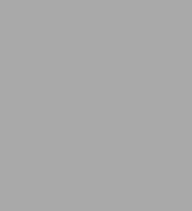
Power Integrity for Nanoscale Integrated Systems
416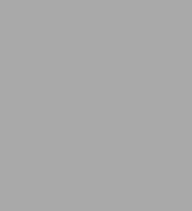
Power Integrity for Nanoscale Integrated Systems
416eBook
Available on Compatible NOOK devices, the free NOOK App and in My Digital Library.
Related collections and offers
Overview
This leading-edge guide discusses the impact of power integrity from a design perspective, emphasizing phenomena and problems induced by power integrity degradation and the latest design trends, including low-power design. Power Integrity for Nanoscale Integrated Systems describes how these problems can be forecast early in the design process and the countermeasures that can be used to address them, such as the inclusion of inductance and accurate modeling for PI analysis, as well as robust circuit design. Detailed examples and a case study on the IBM POWER7+ processor illustrate real-world applications of the techniques presented in this practical resource.
Coverage includes:
- Significance of power integrity for integrated circuits
- Supply and substrate noise impact on circuits
- Clock generation and distribution with power integrity
- Signal and power integrity design for I/O circuits
- Power integrity degradation and modeling
- Lumped, distributed, and 3D modeling for power integrity
- Chip temperature and PI impact
- Low-power techniques and PI impact
- Power integrity case study using the IBM POWER7+ processor chip
- Carbon nanotube interconnects for power delivery
Product Details
ISBN-13: | 9780071787772 |
---|---|
Publisher: | McGraw Hill LLC |
Publication date: | 03/07/2014 |
Sold by: | Barnes & Noble |
Format: | eBook |
Pages: | 416 |
File size: | 25 MB |
Note: | This product may take a few minutes to download. |
About the Author
Masanori Hashimoto is an Associate Professor in the department of Information Systems and Engineering, Graduate School of Information Science Technology, Osaka University. He has been working on modeling and measurement of on-chip power supply noise and signal coupling noise. Professor Hashimoto’s research interests include timing, power, and signal integrity analysis, ultra-low power design, design for reliability, performance optimization in physical design, and on-chip high-speed signaling. He has co-authored several journal articles.
Raj Nair, a consultant in IC power delivery and power integrity, is co-founder of Anasim Corporation, which commercializes power integrity and energy aware SoC (System on Chip) design tools.
Table of Contents
Contributors xv
Preface xvii
Acknowledgments xxi
1 Significance of Power Integrity for Integrated Circuits Raj Nair Masanori Hashimoto 1
1.1 Transistor Scaling and PI Degradation Progression 2
1.1.1 PI under Constant-Power and Constant-Power-Density Scaling 4
1.1.2 Low-Power Design and PI Degradation 6
1.1.3 Power Grid Noise in ICs 7
1.1.4 Impact of PI Degradation on I/O Circuits and Signal Integrity 11
1.2 Implications of Worsening PI 13
1.2.1 Yield Spreading and Loss Through PI Degradation 13
1.2.2 Diminished Voltage Scaling and Increasing Power 15
1.2.3 Fabrication and Packaging Technology Enhancement and Cost 17
1.2.4 Design and Verification Cost 18
1.2.5 Unsustainable Energy Wastage 19
1.3 References 20
2 Supply and Substrate Noise Impact on Circuits Masanori Hashimoto 21
2.1 Supply Noise and Substrate Noise 22
2.2 Path and Cell Delays and Supply Noise 24
2.2.1 Relation between Path Delay and Supply Noise 25
2.2.2 Combinatorial Cell Delay 30
2.2.3 Flip-Flop Timing Characteristics 33
2.3 Noise-Aware Circuit-Level Timing Analysis 37
2.3.1 Difficulties 37
2.3.2 Spatial and Temporal Correlation of Supply Noise 40
2.3.3 Statistical Noise Modeling 44
2.3.4 A Case Study 46
2.4 Noise Impact on Analog/RF Circuits 50
2.4.1 Supply Noise 50
2.4.2 Substrate Noise 52
2.5 Exercises 53
2.6 References 53
3 Clock Generation and Distribution with Power Integrity Yasuhiro Ogasahara Masanori Hashimoto Raj Nair 55
3.1 Delay, Skew, and Jitter of the Clock 56
3.2 Interconnect Elements for a Clock Tree 59
3.2.1 Parasitic Components of Interconnect Elements 59
3.2.2 Definitions of Inductance 60
3.2.3 Inductance Extraction 61
3.2.4 Interconnect Simulation 68
3.2.5 Specifying Inductive Interconnect Elements 71
3.2.6 Signal Transition Time and Inductance 74
3.3 Clock Tree Structures and Simulation 76
3.3.1 Clock Tree Structure 76
3.3.2 Industrial CDN Implementations 79
3.4 Clock Skew Under Power Supply Noise 80
3.4.1 Power Supply Noise in Sequential Circuits 80
3.4.2 Noise-Aware CDN Simulation 82
3.4.3 A Case Study on Clock Skew Analysis under V and T Variation 83
3.4.4 Related Works on Clock Skew and Supply Noise 88
3.5 Clock Generation 89
3.5.1 Brief Discussion on PLLs and DLLs Relating to PI 90
3.5.2 PLL Architecture 91
3.5.3 Rule 1: Isolate PLL Circuits from Noise 93
3.5.4 Rule 2: Design Single-Ended Circuits and Physical Layout Differentially 95
3.5.5 Rule 3: Design Loop Filter, Bias Generator, and VCO PSRR and Noise 98
3.6 Clock Extraction for Data Communications 100
3.6.1 Bang-Bang Phase Detectors 101
3.6.2 Data Recovery DLLs and Phase Interpolators 101
3.7 Summary 101
3.8 References 102
4 Signal and Power Integrity Design for I/O Circuits Toshio Sudo 105
4.1 Introduction 105
4.2 Single-Ended I/O Design 106
4.2.1 Modeling Simultaneous Switching Output Noise 106
4.2.2 Measurement of SSO Noise and Correlation with Simulation 109
4.2.3 Measurement of On-Die PDN and Anti-resonance Peak in the Full PDN 112
4.2.4 Co-simulation of SI and PI 114
4.2.5 Synthesized Total PDN Impedance Seen from the ASIC Chip 118
4.2.6 Frequency-Dependent Target Impedance 121
4.2.7 Signal Degradation Estimation Employing Frequency-Dependent Target Impedance 123
4.3 Differential I/O Design 123
4.3.1 Modeling of Signal Integrity of Differential I/O Circuit 124
4.3.2 Differential Transmission Line, Influence of Crosstalk Noise and Through-Hole Stub 125
4.3.3 Common-Mode Conversion by Woven Fiberglass 128
4.4 Power Integrity Design and Evaluation in 3D System-in-Package (SiP) 131
4.4.1 Benefits of Wide Bus Structures 131
4.4.2 Three Stacked Chips and Configuration of 3D SiP 132
4.4.3 Total PDN Impedance and Impact on SSO Noise 136
4.5 Summary 142
4.6 References 145
5 Power Integrity Degradation and Modeling Chung-Kuan Cheng Xiang Hu Amirali Shayan 147
5.1 Background 147
5.2 Power Integrity Modeling 150
5.2.1 Board Power Integrity 150
5.2.2 Package Power Integrity 151
5.2.3 On-Die Grid Power Integrity 151
5.3 Power Integrity Analysis 152
5.4 Frequency-Domain Analysis 152
5.5 Time-Domain Analysis 156
5.6 Target Impedance Background 156
5.7 Problem Formulation 157
5.8 Worst-Case PDN Output Voltage Noise 158
5.9 Impedance without Realizability Constraints 159
5.10 Impedance with Realizability Constraints 161
5.10.1 First-Order Impedance 161
5.10.2 Second-Order Impedance 162
5.11 Real Power Distribution Networks 169
5.11.1 Ideal LC Tank without ESRCc 169
5.11.2 Standard LC Tank with ESRCc 171
5.11.3 A Complete PDN Path 175
5.12 Summary 178
5.13 References 178
6 Lumped, Distributed, and 3D Modeling for Power Integrity Chung-Kuan Cheng Xiang Hu Amirali Shayan 181
6.1 3D PDN Modeling 181
6.1.1 Distributed Power Grid Model 182
6.1.2 Current Stimulus Model 183
6.1.3 Lumped Model 184
6.1.4 Effect of On-Chip Inductance 186
6.2 3D PDN Analysis Flow 189
6.3 Experimental Results 190
6.3.1 Current Distribution Model 191
6.3.2 Resonance Phenomena 196
6.3.3 Decoupling Capacitance 203
6.3.4 Connection Impedance between Tiers 205
6.3.5 Worst-Case Voltage Noise 208
6.3.6 Rogue Wave 211
6.4 Summary 212
6.5 Exercises 213
6.6 References 215
7 Chip Temperature and PI impact Kian Haghdad Mohab Anis 217
7.1 High-Temperature Effect on VLSI Circuits and Systems 217
7.1.1 Impact on Power 217
7.1.2 Implication for Performance 223
7.1.3 Reliability Concerns 228
7.2 Temperature Distribution and Impact on Voltage Drop 230
7.2.1 Distribution and Management of Temperature 230
7.2.2 Effect of Load Changes on Voltage Drop 234
7.2.3 Impact of Interconnect Resistance on Voltage Drop 235
7.3 Temperature and Voltage Drop Modeling and Estimation 236
7.3.1 Thermal Modeling 236
7.3.2 Direct Temperature Measurement 241
7.3.3 Modeling Voltage Drop 243
7.4 Thermal and Voltage Drop Profile for an Alpha Processor 244
7.5 Design Enhancements for Robust Power Distribution with High Temperature 250
7.5.1 Floor Planning 250
7.5.2 Supply Pad Assignment 252
7.5.3 From Design to Manufacturing 255
7.6 Summary 259
7.7 Exercises 260
7.8 References 262
8 Low-Power Techniques and PI Impact Masanori Hashimoto Raj Nair 265
8.1 Power Dissipation of Digital CMOS Circuits and Voltage Scaling 265
8.1.1 Dynamic Power 266
8.1.2 Short-Circuit Power 266
8.1.3 Leakage Power 266
8.2 Voltage Scaling 267
8.3 Clock Gating 272
8.3.1 Overview 272
8.3.2 Power Integrity Issues 274
8.3.3 Charge Pump Active Noise Regulation 278
8.4 Power Gating 279
8.4.1 Overview 279
8.4.2 Inrush Current and Inrush Current-Induced Noise 280
8.4.3 Well Structure and Inrush Current-Induced Noise 283
8.4.4 Mitigating Inrush Current-Induced Noise 288
8.4.5 Decoupling 291
8.4.6 Architectural, System-Level Techniques and Active Noise Regulation 292
8.5 Exercises 296
8.6 References 296
9 Power Integrity Case Study Using the IBM POWER7+ Processor Chip Howard H. Smith 299
9.1 Introduction 299
9.2 IBM POWER7+Description 300
9.3 Power Integrity Considerations for IBM Processor Chips 303
9.4 Load Current Estimation Approaches for Power Integrity 307
9.5 POWER7+ Power Grid Design Considerations 309
9.6 Static Power Grid Verification 317
9.7 Transient Power Grid Analysis 323
9.8 Acknowledgments 343
9.9 References 344
10 Carbon Nanotube Interconnect for Power Delivery Navin Srivastava Mizuhisa Nihei 345
10.1 Why a New Interconnect Material? 346
10.1.1 Increasing Copper Interconnect Resistivity 346
10.1.2 Electromigration Reliability 347
10.1.3 Scaling Trends for Copper Interconnect Elements 348
10.2 Basic Properties of Carbon Nanotubes 349
10.2.1 Chirality 350
10.2.2 Current-Carrying Capacity 351
10.2.3 Thermal Conductivity 352
10.3 Electrical Properties of Carbon Nanotubes 352
10.3.1 Resistance 353
10.3.2 Capacitance 354
10.3.3 Inductance 355
10.4 Carbon Nanotube Interconnect Elements 356
10.4.1 Resistance of a Carbon Nanotube Interconnect 357
10.4.2 Capacitance of a Carbon Nanotube Interconnect 359
10.4.3 Inductance of a Carbon Nanotube Interconnect 361
10.5 Thermal Management with Carbon Nanotube Interconnect elements 361
10.5.1 Through-Silicon Vias for 3D ICs 362
10.6 Fabrication and Integration of CNT Interconnect Elements 364
10.6.1 Carbon Nanotube Integration with Standard VLSI Process 364
10.6.2 Salient Features of CNT Interconnect Fabrication 365
10.7 Carbon Nanotubes for Power Integrity 368
10.7.1 Structural and Thermal Benefits 368
10.7.2 Electromigration Reliability 369
10.7.3 Low-Resistance Power Grids 370
10.8 Summary 370
10.9 References 372
Index 375