Practical Environmental Analysis / Edition 2 available in Hardcover, eBook
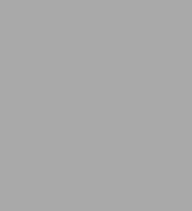
Practical Environmental Analysis / Edition 2
- ISBN-10:
- 0854046798
- ISBN-13:
- 9780854046799
- Pub. Date:
- 04/26/2006
- Publisher:
- RSC
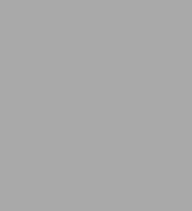
Practical Environmental Analysis / Edition 2
Buy New
$56.00Buy Used
$42.46-
SHIP THIS ITEM— Not Eligible for Free Shipping
-
SHIP THIS ITEM
Temporarily Out of Stock Online
Please check back later for updated availability.
-
Overview
Product Details
ISBN-13: | 9780854046799 |
---|---|
Publisher: | RSC |
Publication date: | 04/26/2006 |
Edition description: | Second Edition,New edition |
Pages: | 479 |
Product dimensions: | 6.15(w) x 9.20(h) x (d) |
About the Author
Dr. Miroslav Radojevic, Associate Professor. School of Science and Technology, Univeristy Malaysia Sabah.
Professor, Dr. Vladimir Bashkin is the author of 22 books, including Modern Biogeochemistry and Environmental Chemistry: Asian lessons (by Kluwer), and more than 100 papers. Under his supervision more than 20 PhD and DrSc dissertations were presented in various countries and universities. He is the board member of 5 international journals in field of environmental pollution. During 5 yr period he was selected as a vice-chairman of Working Group of Effects (scientific committee) of UN/EC Long-Range Trans-boundary Air Pollution Convention.
Read an Excerpt
Practical Environmental Analysis
By Miroslav Radojevic, Vladimir N. Bashkin
The Royal Society of Chemistry
Copyright © 1999 The Royal Society of ChemistryAll rights reserved.
ISBN: 978-1-84755-174-0
CHAPTER 1
Introduction
1.1 THE ENVIRONMENT
The environment is the sum total of human surroundings consisting of the atmosphere, the hydrosphere, the lithosphere and the biota. Human beings are totally dependent on the environment for life itself. The atmosphere provides us with the air we breathe, the hydrosphere provides the water we drink and the soil of the lithosphere provides us with the vegetables that we eat. In addition, the environment provides us with the raw materials to fulfill our other needs: the construction of housing, the production of the numerous consumer goods, etc. In view of these important functions it is imperative that we maintain the environment in as pristine a state as is possible. Fouling of the environment by the products of our industrial society (i.e. pollution) can have many harmful consequences, damage to human health being of greatest concern.
In addition to the outdoor environment, increasing concern is being expressed about the exposure of individuals to harmful pollutants within the indoor environment, both at home and at work. Levels of harmful pollutants can often be higher indoors than outdoors, and this is especially true of the workplace where workers can be exposed to fairly high levels of toxic substances. Occupational health, occupational medicine, and industrial hygiene are subjects that deal with exposure at the workplace.
Pollution is mainly, although not exclusively, chemical in nature. The job of the environmental analyst is therefore of great importance to society. Ultimately, it is the environmental analyst who keeps us informed about the quality of our environment and alerts us to any major pollution incidents which may warrant our concern and response.
1.1.1 Biogeochemical Cycles
The different components of the biosphere and their interactions are illustrated in Figure 1.1. The biosphere is that part of the environment where life exists. It consists of the hydrosphere (oceans, rivers, lakes), the lower part of the atmosphere, the upper layer of the lithosphere (soil) and all life forms. The concept of the biosphere was first introduced by the Russian scientist Vladimir Vernadsky (1863–1945) as the "sphere of living organisms distribution". Vernadsky was among the first to recognise the important role played by living organisms in various interactions within the biosphere, and he established the first-ever biogeochemical laboratory specifically dedicated to the study of these interactions. He expounded his theories in an aptly entitled book, "Biosphere", published in 1926.
The various spheres act as reservoirs of environmental constituents and they are closely linked through various physical, chemical and biological processes; there is constant exchange of material between them. Chemical substances can move through the biosphere from one reservoir to another, and this transport of constituents is described in terms of a biogeochemical cycle. Biogeochemical cycles of many elements are closely linked to the hydrological cycle, The hydrological cycle acts as a vehicle for moving water soluble nutrients and pollutants through the environment. If all the components of the cycle are identified and the amounts and rates of material transfer quantified, the term budget is used. Both beneficial nutrients and harmful pollutants are transported through biogeochemical cycles with far-reaching consequences. The more commonly discussed biogeochemical cycles are those of important macronutrients such as carbon, sulfur, nitrogen and phosphorus, but, in principle, a biogeochemical cycle could be drawn up for any substance. The cycle is usually illustrated as a series of compartments (reservoirs) and pathways between them. Each reservoir can be viewed in terms of a box model shown in Figure 1.2.
If the input into a reservoir equals the output, the system is said to be in a steady state. The residence time, τ, is defined as:
τ = Amount of substance in the reservoir (mass)/ Flux (mass/time)
Flux is the rate of transfer through the reservoir (i.e. the rate of input or output). If the input exceeds the output, there will be an increase in the amount of substance in the reservoir. There are many examples of the build-up of pollution in environmental systems since pollutants are often added at rates greater than the rates of natural processes that act to remove them from the system. On the other hand, if the output is greater than the input, the amount of substance in a reservoir will decrease. An example of this is the depletion of natural resources.
It is debatable whether, in the absence of human activities, natural systems would tend towards some sort of steady-state or equilibrium. Natural systems are dynamic, and both natural and human-induced disturbances lead to change, albeit over different time scales. Natural changes to biogeochemical cycles generally take place over geological time scales, and for millennia these cycles have maintained the delicate balance of nature conducive to life. However, since the industrial revolution, and especially over the last 40 years, human activities have caused significant perturbations to these cycles. The effects of these disruptions are already becoming apparent, and are likely to become even more severe in the coming millennium. Serious environmental problems that have been caused by disruptions of biogeochemical cycles include: global warming, acid rain, depletion of the ozone layer, bioaccumulation of toxic wastes and decline in freshwater resources. Modelling of biogeochemical cycles is becoming increasingly important in understanding, and predicting, human impacts on the environment, and the possibility of using biogeochemical cycles to solve environmental problems, so-called biogeochemical engineering, has recently been recognised.
Some of the major human impacts on biogeochemical cycles are given in Table 1.1.
The extent of human impacts on biogeochemical cycles can be illustrated by comparing the contribution of anthropogenic emissions to the atmosphere with natural emissions (Table 1.2). For some toxic substances the contribution of industrial emissions is even more striking: the ratio of anthropogenic to natural emissions to the environment is 3:1 for arsenic, 5:1 for cadmium, 10:1 for mercury and 28:1 for lead.
1.1.2 Environmental Pollution
Pollution is commonly defined as the addition of a substance by human activity to the environment which can cause injury to human health or damage to natural ecosystems. This definition excludes "natural pollution", although natural processes can also release harmful substances into the environment. There are different categories of pollution: chemical, physical, radioactive, biological and aesthetic. This book is concerned primarily with chemical pollutants and their determination in environmental matrices.
Most substances that are considered as pollutants are actually natural constituents of the environment, albeit at concentrations which are generally harmless. It is the increase in the concentration of these natural constituents, usually by industrial activity, to levels at which they may have harmful effects that is of concern. There are, however, a few pollutants which are entirely synthetic and would not be present in the environment if it were not for human activity (e.g. chlorofluoro-carbons).
Pollution can be classified according to its geographical scale as local, regional or global. Local pollution may affect only a single field, small stream or a city (e.g. photochemical smog). Regional pollution may affect a part of a country, a whole country or even an entire continent. Global warming due to the greenhouse effect of CO2 is an example of a pollution problem on a global scale. However, the distinction between these different categories is not always clear-cut. For example, many contemporary megacities extend over enormous areas and many urban conurbations may consist of several cities (Metro Manila, Los Angeles area, north-eastern seaboard of the USA, etc.). In such areas, photo-chemical smog is a regional problem. Acid rain was, until lately, considered a regional problem as it affects almost all of Europe and North America. However, acid rain has recently been identified at locations throughout the world, from tropical rainforests in Asia, Africa and South America to the polar ice caps in the Arctic. Therefore, acid rain may now be viewed as a global problem.
The number of pollution sources is constantly rising throughout the world as a consequence of growing industrial development. The driving force behind the increase in pollution is the rapidly growing population of the world (Figure 1.3). The world's population has more than doubled over the last 40 years, and over the next 30 years it is expected to increase by another 2–4 billion. The consequent demand for energy and resources required to feed, clothe and house the increasing population will be accompanied by a parallel increase in waste production. Not only is the population rising, but the standard of living is also rising, placing additional stress on the environment. In the future we may expect environmental problems to become more widespread and more severe unless measures are taken to control pollution.
Problems of environmental pollution have been widely recognised only in the latter half of the 20th century, but they have been known since antiquity. In fact, air pollution has been around since the first humans started using fire for heating, lighting and cooking. The air quality in inhabited prehistoric caves must, almost certainly, have been poor, and early humans must have been exposed to elevated levels of combustion products. In antiquity, and in the Middle Ages, the air of towns was polluted by the products of burning wood and coal, and the smelting of ores of iron and other metals. Also, in the absence of a sewage system, human and other wastes were dumped onto the streets, contributing to the rise of many epidemics. Furthermore, human beings have been throwing their wastes into surface waters since time immemorial. However, most of these early problems were local and had limited impacts, and natural processes within the environment were capable of rapidly diluting and eliminating the pollution.
It was the Industrial Revolution that greatly accelerated the release of pollutants into the environment. The Industrial Revolution originated in the north of England in the late 18th/early 19th century and quickly spread to other regions of Europe and North America. Rapid industrialisation is still going on throughout the world, especially in the developing countries of Asia, Africa and South America. The variety of pollutants and the extent of pollution is now greater than ever in history, and this trend looks set to continue well into the next millennium. Natural processes can no longer cleanse the environment of the enormous quantities of pollutants that are generated daily, and the pollution is steadily accumulating in the air, the oceans and the soil.
While natural ecosystems may accommodate a certain amount of pollution, this capacity is now being overloaded. When pollution levels reach a critical limit, harmful consequences follow. The legacy of present industrial development may have a dramatic impact on future generations.
Technological progress has been a two-edged weapon. Technology has given us enormous power over nature, to use for better or for worse. Medical and technological advances have eradicated many diseases, improved health care, provided protection against many natural disasters, increased the standard of living, eliminated many dangerous jobs, improved safety at work, etc. On the other hand, we now have the ability not only to destroy isolated ecosystems but all life on the earth, including human life, and not just by means of weapons of mass destruction but also by our polluting influence on the environment. The "globalisation" of what were previously minor, local environmental problems (e.g. acid rain), as well as the emergence of new global threats (e.g. destruction of stratospheric ozone, global warming), seems to indicate that we are well on our way to accomplishing this. It would be a sad indictment on the human race if it were to undo, in a small fraction of the geological time scale, what took nature millions of years to achieve: life in its many forms. Clearly, the real-world experiment which we are conducting needs to be carefully controlled if we are to slow down, or reverse, this trend.
However, it is not all gloom and doom; there have been many environmental success stories over the years. Unfortunately, these successes have so far been mainly limited to the developed nations. For example, air quality of most cities in Western Europe and North America has significantly improved compared to half a century ago. Concentrations of SO2 and smoke have decreased steadily since the 1950s and catastrophic smog episodes are no longer a menace to urban populations. More recently, the introduction of catalytic converters could reduce the emissions of automotive air pollutants and improve urban air quality even further. "Car-free" zones have been introduced in some cities and improvements in public transport have been implemented. DDT, organotin compounds, phosphate-containing detergents and many other harmful chemicals have been banned in most developed nations. Water quality has improved in some countries compared to what it was during the Industrial Revolution. Many countries have phased out the use of lead in petrol. Strict controls have been imposed on the transport and disposal of toxic wastes. Increasing emphasis is being placed on so-called "clean technologies". Considerable research has gone into developing alternative, non-polluting energy sources (solar, wind, etc.). The greater general awareness of environmental problems has resulted in the public raising environmental issues and demanding greater environmental accountability from industries and governments. Unfortunately, similar improvements are not evident in developing countries, where development has been accompanied by increasing environmental devastation. Over the past 20 years, the relatively unspoiled environment of these countries has regressed to a state on a par with that of the developed countries during the Industrial Revolution. However, there is cause for optimism. As these countries increasingly adopt pollution control technologies, much as they have adopted other technologies pioneered by the developed nations, the quality of the environment could yet improve.
1.1.3 Environmental Standards
Whether a specific concentration of a particular chemical substance is harmful or not depends on many factors and is the subject of extensive research among various branches of science. The maximum level of a substance which can be allowed in the environment without any foreseeable harmful effects is called a standard, and the establishment of such standards is a complex and difficult process. Many standards change quite frequently as new research sheds more light on the effects of pollution or as better control technologies become available. The tendency is for standard values to decrease (i.e. become more stringent) with time. Most countries specify standards for many air pollutants, water pollutants, etc. These standards are legally enforceable and offenders may be prosecuted for infringement. There are two types of standards:
Quality standards. These refer to the concentration of a pollutant in the environment. For example, air quality standards specify the concentrations of pollutants in the general atmosphere that are not to be exceeded. Such standards are used to maintain the quality of our environment in a generally unpolluted, if not pristine, state.
Emission standards. These refer to the maximum levels of a pollutant that may be emitted from a particular pollution source. For example, waste waters from a particular industry must have con centrations of specific pollutants below the levels required by the emission standard.
Furthermore, there are also guidelines. These are not legally binding but they are recommended levels of pollutants which, if exceeded, may result in some harmful effect. A well known guideline is the World Health Organisation (WHO) recommendations for drinking water quality which cover a large number of inorganic and organic species (see Appendix III). WHO guidelines for three important air pollutants are given in Table 1.3.
The job of the environmental analyst is to test for compliance with the various standards. If it is established that permissible levels are being exceeded, technological measures may be required in order to reduce the emissions of pollutants. For example, such measures may include the installation of a water treatment plant to control the discharge of waste waters, or the operation of a flue gas desulfurisation plant to reduce atmospheric emissions from a power station. The selection and design of the most appropriate pollution control technology is made by environmental engineers. Environmental analysts may be required to assess the efficiency of the control technology, and once installed, to confirm that the problem has been eliminated and that legislative standards are being adhered to. International and national guidelines and standards for air, water, soil, sludge, crops and foods are listed in Appendix 111.
(Continues...)
Excerpted from Practical Environmental Analysis by Miroslav Radojevic, Vladimir N. Bashkin. Copyright © 1999 The Royal Society of Chemistry. Excerpted by permission of The Royal Society of Chemistry.
All rights reserved. No part of this excerpt may be reproduced or reprinted without permission in writing from the publisher.
Excerpts are provided by Dial-A-Book Inc. solely for the personal use of visitors to this web site.
Table of Contents
Chapter 1: Introduction; 1.1: The environment; 1.2: Environmental Analysis; 1.3: Sampling and Atorage; 1.4: Sample Treatment; 1.5: Analytical Methods; 1.6: Standardisation and Calibration; 1.7: Analytical data; 1.8: Common Problems of Environmental Analysis; 1.9: Ethic; 1.10: A Word of Advice; Chapter 2: Rainwater Analysis; 2.1: Acid Rain; 2.2: Sampling and Analysis; 2.3: Data Analysis and Interpretation; 2.4: pH, Conductivity, and Major Anions; 2.5: Major Cations; 2.6: Heavy Metals; 2.7: Exercises and Information; Chapter 3: Air Analysis; 3.1: Introduction; 3.2: Sampling; 3.3: Sulfur dioxide (SO2); 3.4: Nitrogen dioxide (NO2); 3.5: Ozone (O3); 3.6: Ammonia (NH3); 3.7: Hydrogen chloride (HCl); 3.8: Atmospheric Aerosol: Heavy Metals and Major Constituents; Chapter 4: Water Analysis; 4.1: Introduction; 4.2: Solids in Water; 4.3: Electrochemical Measurements; 4.4: Hardness; 4.5: Alkalinity; 4.6: Dissolved Oxygen (DO); 4.7: Biochemical Oxygen Demand (BOD); 4.8: Chemical Oxygen Demand (COD); 4.9: Chlorophyll; 4.10: Nitrogen; 4.11: Phosphorus; 4.12: Chloride; 4.13: Fluoride; 4.14: Sulfate; 4.15: Iron; 4.16: Heavy Metals; 4.17: Oil and Grease; Chapter 5: Soil, Sludge, Sediment and Dust Analysis; 5.1: Introduction; 5.2: Sampling and Sample Preparation; 5.3: Dissolution and Extraction; 5.4: Physical Parameters; 5.5: Electrochemical Measurements; 5.6: Alkalinity; 5.7: Soluble Ions; 5.8: Organic Matter; 5.9: Nitrogen; 5.10: Phosphorus; 5.11: Sulfur; 5.12: Exchangeable Cations (Ca2+ , Mg2+ , K+, and Na+) and Cation Exchange capacity (CEC); 5.13: Heavy Metals; Chapter 6 : Plant Analysis; 6.1: Introduction; 6.2: Sampling and Sample Preparation; 6.3: Dissolution and Extraction; 6.4: Water Content and Ash Content; 6.5: Nitrogen, Phosphorus, and Sulfur; 6.6: Potassium, Sodium, Calcium, and Magnesium; 6.7: Nitrate and Nitrite; 6.8: Heavy MetalsWhat People are Saying About This
It sets out, clearly and logically, procedures for measuring a wide range of chemical elements and compounds across a spectrum of environmental fields. This book will serve the need to train new environmental analysts, and remind existing analysts of the details of the enormous range of skills needed for practical environmental analysis.