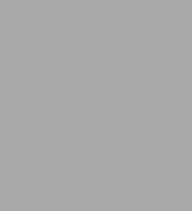
Primer of Genetic Analysis: A Problems Approach
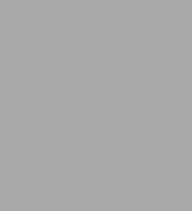
Primer of Genetic Analysis: A Problems Approach
eBookRevised (Revised)
Available on Compatible NOOK devices, the free NOOK App and in My Digital Library.
Related collections and offers
Overview
Product Details
ISBN-13: | 9781107086159 |
---|---|
Publisher: | Cambridge University Press |
Publication date: | 10/01/2007 |
Sold by: | Barnes & Noble |
Format: | eBook |
File size: | 21 MB |
Note: | This product may take a few minutes to download. |
About the Author
Jenna J. Hellack is Professor and Chairperson of the Department of Biology at the University of Central Oklahoma. She currently teaches introductory genetics, evolution, and molecular and population genetics courses. Her research interests are in the area of population genetics.
David S. Durica has been a member of the Department of Zoology at the University of Oklahoma since 1988, where he teaches both undergraduate and graduate courses in genetics. His research focuses on developmental genetics and the organization and expression of multigene families.
Read an Excerpt
Cambridge University Press
978-0-521-84268-6 - Primer of Genetic Analysis - A Problems Approach - by James N. Thompson, Jenna J. Hellack, Gerald Braver and David S. Durica
Excerpt
CHAPTER ONE
Overview of Genetic Organization and Scale
The genetic material is a molecule called deoxyribonucleic acid (DNA). Each chromosome contains a single long strand of DNA that encodes the information needed to produce hundreds or even thousands of different proteins. Each species has a characteristic array of chromosomes that carries all the genes needed to produce that organism from a single cell. The relationship between the genetic makeup of an organism (the genotype) and the developmental effects of these genes (the phenotype) can be complex. It is, therefore, useful to begin with a simple overview of these processes. Here we introduce some of the key concepts of genetics using an illustrated guide that begins at the smallest unit of genetic organization within a nucleus and ends at the level of the population. Some important terms are shown in boldface type, and definitions are given in the Glossary.
■ DNA is made up of subunits called nucleotides composed of a sugar (S), a phosphate group (P), and a nitrogenous base (B). There are four nucleotides that differ by the nucleotide base they contain:adenine (A), guanine (G), thymine (T), and cytosine (C). Genetic information is encoded in DNA by the sequence of these four bases.
Image not available in HTML version |
nucleotide
■ Nucleotides are linked by a bond between the sugar of one nucleotide and the phosphate group of the next.
Image not available in HTML version |
a portion of one strand
■ This produces a long chain that can be literally millions of nucleotides long.
Image not available in HTML version |
two nucleotides (schematic representation)
■ A single DNA molecule is composed of two such strands that join together by bonds between the nucleotide bases (A paired with T, and C paired with G). This forms a DNA double helix.
Image not available in HTML version |
schematic of DNA and double helix
■ The DNA is bound to structural proteins (histones that make up the nucleosome) that help pack the DNA in the nucleus and to regulatory proteins that turn genes on and off during development.
Image not available in HTML version |
nucleosomes with DNA
■ Each chromosome is made up of one long DNA molecule (one DNA double helix) and its associated proteins. The centromere is the attachment site for the spindle fiber that moves the chromosome during cell division.
Image not available in HTML version |
■ Along the length of this DNA molecule are the regions that code for the production of proteins. These regions (or genetic loci) are the genes. Each gene can be up to a thousand or more nucleotides long, and every chromosome carries as many as several thousand different genes. All of the genes on a given type of chromosome are thus linked on a single DNA molecule. This linked group of genes is called a linkage group. The linear order of genes on a chromosome can be mapped to produce a linkage map.
Image not available in HTML version |
chromosome showing a map of five genes in linear order on its linkage group
■ The body cells (somatic cells) of most organisms contain two copies of each type of chromosome (diploid). These are the homologous chromosomes. Since homologous chromosomes carry the same series of genes, they are members of the same linkage group. They can, however, differ in the form that a given gene takes (that is, normal or mutant). The different forms of a gene are called alleles.
Image not available in HTML version |
two homologous chromosomes carrying alleles A and a
■ When the nucleus prepares to divide, each DNA molecule replicates except for the centromere. This yields two identical copies of the DNA molecule bound at the centromere. At this stage, the two copies are called sister chromatids. Since they have not yet divided at the centromere, however, each unit is still considered a single chromosome.
Image not available in HTML version |
two homologous chromosomes, each with two sister chromatids
■ Chromosomes that carry different sets of genes are called nonhomologous chromosomes. Every species has its own characteristic number of different chromo-somes (n). The total number of chromosomes in a somatic cell is, therefore, 2n. All the genes needed to produce that organism will be found somewhere on one of these n linkage groups. The total 2n genetic makeup is the genome. In the figure of the hypothetical cell, there are three pairs of nonhomologous chromosomes in the genome.
Image not available in HTML version |
hypothetical cell with 2n = 6, as seen during nuclear division
■ Mitosis is a type of nuclear division that yields two identical diploid cells (2n). Meiosis is a special type of nuclear division found in reproductive (germinal) tissue that yields gametes. Each gamete carries only one copy of each linkage group and has a haploid (n) number of chromosomes. The diploid chromosome number is re-created at fertilization when the haploid maternal set and the haploid paternal set fuse.
Image not available in HTML version |
mitosis and meiosis
■ If we focus our attention on one gene, the alleles on the two homologous chromo-somes can either be the same (AA or aa = homo-zygous genotypes) or be different (Aa = hetero-zygous genotype). These separate (segregate) during meiosis to produce haploid gametes.
Image not available in HTML version |
branching diagram to show haploid products
■ The products of segregation and fertilization are highly predictable, giving rise to the basic rules of genetic transmission. Gregor Mendel set the foundation for this area of genetics.
Image not available in HTML version |
Mendelian cross using Punnett square for two heterozygous parents
■ A pedigree diagram shows genetic relationships from a series of different Mendelian crosses. Circles indicate females and squares indicate males.
Image not available in HTML version |
a simple pedigree
■ Most genes code for the production of proteins. One of the two strands (the template strand) of a DNA molecule is “read” (through transcription) to yield a molecule of messenger RNA (mRNA). This then binds with ribosomes, where it defines the sequence of amino acids needed to produce the correct polypeptide (protein). This is translation.
Image not available in HTML version |
DNA → mRNA → protein
■ Many proteins are enzymes, which catalyze specific biochemical steps. Thus, genes work by controlling the biochemical activities for growth and function of cells. In this way, the genome codes for all of the morphological, physiological, and behavioral characteristics (phenotypes) of an animal or plant.
Image not available in HTML version |
biochemical pathway
■ Some characteristics are the result of several genes and environmental factors working together. Their expression is measured on an appropriate scale (such as height in meters). These are quantitative traits (multifactorial or polygenic traits).
Image not available in HTML version |
■ The genetic makeup of an individual is the genome, whereas the total genetic makeup of all individuals in the population is the gene pool. The gene pool is described in terms of allele frequencies, where p is the frequency of the A allele and q is the frequency of the a allele. By using appropriate assumptions, the genetic makeup of individuals in the population can be predicted.
Image not available in HTML version |
alleles in a hypothetical gene pool
■ Hardy, Weinberg, and Castle established the foundation for population genetics by showing that allele frequencies remain in equilibrium unless acted upon by selection, migration, mutation, sampling error in small populations, or deviations from random mating. Population genetics is the study of changes in allele and genotype frequencies that occur when these factors act on animals or plants.
Image not available in HTML version |
Punnett square with allele frequencies
Genetics is a dynamic and exciting field (but, of course, you would expect us to say something like that). But it can also be confusing, since there are so many levels at which you can look at inheritance and the use of genetic information. This introduction to genetic organization and scale is intended as a kind of outline to some key levels and processes. Although they overlap, we can readily see three main perspectives. First is the molecular level of DNA structure and coding (nucleotide—DNA—transcription—translation—protein product). Second are the rules of genetic transmission, which are based on probabilities of inheritance (segregation and independent assortment in meiosis—probabilities and genotypes—genes in families—genes in populations). Third is the way the genotype controls biochemical activities during development to produce the organism’s phenotype (proteins—enzyme control of biochemical pathways—gene interactions—development). This primer will investigate these areas of genetics individually. But it is always important to keep in mind that they are really just different ways of looking at the same thing: the coding, transmission, and use of information by cells.
CHAPTER TWO
Mitosis and Meiosis
STUDY HINTS
Genes are located on chromosomes, and the stable manner in which chromosomes are first replicated and then distributed to daughter cells during cell division is the basis for genetic inheritance. Since much of genetic theory is based on the behavior of chromosomes and the genes they carry, it is very important to understand clearly how nuclear division occurs. In this way you can predict its consequences and understand the effect of errors that might occur in it. Yet the subject of cell division is complex, with many new terms to memorize and numerous things happening simultaneously. It is a continuous process that has been divided into stages somewhat artificially, so that we can describe it conveniently. All of this makes it rather hard to grasp at the beginning. Do not despair! It is really much simpler than it looks at first. The secret is to learn in stages. First one must understand the “strategies” of mitosis and meiosis, and the differences between them.
Mitosis has evolved as a mechanism to distribute accurately a copy of each chromo-some present in the original cell to two new cells. The “goal” of meiosis is quite different. Meiosis passes alternate (homologous) copies of each type of chromosome to daughter cells and reduces the total chromosome number by half. These different objectives require slightly different chromosome behaviors. We shall briefly summarize these two processes, keeping in mind the different strategies they represent.
Both processes begin in essentially the same way. The chromosome (and the deoxy-ribonucleic acid [DNA] molecule it contains) duplicates, forming two identical chromosome strands attached to each other at the centromere. This is accompanied by a physical reorganization (coiling) that greatly reduces the chromosome’s apparent length. The transition between these levels is illustrated in the following figure. One of the earliest signs of coiling is the formation of chromomeres, shown in this “magnified” insert to part A in the figure.
Image not available in HTML version |
During late interphase, nuclear DNA and the histone proteins in chromosomes (A) duplicate (B), though remaining attached at the centromere. The centromere is really a constriction, but we draw it here as a dark spot so it can be seen easily. The two identical DNA copies are called sister chromatids. During prophase (C and D), these DNA strands coil into discretely identifiable chromosomes.
Each of the identical chromosome strands coils to form one of the two strands (sister chromatids) in a duplicated chromosome. The shape of the chromosome is determined by the position of the centromere. Without such coiling (or condensation), separating chromosomes would be a little like trying to separate a plate of spaghetti into two piles without breaking anything. People sometimes get confused about chromosome number at this point. Just remember that chromosome means “colored body.” It is a single structural unit, no matter how much DNA it holds. So when counting chromosomes, count the centromeres, since whatever is attached to a centromere is a chromosomal unit.
In meiosis, the objective is to reduce the chromosome number so that there is only one copy of each kind in a gamete. The most direct way to do this is to pair the chromosomes carrying the same type of information or the same linear array of genes (homologous chromosomes). This is one purpose of synapsis. The first meiotic division, therefore, simply separates (segregates) homologous copies of each chromosome. The second meiotic division, like mitosis, distributes the identical copies (sister chromatids) that originated from chromosome replication.
Image not available in HTML version |
These two strategies are contrasted in the preceding figure, in which the original cell has a chromosome number of 2n = 2. In the Important Terms section of the chapter we have listed some of the most important terms you need to know to describe these processes. Definitions are given in the Glossary.
The only additional hint we might add concerns prophase I of meiosis. The divisions of this phase of meiosis are described in your text and summarized in Table 2.1. It is sometimes useful to have a mnemonic (memory-assisting) device to help you remember the sequence of events. For the divisions of prophase I, try this phrase, whose first letters are the same as the letters of the terms you need to remember: “Leaping zebras pound down dunes”: that is, leptotene, zygotene, pachytene, diplotene, diakinesis (see Table 2.1). Also remember these divisions are somewhat artificial, since the process is continuous, and some cell biologists assign events in a slightly different way.
TABLE 2.1 Substages of Prophase I of Meiosis
I. Leptotene |
A. Chromatin begins condensing and becomes visible. |
1. Chromomeres form as irregular tightly coiled regions of the chromosome. |
a. Location, size, and number of chromomeres are chromosome specific. |
b. G bands on human chromosomes are represented by several chromomeres coiling together. |
c. Homologous chromosomes have the same banding pattern. |
B. Homologous search begins. |
C. Telomere proteins attach the telomeres to nuclear membrane. |
II. Zygotene |
A. Pairing of homologous chromosomes occurs. |
1. Homologous search and rough pairing take place. |
2. Synaptonemal complex is present but homologues are not tightly synapsed. |
a. The pairs of chromosomes are called bivalents at this stage. |
b. The number of bivalents is equal to the haploid number. |
B. Chromosomes continue to coil and shorten. |
III. Pachytene |
A. Synapsis tightens so homologues are very close together (about 100 Å between the pairs). The term tetrad is often used here to indicate the presence of 4 chromatids (2 for each chromosome). |
B. Recombination occurs. |
C. The telomeres lose contact with the nuclear membrane. |
IV. Diplotene |
A. The transition between pachytene and diplotene occurs when the synaptonemal complex breaks down. |
B. Homologues begin to move apart. |
C. Chiasmata are present. |
D. Terminalization begins.a |
V. Diakinesis |
A. The homologous chromosomes continue to terminalize.a |
B. Nuclear membrane breaks down. |
C. Chromosomes move toward the equatorial plate. |
a As terminalization is completed, the chromosomes line up in the center of the cell as the cell enters metaphase I.
IMPORTANT TERMS
Acrocentric | Euchromatin | Polar body |
Bivalent | Gamete | Primary oocyte |
Centriole | Haploid | Primary spermatocyte |
Centromere | Heterochromatin | Secondary oocyte |
Chiasma (pl., chiasmata) | Homologous | Secondary spermatocyte |
Chromatid | chromosomes | Spindle |
Chromatin | Linkage group | Synapsis |
Chromomere | Meiosis | Synaptonemal complex |
Cytokinesis | Metacentric | Telocentric |
Diploid | Mitosis | Terminalization |
Dyad | Nucleolus | Tetrad |
PROBLEM SET 2
General Study Hint: To help reinforce these ideas, you should sketch the cell and its chromosomes before trying to answer these questions.
1. Suppose that you are studying a new species in which the chromosome number is unknown. You are looking at a spread of cells in some unknown stage of cell division, and you discover that there are seven chromosomes (three metacentric, three telocentric, and one acrocentric) visible in each dividing cell. What type of nuclear division have you found, and what conclusions can be drawn about the chromosome makeup of the species?
2. How many chromosomes are there in a human intestinal cell at anaphase?
3. How many different kinds of gametes can be produced by an individual who has three linkage groups with each chromosome identifiable by the forms of the genes (alleles) it carries?
4. In an organism with a haploid chromosome number of 7, how many sister chromatids are present
(a) in its mitotic metaphase nucleus?
(b) in its meiotic metaphase I nucleus?
(c) in its meiotic metaphase II nucleus?
5. A particular beetle species is found to have a chromosome complement of 2n = 16 chromosomes. How many linkage groups would the beetle have?
6. Recombination can be seen as chiasmata between homologous chromosomes at what stage of cell division? Sketch a bivalent involving metacentric chromosomes having one chiasma.
7. Is it possible for meiosis to occur in a haploid cell? Is mitosis possible? Please explain your answer to each question.
8. For each of the following figures, identify the specific stage of nuclear division and the diploid, 2n, chromosome number of the organism.
Image not available in HTML version |
9. Draw and label a cell at metaphase I of meiosis, where 2n = 8 metacentric chromosomes.
10. Draw and label a cell at late anaphase of mitosis, where 2n = 6 chromosomes (two pairs of acrocentric chromosomes and one pair of telocentric chromosomes).
11. Draw and label all cells at telophase I that result from meiosis of a primary spermatocyte (2n = 8 acrocentric chromosomes).
12. Draw, label, and compare cells at prophase I and prophase II of meiosis in a species characterized by2n = 6 acrocentric chromosomes.
13. Draw, label, and compare cells at anaphase of mitosis and anaphase I of meiosis in a species having 2n = 4 chromosomes (one homologous pair of metacentric chromosomes and one pair of telocentric chromosomes).
14. In humans, atopic allergic disease (ragweed hay fever) is dependent on a dominant gene A, while a neurological disorder called Huntington disease is determined by a dominant gene H. The normal alleles are a and h, respectively. A man who is Aahh married a woman who is aaHh. Assume the A and H are on nonhomologous chromosomes and that the relevant chromosomes are all telocentric.
(a) Diagram the chromosomal constitution of the each parent at metaphase I and label each chromatid with the associated genotype.
(b) Diagram the gametes that each parent would produce and label chromosomes in each gamete.
15. In studying the chromosomes of a new species of moth, you discover that there are eight bivalents formed in meiosis. From this you can conclude that the species has how many linkage groups? (a) 4; (b) 8; (c) 16; (d) 32; (e) not enough information is presented to answer the question.
16. A mature rat’s sperm cell has an amount of DNA that is the equivalent of a haploid genome, which we will call a “c” amount of DNA. Compared to this sperm cell, how much DNA will a somatic cell have if it is in the G2 phase of interphase? (a) c; (b) 2c; (c) 3c; (d) 4c; (e) more than 4c.
© Cambridge University Press