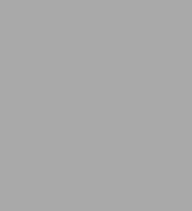
Power Sources and Supplies: World Class Designs: World Class Designs
400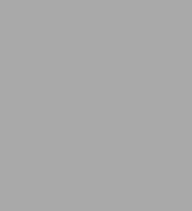
Power Sources and Supplies: World Class Designs: World Class Designs
400eBook
Available on Compatible NOOK devices, the free NOOK App and in My Digital Library.
Related collections and offers
Overview
*Marty Brown, author and power supply design consultant, has personally selected all content for its relevance and usefulness
*Covers best design practices for switching power supplies and power converters
*Emphasis is on pragmatic solutions to commonly encountered design problems and tasks
Product Details
ISBN-13: | 9780080556574 |
---|---|
Publisher: | Elsevier Science |
Publication date: | 04/08/2011 |
Series: | World Class Designs |
Sold by: | Barnes & Noble |
Format: | eBook |
Pages: | 400 |
File size: | 5 MB |
About the Author
Marty Brown is the author of the Power Supply Cookbook and Practical Switching Power Supply Design. He earned his amateur radio license at the age of 11 and has had electronics as a hobby throughout his life. He graduated cum laude from Drexel University in 1974. His electronic design history includes underwater acoustics with the department of the Navy, airborne weather radar design (digital and SMPS), a satellite CODEC, and process control equipment. He was previously with Motorola Semiconductor as a principle application engineer, where he defined more than eight semiconductor products in the power conversion market and received two patents. He later started his own electronics consulting firm where he designed products from satellite power systems to power-related integrated circuits for many semiconductor companies. He is presently working in the field of digitally-controlled power supplies with Microchip Technologies. He has eight children, five of whom are adopted. His wife is an internationally known writer and speaker in the area of inter-racial adoption and related issues. He presently lives in Scottsdale, Arizona.
Read an Excerpt
Power Sources and Supplies
World Class DesignsBy Marty Brown Nihal Kularatna Raymond A. Mack, Jr. Sanjaya Maniktala
Newnes
Copyright © 2008 Elsevier Inc.All right reserved.
ISBN: 978-0-08-055657-4
Chapter One
An Introduction to the Linear Regulator Marty Brown
Linear power supplies are the simplest of the DC/DC converters, but don't be fooled by the apparent simplicity of them. There are several factors in every application of linear supplies that are important for their reliable operation. These are thermal design, output regulation, stability considerations and its transient response, any of which could cause the system to behave badly.
Linear regulators are used much more often than switching regulators. One finds them distributed throughout products as POL (point of load) supplies, where local circuit regulation is needed, voltage bus quieting for noise sensitive circuits, and inexpensive voltage bus generation.
If you have done a design completely using linear regulators, you may technically call yourself a "power supply designer," but you will not fully appreciate the complexities of the field until you have experienced a switching power supply design. You have only reached the " tenderfoot " level of experience.
I've attempted to cover the material in a succinct and intuitive manner showing how flexible the humble linear regulator can be. The design examples can be scaled and adapted to many other applications. Related topics such as thermal design can be found in chapter 12.
—Marty Brown
The linear regulator is the original form of the regulating power supply. It relies upon the variable conductivity of an active electronic device to drop voltage from an input voltage to a regulated output voltage. In accomplishing this, the linear regulator wastes a lot of power in the form of heat, and therefore gets hot. It is, though, a very electrically "quiet" power supply.
The linear power supply finds a very strong niche within applications where its inefficiency is not important. These include wall-powered, ground-base equipment where forced air cooling is not a problem; and also those applications in which the instrument is so sensitive to electrical noise that it requires an electrically "quiet" power supply—these products might include audio and video amplifiers, RF receivers, and so forth. Linear regulators are also popular as local, board-level regulators. Here only a few watts are needed by the board, so the few watts of loss can be accommodated by a simple heatsink. If dielectric isolation is desired from an AC input power source, it is provided by an AC transformer or bulk power supply.
In general, the linear regulator is quite useful for those power supply applications requiring less than 10W of output power. Above 10W, the heatsink required becomes so large and expensive that a switching power supply becomes more attractive.
1.1 Basic Linear Regulator Operation
All power supplies work under the same basic principle, whether the supply is a linear or a more complicated switching supply. All power supplies have at their heart a closed negative feedback loop. This feedback loop does nothing more than hold the output voltage at a constant value. Figure 1.1 shows the major parts of a series-pass linear regulator.
Linear regulators are step-down regulators only; that is, the input voltage source must be higher than the desired output voltage. There are two types of linear regulators: the shunt regulator and the series-pass regulator. The shunt regulator is a voltage regulator that is placed in parallel with the load. An unregulated current source is connected to a higher voltage source; the shunt regulator draws output current to maintain a constant voltage across the load given a variable input voltage and load current. A common example of this is a Zener diode regulator. The series-pass linear regulator is more efficient than the shunt regulator and uses an active semiconductor as the series-pass unit, between the input source and the load.
The series-pass unit operates in the linear mode, which means that the unit is not designed to operate in the full on or off mode but instead operates in a degree of "partially on." The negative feedback loop determines the degree of conductivity the pass unit should assume to maintain the output voltage.
The heart of the negative feedback loop is a high-gain operational amplifier called a voltage error amplifier. Its purpose is to continuously compare the difference between a very stable voltage reference and the output voltage. If the output differs by mere millivolts, then a correction to the pass unit's conductivity is made. A stable voltage reference is placed on the noninverting input and is usually lower than the output voltage. The output voltage is divided down to the level of the voltage reference. This divided output voltage is placed into the inverting input of the operational amplifier. So at the rated output voltage, the center node of the output voltage divider is identical to the reference voltage.
The gain of the error amplifier produces a voltage that represents the greatly amplified difference between the reference and the output voltage (error voltage). The error voltage directly controls the conductivity of the pass unit thus maintaining the rated output voltage. If the load increases, the output voltage will fall. This will then increase the amplifier's output, thus providing more current to the load. Similarly, if the load decreases, the output voltage will rise, thus making the error amplifier respond by decreasing pass unit current to the load.
The speed by which the error amplifier responds to any changes on the output and how accurately the output voltage is maintained depends on the error amplifier's feedback loop compensation. The feedback compensation is controlled by the placement of elements within the voltage divider and between the negative input and the output of the error amplifier. Its design dictates how much gain at DC is exhibited, which dictates how accurate output voltage will be. It also dictates how much gain at a higher frequency and bandwidth the amplifier exhibits, which dictates the time it takes to respond to output load changes or transient response time.
The operation of a linear regulator is very simple. The very same circuitry exists in the heart of all regulators, including the more complicated switching regulators. The voltage feedback loop performs the ultimate function of the power supply—the maintaining of the output voltage.
1.2 General Linear Regulator Considerations
The majority of linear regulator applications today are board-level, low-power applications that are easily satisfied through the use of highly integrated three-terminal regulator integrated circuits. Occasionally, though, the application calls for either a higher output current or greater functionality than the three-terminal regulators can provide.
There are design considerations that are common to both approaches and those that are only applicable to the nonintegrated, custom designs. These considerations define the operating boundary conditions that the final design will meet, and the relevant ones must be calculated for each design. Unfortunately, many engineers neglect them and have trouble over the entire specified operating range of the product after production.
The first consideration is the headroom voltage. The headroom voltage is the actual voltage drop between the input voltage and the output voltage during operation. This enters predominantly into the later design process, but it should be considered first, just to see whether the linear supply is appropriate for the needs of the system. First, more than 95 percent of all the power lost within the linear regulator is lost across this voltage drop. This headroom loss is found by
PHR = (Vin(max) - Vout)[Iload(rated) (1-1)
If the system cannot handle the heat dissipated by this loss at its maximum specified ambient operating temperature, then another design approach should be taken. This loss determines how large a heatsink the linear regulator must have on the pass unit.
A quick estimated thermal analysis will reveal to the designer whether the linear regulator will have enough thermal margin to meet the needs of the product at its highest specified operating ambient temperature. One can find such a thermal analysis in Chapter 12.
The second major consideration is the minimum dropout voltage of a particular topology of linear regulator. This voltage is the minimum headroom voltage that can be experienced by the linear regulator, below which it falls out of regulation. This is predicated only by how the pass transistors derive their drive bias current and voltage. The common positive linear regulator utilizes an NPN bipolar power transistor (see Figure 1.2a). To generate the needed base-emitter voltage for the pass transistor's operation, this voltage must be derived from its own collector-emitter voltage. For the NPN pass units, this is the actual minimum headroom voltage. This dictates that the headroom voltage cannot get any lower than the base-emitter voltage (~0.65 VDC) of the NPN pass unit plus the drop across any base drive devices (transistors and resistors). For the three terminal regulators such as the MC78XX series, this voltage is 1.8 to 1.5 VDC. For custom designs using NPN pass transistors for positive outputs, the dropout voltage may be higher. For applications where the input voltage may come even closer than 1.8 to 1.5 VDC to the output voltage, a low dropout regulator is recommended. This topology utilizes a PNP pass transistor, which now derives its base-emitter voltage from the output voltage instead of the headroom or input voltage (see Figure 1.2b). This allows the regulator to have a dropout voltage of 0.6 VDC minimum. P-Channel MOSFETs can also be used in this function and can exhibit dropout voltages close to zero volts.
The dropout voltage becomes a driving issue when the input to the linear regulator during normal operation is allowed to fall close to the output voltage. If operating from an AC wall transformer, this would occur at brown-out conditions (minimum AC voltages). The low dropout regulator (e.g., LM29XX) would allow the regulator to operate to a lower AC input voltage. Low dropout regulators are also widely used as post regulators on the output of switching power supplies. Within switching regulators, the efficiency is of great concern, so the headroom drop needs to be kept to a minimum. Here, the low dropout regulator will save several watts of loss over a conventional NPN-based linear regulator. If the application will never see headroom voltages less than 1.5V, then use the conventional linear regulators (e.g., MC78XX).
Another consideration is the type of pass unit to be used. From a headroom loss standpoint, it makes absolutely no difference whether a bipolar power transistor or a power MOSFET is used. The difference comes in the drive circuitry. If the headroom voltage is high, the controller (usually a ground-oriented circuit) must pull current from the input or output voltage to ground. For a single bipolar pass transistor this current is
IB = ILoad/hFE (1-2)
The power lost just in driving the bipolar pass transistor is
Pdrive = Vin/max · IB or Vout · IB (1-3)
This drive loss can become significant. A driver transistor can be added to the pass transistor to increase the effective gain of the pass unit and thus decrease the drive current, or a power MOSFET can be used as a pass unit that uses magnitudes less DC drive current than the bipolar power transistor. Unfortunately, the MOSFET requires up to 10 VDC to drive the gate. This can drastically increase the dropout voltage. In the vast majority of linear regulator applications, there is little difference in operation between a buffered pass unit and a MOSFET insofar as efficiency is concerned. Bipolar transistors are much less expensive than power MOSFETs and have less propensity to oscillate.
The linear regulator is a mature technology and therefore can usually be accommodated by the integrated solutions provided by the semiconductor manufacturers. For applications beyond the limits of these integrated linear regulators alone, usually adding more components around the IC will satisfy the requirement. Otherwise, a completely custom approach would need to be utilized. These various approaches are overviewed in the design examples in the following section.
(Continues...)
Excerpted from Power Sources and Supplies by Marty Brown Nihal Kularatna Raymond A. Mack, Jr. Sanjaya Maniktala Copyright © 2008 by Elsevier Inc. . Excerpted by permission of Newnes. All rights reserved. No part of this excerpt may be reproduced or reprinted without permission in writing from the publisher.
Excerpts are provided by Dial-A-Book Inc. solely for the personal use of visitors to this web site.
Table of Contents
Chapter 1. An Introduction to the Linear RegulatorChapter 2. Basic Switching Circuits
Chapter 3 DC-DC Converter Design and Magnetics
Chapter 4 Control Circuits
Chapter 5 Non-Isolated Circuits
Chapter 6 Transformer Isolated Circuits
Chapter 7 Power Semiconductors
Chapter 8 Conduction and Switching Losses
Chapter 9 Power Factor Correction
Chapter 10 Off-line Converter Design and Magnetics
Chapter 11 A “True Sine Wave? Inverter Design Example
Chapter 12 Thermal Analysis and Design