and Assessment / Edition 1
- ISBN-10:
- 0471899232
- ISBN-13:
- 9780471899235
- Pub. Date:
- 05/07/2003
- Publisher:
- Wiley
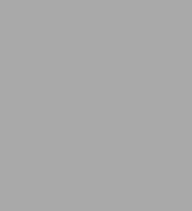
Radioactive Releases in the Environment: Impact and Assessment / Edition 1
Hardcover
Buy New
$358.95Overview
Product Details
ISBN-13: | 9780471899235 |
---|---|
Publisher: | Wiley |
Publication date: | 05/07/2003 |
Pages: | 492 |
Product dimensions: | 6.85(w) x 9.80(h) x 1.30(d) |
About the Author
Keith Randle is the author of Radioactive Releases in the Environment: Impact and Assessment, published by Wiley.
Ranjeet S. Sokhi is the author of Radioactive Releases in the Environment: Impact and Assessment, published by Wiley.
Read an Excerpt
Radioactive Releases in the Environment
Impact and AssessmentBy John R. Cooper Keith Randle Ranjeet S. Sokhi
John Wiley & Sons
ISBN: 0-471-89924-0Chapter One
Introduction to Environmental RadioactivitySeveral modern industries, particularly in the area of power production and medicine, involve radiation and may discharge radioactive materials to the environment. This present book is about the effects of these discharges and the basis for their control. This chapter sets the scene by briefly describing the history of the discovery of radiation, its uses and the radiation exposures that we may all experience. It also provides a 'route map' for the rest of the book.
1.1 THE DISCOVERY AND EARLY HISTORY OF RADIOACTIVITY
In 1895, a German physicist, Wilhelm Röntgen, made an epoch-making discovery. While studying the cathode rays formed by electrical discharges through tubes containing a rarefied gas, he noticed that across the room a screen coated with barium platinocyanide gently glowed. The rays responsible for this phenomenon were quickly shown to pass through materials opaque to ordinary light. Importantly, the rays, now called X-rays, would expose a photographic plate, enabling, for example, photographs of bone fractures to be made.
This discovery provoked a wave of scientific and public interest. One scientist who was excited by the discovery was Antoine Henri Becquerel, a French physicist. He had been investigatingfluorescence and phosphorescence and thought that fluorescing substances might produce X-rays. He used potassium uranyl sulfate in his experiments because these crystals were unaffected by exposure to air. Photographic plates were wrapped in black paper with the crystals being placed on top. The entire device was then exposed to bright sunlight for several hours before the photographic plate was developed. The photographic plate showed a faint outline of the crystals, which Becquerel ascribed to rays that were emitted during fluorescence of the crystals. Becquerel attempted to repeat these experiments but the weather was overcast and he placed the photographic plates together with the crystals away in a drawer after only a brief exposure to sunlight. The weather remained overcast during the following days and eventually Becquerel developed the plates expecting to see only a very faint image. He was surprised to see that the image was dark and well defined - rays were being emitted from the crystals without any prior exposure to sunlight! Becquerel quickly showed that the phenomenon was related to the uranium content of the crystals. The uranium emitted penetrating radiation continuously and without any form of initiation - the phenomenon of radioactivity had been discovered but it prompted little immediate interest (further details of Becquerel's work are given in Allisy, 1996).
One person who was interested was Marie Curie; she discovered that another heavy element, thorium, also emitted radiation. Early on, together with her husband Pierre, Marie Curie discovered that pitchblende and the mineral chalcocite emitted more radiation than could be accounted for by their uranium content. Further investigations on pitchblende by the Curies led to the identification of two other radioactive elements, i.e. polonium and radium. The significance of these discoveries was quickly recognized and in 1903 the Curies shared the Nobel Prize for physics with Becquerel for their work on radioactivity. Marie Curie was later awarded the Nobel Prize for chemistry for her work on radium and polonium - she is one of the few people to have been awarded two Nobel Prizes. Becquerel gave his name to the unit of radioactivity - the becquerel, symbol Bq (one becquerel is one nuclear disintegration per second).
Not only had the phenomenon of radioactivity been discovered but it could be said that environmental radioactivity had also been discovered. Uranium, thorium, polonium and radium are all naturally occurring elements. Work on radioactivity developed and expanded rapidly following the early discoveries of Becquerel and the Curies. In 1899, Rutherford showed that one type of radiation comprised positively charged particles and these were later identified as helium nuclei (alpha particles). In 1900, the French scientist Villard characterized another type of radiation - the uncharged, penetrating gamma rays. The laws of radioactive decay were established by 1903 by Rutherford and Soddy, and by 1912 the concept of isotopes had been developed to explain why radioelements exist with more than one atomic weight. The first artificial radioactive isotopes were produced in 1934.
Radioactivity decay and the decay laws are explained in detail in Chapter 2. For the moment, it is important to realize that atoms of the same element may have different atomic weights and some types of atoms are unstable. An unstable atom can transform spontaneously into an atom of another element and, in so doing, emit radiation. As this process involves the transformation of atoms, the number of the original atoms must decrease with time. The time taken for the number to fall by half is called the half-life. It is a unique and unalterable property of each radionuclide. Half-lives can range from fractions of a second to millions of years.
The three main types of ionizing radiation were quickly identified as alpha particles (two protons plus two neutrons), beta particles (a negatively charged electron), and gamma rays (uncharged, energetic photons). The characteristics of ionizing radiation are described in Chapter 2. It should be emphasized that this book is about ionizing radiation, namely radiation of sufficient energy to displace electrons from atoms or molecules and so produce ions (see Chapter 3). Ionizing radiation should be distinguished from non-ionizing radiation, e.g. UV light and radio waves, neither of which have enough energy to cause ionization. In this book, the word 'radiation' always refers to ionizing radiation.
The possible beneficial effects of radiation were quickly exploited. Radioactivity was soon recognized as a more concentrated form of energy than had been hitherto known. Radium was used in the treatment of solid cancers where the radiation often destroyed the tumour tissue. It became to be viewed as an almost miraculous substance and at one time it was more valuable than gold.
Fascination with radium and radioactivity lead to a plethora of 'quack uses', including belts, pads, face creams, etc., all of which were claimed to have therapeutic properties. One of the most significant legitimate uses was in the production of luminous dials - a use that continued until fairly recently. In the early days, the dials were often painted by young women who licked the brushes to obtain a fine point; consequently they took some of the radioactive material into their bodies!
The fascination with radioactivity was tempered when it slowly became clear that it could have fatal effects. Over a number of years some people who had worked with radioactive materials experienced ill health and died. Sufferers included some of the radium dial painters. In July 1934, at the age of 66, Madame Curie died of a blood disorder that was almost certainly brought on by her work with radioactivity. Recognition of possible harmful effects led, in 1928, to the setting up of the International X-ray and Radium Protection Committee that was to advise on standards of protection (see Chapter 4 for a discussion of the biological effects of ionizing radiation and of radiological protection).
Nevertheless, we live in an environment pervaded by radiation of natural origin. Artificial uses of radioactivity can bring about many benefits, ranging from nuclear medicine to the production of electricity by nuclear power. It is the purpose of this book to present the reader with a balanced view of the sources of radiation exposure, the behaviour of radionuclides in the environment, the effects on man and the basic principles of control. The remainder of this chapter gives an overview of the sources of radiation exposure; many of the topics covered are amplified in later chapters.
1.2 SOURCES OF RADIATION
We cannot sense radiation but it is a continual feature of human existence. Natural radiation sources are the most significant contributors to exposure for the vast majority of individuals.
1.2.1 Natural Sources of Radiation
Studies since the early 1900s have characterized the natural radiation environment. Historical reviews are contained in Kathren (1984) and Glasstone (1979). On Earth, we are exposed to ionizing radiation of both terrestrial and extra-terrestrial origins. Radiation from outside the Earth's atmosphere is called cosmic radiation and is described first, followed by other natural sources.
Cosmic radiation
During the first decade of the twentieth century, experiments suggested a hitherto unknown source of radiation that was thought to be entirely gamma radiation from terrestrial sources. An Austrian physicist, V F Hess, was investigating the attenuation of terrestrial gamma radiation by the atmosphere and conducted experiments with radiation detectors in balloons; he found, to his surprise, that his instruments registered increasing radiation levels with increasing altitude. He concluded, in 1912, that penetrating radiation was coming from extraterrestrial sources: cosmic radiation had been discovered.
Cosmic radiation has two main sources, i.e. galactic and solar. Before they interact with the Earth's atmosphere, both types of radiation consist mainly of protons together with some helium and other heavier ions. Galactic cosmic rays are produced outside of the solar system possibly because of stellar flares, supernova explosions, etc. However, there is no generally accepted theory as to their generation. The energy of galactic cosmic rays averages about [10.sup.4] MeV, with maximum energies of up to around [10.sup.14] MeV.
The other origin of cosmic radiation is the Sun. Particles of low energy are emitted continuously by the Sun but more energetic ones are emitted during solar events such as magnetic disturbances or flares. Thus, emission of solar cosmic radiation tends to follow the 11 year solar cycle with a maximum during increased solar activity and a minimum in the 'quiet' period.
Solar cosmic rays are usually of considerably lower energy than those of galactic origin and energies are typically between 1 and 100 MeV. They are of less significance at the Earth's surface because most have insufficient energy to penetrate the Earth's magnetic field.
On entering the atmosphere, energetic particles, primarily protons, may interact with nuclei of atmospheric gases (nitrogen, oxygen and argon) in a variety of nuclear reactions. The products include neutrons, protons, muons, pions and kaons, together with radioisotopes such as [sup.3]H (tritium) and [sup.7]Be (see cosmogenic radionuclides, p. 11 below). The secondary particles may be energetic enough to initiate further reactions leading to a cascade of events. Cosmic radiation at the Earth's surface comprises directly ionizing secondary particles, together with a small neutron component. Exposure to cosmic rays increases with altitude and hence air travel increases exposure to cosmic radiation. There is also a relatively smaller variation with latitude - the Earth's magnetic field causes a greater flux of low-energy protons to reach the top of the atmosphere at the poles than at equatorial regions.
Terrestrial sources of radiation
The work of the Curies showed that radioactive materials existed naturally in the environment. Natural radionuclides can be divided into two groups depending on their origin. The first and the most important group is the primordial radionuclides. These have been present since the origin of the Earth. The second group is the cosmogenic radionuclides. They are continuously produced in the upper atmosphere by the action of cosmic rays. Concentrations of primordial and cosmogenic radionuclides in environmental materials and foodstuffs are given in Tables 1.1 to 1.5. The information is taken from two published reviews by Bradley (1993) and The United Nations Scientific Committee on the Effects of Atomic Radiation (UNSCEAR, 2000a).
Primordial radionuclides This group of radionuclides is responsible for most of the radiation exposure of the vast majority of individuals. The principal radionuclides are [sup.40]K (half-life, 1.28 x [10.sup.9] years), [sup.232]Th (half-life, 1.41 x [10.sup.10] years) and [sup.238]U (half-life, 4.47 x [10.sup.9] years). There are two other primordial radionuclides of lesser importance for human exposure, i.e. [sup.87]Rb (half-life, 4.7 x [10.sup.10] years) and [sup.235]U (half-life, 7.04 x [10.sup.8] years). The uranium and thorium radionuclides are the starting points for decay chains of several radionuclides. Members of these decay chains may be important in their own right, either as contributors to human exposure or for other radioactive properties. The decay chains of [sup.238]U, [sup.232]Th and [sup.235]U are shown in Appendix 3. The general properties of decay chains are discussed in Chapter 2, but for the moment it is sufficient to understand that a radioactive isotope decays to another and that one to another, etc., until a stable isotope is reached. The two decay series headed by [sup.238]U and [sup.232]Th are the most important, while the series headed by [sup.235]U is of lesser importance. The primordial radionuclides are listed in Table 1.6.
A primordial radionuclide that causes widespread and inescapable exposure but not necessarily the highest is the beta/gamma emitter, [sup.40]K. This is present in natural potassium in an effectively fixed proportion of 0.012%. Potassium is a biologically essential element and its body concentration is under homeostatic control; thus, exposure to [sup.40]K is unavoidable but does vary with age and sex in line with corresponding changes in the concentration of potassium. The presence of [sup.40]K in rocks and soils is also a source of external exposure. Concentrations of [sup.40]K in soils range from zero for some soils in the Scottish islands to over 3200 Bq [kg.sup.-1] dry weight, again in the Scottish islands. UNSCEAR estimates the worldwide average concentration of [sup.40]K in soil at 420Bq [kg.sup.-1] (UNSCEAR, 2000a). Concentrations in rocks are less well studied but appear to be lower: concentrations of between 4 and 40 Bq [kg.sup.-1] have been reported for phosphate-bearing rocks.
The decay chain headed by [sup.238]U is important. [sup.238]U is a ubiquitous radionuclide. It is present in most environmental materials and it has been widely studied in rocks and soils in connection with uranium prospecting. Levels of [sup.238]U are generally in the range of 2 to 300 Bq [kg.sup.-1] dry soil.
Continues...
Excerpted from Radioactive Releases in the Environment by John R. Cooper Keith Randle Ranjeet S. Sokhi Excerpted by permission.
All rights reserved. No part of this excerpt may be reproduced or reprinted without permission in writing from the publisher.
Excerpts are provided by Dial-A-Book Inc. solely for the personal use of visitors to this web site.
Table of Contents
Preface.1. INTRODUCTION TO ENVIRONMENTAL RADIOACTIVITY.
2. NATURE OF RADIOACTIVITY.
3. INTERACTION OF RADIATION WITH MATTER.
4. BIOLOGICAL EFFECTS OF IONIZING RADIATION AND RADIOLOGICAL PROTECTION.
5. MANAGEMENT OF RADIOACTIVE WASTE.
6. NUCLEAR POWER.
7. RELEASES OF RADIONUCLIDES FROM NON-NUCLEAR POWER INDUSTRIES.
8. INSTRUMENTATION FOR RADIATION DETECTION AND MEASUREMENT.
9. MEASUREMENT TECHNIQUES AND PROCEDURES.
10. SAMPLING AND SAMPLE PREPARATION.
11. STATISTICAL TREATMENT OF RADIOACTIVITY MEASUREMENTS.
12. RADIOACTIVE SURVEYING AND REMOTE SENSING.
13. MODELLING THE DISPERSION OF RADIONUCLIDES IN THE ENVIRONMENT: AN INTRODUCTION TO MODELLING CONCEPTS.
14. MODELLING DISPERSION OF RADIONUCLIDES IN THE ATMOSPHERE.
15. MODELLING DISPERSION OF RADIONUCLIDES IN AQUATIC AND TERRESTRIAL ENVIRONMENTS.
16. ASSESSMENT OF RADIATION DOSES.
APPENDIX 1. Acronyms Used in the Text.
APPENDIX 2. Units, Terms and Conversion Factors for Radiation, Radioactivity and Related Areas.
APPENDIX 3. Data for the Most Important Environmental Radionuclides.
Bibliography.
References.
Index.