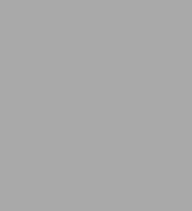
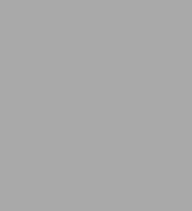
eBook
Available on Compatible NOOK devices, the free NOOK App and in My Digital Library.
Related collections and offers
Overview
The applications of ionic liquids can be enormously expanded by arranging the organic ions in the form of a polymer architecture. Polymerized ionic liquids (PILs), also known as poly(ionic liquid)s or polymeric ionic liquids, provide almost all features of ionic polymers plus a rare versatility in design. The mechanical properties of the solid or solid-like polymers can also be controlled by external stimuli, the basis for designing smart materials.
Known for over four decades, PILs are a member of the ionic polymers family. Although the previous forms of ionic polymers have a partial ionicity, PILs are entirely composed of ions. Therefore, they offer a better flexibility for designing a responsive architecture as smart materials. Despite the terminology, PILs can be synthesized from solid organic ionic salts since the monomer liquidity is not a requirement for the polymerization process. Ionicity can also be induced to a neutral polymer by post-polymerization treatments.
This is indeed an emerging field whose capabilities have been somehow overshadowed by the popularity of ionic liquids. However, recent reports in the literature have shown impressive potentials for the future. Written by leading authors, the present book provides a comprehensive overview of this exciting area, discussing various aspects of PILs and their applications as smart materials. Owing to the novelty of this area of research, the book will appeal to a broad readership including students and researchers from materials science, polymer science, chemistry, and physics.
Product Details
ISBN-13: | 9781788012218 |
---|---|
Publisher: | Royal Society of Chemistry |
Publication date: | 09/18/2017 |
Series: | ISSN |
Sold by: | Barnes & Noble |
Format: | eBook |
Pages: | 541 |
File size: | 12 MB |
Note: | This product may take a few minutes to download. |
About the Author
Åbo Akademi University, Sweden
Read an Excerpt
CHAPTER 1
Polymerization in Ionic Liquids
NIKHIL K. SINGHA, KUNLUN HONG AND JIMMY W. MAYS
Introduction
Most liquids used as solvents are composed of neutral molecules. In contrast, ionic liquids (ILs) are salts in the liquid state at ambient or near ambient temperatures. This room temperature ionic liquid state is often achieved by choosing ion pairs where one is organic and has a delocalized charge, or by choosing bulky asymmetric substituents. This causes the ions to be poorly coordinated, resulting in low melting temperatures. In principle, literally millions of ionic liquids with an exceptionally wide range of properties can be produced. This has led to ILs being considered as designer solvents or task specific solvents, where their extremely low vapor pressures offer potential to minimize pollution associated with volatile organic compound (VOC) solvents through recycling.
Some examples of common IL cations and anions and their general characteristics are shown in Figure 1.1. Imidazolium- and pyridinium-based ILs feature delocalized cations, whereas quaternary ammonium cations are asymmetrically substituted. A very wide range of properties, including viscosity, hydrophobicity or hydrophilicity, thermal and chemical stability, melting point, flammability and cost, can be tuned by judicious selection of the cation and anion.
Probably the first room temperature IL, ethylammonium nitrate (m.p. 12 °C), was reported by Walden in 1914. However, there was little interest in ILs until the mid-1970s when they attracted attention as electrolytes for batteries. Nowadays, ILs are being intensely investigated in a wide range of applications, including use as solvents in a wide range of chemical processes. ILs have been used as solvents for Diels-Alder reactions, hydrogenations, alkylations, Friedel-Crafts reactions, Heck reactions, Suzuki couplings, metathesis reactions,^ and many others.
To the best of our knowledge, the first polymerization in ILs was reported in 1990 by Carlin et al. They reported that TiCl4 and AlEthylCl in AlCl3/1-ethyl3-methylimmidazolium chloride ([EMIM]Cl) could polymerize ethylene in low yields. Subsequent work by the same group, where TiCl4 was replaced by Cp2TiCl2, gave higher yields. This pioneering work, using ILs as a reaction medium for polymerization, has inspired numerous researchers over the past quarter of a century to investigate a wide range of different types of polymerization in ILs. While much of this work was inspired by the "green" aspects of ionic liquids (very low vapor pressure and potential for recycling), it quickly became apparent that chemistry could often proceed differently (faster polymerization rates, higher molecular weights, enhanced yields, etc.) in ILs. Providing a review of the field of polymerization in ionic liquids, with particular attention to developments over the past several years, is the subject of this chapter. The reader is referred to earlier reviews in this field for additional details on work in this area.
ILs in Conventional Free Radical Polymerization
Free radical polymerization, because of its compatibility with a wide range of monomers having different types of functional groups, is one of the most widely used polymerization techniques. Free radical solution polymerization is of great commercial importance as dilution of radical polymerizations with a solvent, typically a VOC, is effective in controlling viscosity and the exotherm accompanying polymerization. Hong et al. noted large increases in the rate of polymerization and much higher molecular weights for free radical polymerization of methyl methacrylate (MMA) in [BMIM]PF6 as compared to polymerizations carried out under identical conditions in VOCs. These effects were attributed at least in part to the high viscosity of the polymerization medium. A "diffusion-controlled termination" mechanism was proposed to explain the decreased rate of chain termination in these viscous systems. A decrease in termination rate could explain a simultaneous increase in the rate of polymerization and molecular weight. In contrast to variations in the rate of polymerization and molecular weight, the polymers synthesized in RTILs have similar glass transition temperatures and microstructures as compared to those obtained in benzene or in bulk, based upon thermal analysis and 13C-NMR experiments. Since then, other groups have reported similar behavior, high molecular weights and rapid polymerization rates, for MMA and other methacrylates in [BMIM]pF6. Harrison et al. used pulse laser polymerization (PLP) techniques to polymerize MMA in [BMIM]pF. They found that both the propagation and termination rates, kp and kt, respectively, were strongly affected by the presence of [BMIM]PF6. They attributed the increase in propagation rate to the high polarity of the ionic liquid solution, which reduces the activation energy of propagation via charge–transfer interactions. The termination rate decrease was attributed to the increased viscosity of the polymerization medium. Both the increase of kp and the decrease of kt combine to account for a ten-fold increase in the overall rate of polymerization. Subsequent PLP studies have reported kt to be decreased by an order of magnitude and kp to be increased by a factor of 4 for free radical polymerization in ILs.
Polenz and co-workers recently studied the polymerization of MMA co-initiated by imine bases and found that the reaction was accelerated greatly by even a trace amount of IL. They demonstrated that the polymerization proceeded via a free radical mechanism, with the addition of IL decreasing the activation energy of polymerization and increasing the rate of polymerization. These effects were attributed to interactions between the IL and the imine base. Cheng et al. studied free radical polymerization of acrylonitrile in [BMIM]BF4 using AIBN as an initiator. This team found that ionic liquids are excellent media for obtaining high molecular weight polymers. They attribute this finding to the low chain transfer constants for ILs and their ability to stabilize growing radical chain ends. Puttick et al. used NMR to investigate nanoscale domains formed in dialkylimidazolium ILs. When polymerizing MMA in this type of IL, it was shown that the reactants and intermediates have different affinities for nanodomains that form within the IL. Segregation of different species within these domains accounts for the unusually high polymerization rates and increased molecular weights.
Many early studies on free radical polymerization in ILs used [BMIM]PF6 due to its commercial availability and ease of synthesis. However, [BMIM]PF6 can hydrolyze to generate toxic HF, and it is desirable to use more benign ILs in polymer systems. Zhang and co-workers measured the viscosity and polarity of many different ILs containing a wide range of cations and anions, and attempted to correlate these physical properties with their behavior in the free radical polymerization of styrene and MMA. This study, which limited polymer conversion in order to avoid the Trommsdorf effect, showed no trends between the viscosity or polarity of the IL and the polymerization rate or molecular weight of the polymer. Strehmel and co-workers studied free radical polymerization of n-butylmethacrylate in a wide range of ILs, imidazolium, pyridinium, and alkyl ammonium salts. They reported that the use of ILs results in higher molecular weights, which can even exceed those obtained in bulk radical polymerization. They also found increased glass transition temperatures (in contrast with prior studies) and reported improved thermal stability, although the microstructure (tacticity) reportedly did not change. Their results show that increased molecular weight is a general phenomenon observed for free radical polymerization in ILs. They also found that high molecular weights are favored by use of high viscosity imidazolium salts or by conducting polymerizations close to the melting temperature of the IL. They hypothesized that this behavior is due to locally ordered structures in the ILs, which favor propagation (their hypothesis is supported by later work, ref. 35). This team later studied copolymerization of nonpolar and zwitterionic methacrylates in ILs as well as the use of 1-alkyl-3-methylimidazolium tosylates as solvents for polymerization of n-butylmethacrylate and styrene, where high yields and high molecular weights were obtained. Styrene polymerization by iron-containing imidazolium-based ILs was recently reported. The ILs showed high catalytic activity, with 2-3 catalytic species being present as evidenced by very broad multimodal molecular weight distributions.
IL gels for ionic transport and separations were synthesized by free radical copolymerization of 2-acrylamido-2-methyl-1-propanesulfonic acid and bisacrylamide in 1-ethyl-3-methylimidazolium ethylsulfate. Improved grafting of polystyrene onto carbon black was reported by in situ free radical polymerization of styrene in the presence of carbon black. The improvement was believed to be due to the high viscosity of the ionic liquid solvent. New ILs featuring hydrolytically stable anions have been studied. ILs featuring four anions, tris(pentafluoroethyl)trifluorophosphate, trifluoromethane-sulfonate, trifluoroacetate, and tetracyanoborate, were used as solvents in free radical polymerization, and the nature of the anion had a significant effect on the polymerization process. Free radical polymerization of vinyl fluoride was recently reported to be enhanced through polymerization in ILs. ILs containing alkylimidazolium cations and fluorinated anions gave poor conversions but polymerization in the presence of the lithium salts of the anions gave high conversions and high molecular weights at low pressures.
The use of ILs in free radical photopolymerization has been investigated by Dietlin and co-workers. The efficiency of four Type I photoinitiators in ILs was investigated by kinetics, spectroscopic and photolysis studies. polymerizations initiated by three of the photoinitiators were faster in ILs, but in the fourth case there was a significant decrease in reaction rate. This result underlines the need to match the photoinitiator to the ionic liquid. Addition of lithium bistriflimide RTIL to 1-vinylimidazole significantly improved both the monomer conversion and photopolymerization rate, likely due to coordination between the imidazole and the Li+ cation. Photopolymerization of PEG-methacrylate was investigated in [BMIM]BF4 and 1-ethyl-3-methylimidazolium trifluoroacetate in order to probe the effect of the IL on the propagation and termination rates. The use of [BMIM]BF4 gave a greater increase in kp due to its stronger Coulombic cation-anion attractions and the lower H-bond accepting ability of the BF4- anion. The reductions in kt were believed to be caused by the high viscosities of the systems explored.
The insoluble nature of some polymers in ILs creates the opportunity to make diblock copolymers by simple sequential monomer addition. Zhang and co-workers synthesized PS-b-PMMA by sequential addition of styrene, then MMA, in [BMIM]PF6 through conventional free radical polymerization using benzoyl peroxide (BPO) as an initiator. The PS first block gradually precipitated out when the conversion reached around 50% due to the insolubility of PS in [BMIM]PF6. The collapsed PS coils wrapped around the macroradicals, resulting in extended lifetimes due to diminished termination. Unreacted styrene was removed using a high vacuum line. After adding MMA, a diblock copolymer was formed at room temperature, although the re-initiation was not 100% (see Figure 1.2). Reversing the polymerization sequence resulted in a PMMA homopolymer.
The effect of IL solvents on reactivity ratios and sequence length distributions for statistical free radical copolymerization has been extensively studied. The calculated reactivity ratios for St and MMA (rSt = 0.381 ± 0.02 and rMMA = 0.464 ± 0.02) in [BMIM]PF6 are significantly different from those (rSt = 0.54 ± 0.04 and rMMA = 0.50 ± 0.04) in benzene at 60 °C. The "boot-strap" model, polarity of the solvents, interaction between solvent and monomers, viscosity, and system heterogeneity all possibly contribute to the different reactivity ratios observed in ILs.
1.3 ILs in Controlled Free Radical Polymerizations
Over the past 25 years there has been tremendous interest, primarily in academia, in controlled radical polymerizations. These systems are sometimes called "living radical polymerizations", but they are not truly living systems in the manner that some anionic polymerizations are living (the latter can be truly termination-free). Controlled radical polymerizations employ an equilibrium between dormant chain ends and active radical chain ends, with the equilibrium heavily in favor of the dormant species, in order to minimize termination in the system, which occurs through radical coupling and/or disproportionation. The result is the ability to target precise molecular weights and obtain polymers with relatively narrow molecular weight distributions, and the ability to synthesize block copolymers by sequential monomer addition. There are three main ways of achieving this equilibrium, nitroxide mediated polymerization (NMP), atom transfer radical polymerization (ATRP), and reversible addition–fragmentation chain transfer (RAFT) polymerization. These polymerization techniques have been thoroughly reviewed.
The first study of a controlled radical polymerization was carried out by Carmichael and co-workers. They conducted ATRP of MMA using ethyl-2-bromoisobutyrate as an initiator and CuBr/N-propyl-2-pyridylmethanimine as the catalyst in [BMIM]PF6. The reaction was found to be faster as compared to the same reaction carried out in VOCs, with narrow polydispersity indices (1.30–1.43). By extraction with toluene, the polymer was separated from the polymerization solution. The copper catalyst remained in the IL phase, which allowed catalyst recycling. The use of the RTIL medium also eliminated the need for post-purification to eliminate residual copper salts, which entails passing the polymer solution through purification columns. Carmichael and co-workers attributed this polymerization behavior to the increased polarity of ionic liquids, because a similar increase in the rate has been observed with other polar/coordinating solvents.
Biedron and Kubisa reported ATRP in [BMIM]PF6 for various alkyl acrylates (methyl, butyl, hexyl, and dodecyl). The resulting polymers ranged from soluble to insoluble in this IL depending on the length of the alkyl substituent. For the heterogeneous systems, the alkyl acrylate formed an upper monomer phase while the CuBr/pentamethyldiethylenetriamine (PMDETA) catalyst remained in the lower IL phase. Methyl acrylate (MA) and poly(methyl acrylate) (PMA) are miscible with [BMIM]PF6 and form a homogeneous polymerization reaction mixture where all reactions proceed in one phase. For the three other acrylates, the growing macromolecular chains react with the monomer at the interface but reside predominantly in the monomer phase during polymerization. All the polymerizations were reasonably well-controlled. Heterogeneous ATRP facilitates easy separation of polyacrylate from the IL phase after the reaction, with less copper catalyst contamination and reduction of side reactions due to the absence of catalyst in the upper monomer phase, as compared to bulk ATRR It was discovered by this same group that a chiral IL (1-(R-(+)-2'-methylbutyl)-3-methylimidazolium hexafluoro-phosphate) could regulate the stereoregularity of the polymer.
Sarbu and Matyjaszewski used both iron and copper halide catalyst systems for ATRp in a range of 1-butyl-3-methylimidazolium ionic liquids. In iron-mediated ATRp, no added ligand was necessary for controlled polymerization of MMA although both the initiation and polymerization rates were low. Systems without organic ligands were effective only in a phosphonate ionic liquid for copper-mediated ATRp of MMA, and a ligand was also necessary in ionic liquids with halide or carbonate anions. ATRp in ionic liquids is characterized by low initiation efficiency. This can be attributed to the high concentration of the catalyst in the IL phase, thus generating a high concentration of free radicals which can undergo termination. The initiator efficiency was improved by employing macroinitiators, which show a greatly reduced tendency to diffuse into the IL phase. An advantage in these systems is that the catalyst can be readily regenerated after polymerization.
(Continues…)
Excerpted from "Polymerized Ionic Liquids"
by .
Copyright © 2018 The Royal Society of Chemistry.
Excerpted by permission of The Royal Society of Chemistry.
All rights reserved. No part of this excerpt may be reproduced or reprinted without permission in writing from the publisher.
Excerpts are provided by Dial-A-Book Inc. solely for the personal use of visitors to this web site.