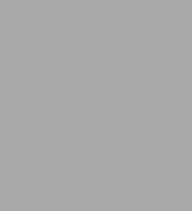
Petrophysics: Theory and Practice of Measuring Reservoir Rock and Fluid Transport Properties
976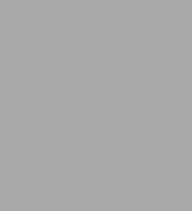
Petrophysics: Theory and Practice of Measuring Reservoir Rock and Fluid Transport Properties
976eBook
Available on Compatible NOOK devices, the free NOOK App and in My Digital Library.
Related collections and offers
Overview
Product Details
ISBN-13: | 9780123838490 |
---|---|
Publisher: | Elsevier Science |
Publication date: | 09/30/2011 |
Sold by: | Barnes & Noble |
Format: | eBook |
Pages: | 976 |
File size: | 15 MB |
Note: | This product may take a few minutes to download. |
About the Author
Erle C. Donaldson began his career as a pilot plant project manager for Signal Oil and Gas Research in Houston, Texas. Later he joined the U.S. Bureau of Mines Petroleum Research Center in Bartlesville, Oklahoma, as a project manager of subsurface disposal and industrial wastes and reservoir characterization; when the laboratory was transferred to the U.S. Department of Energy, Dr. Donaldson continued as chief of petroleum reservoir characterization. When the laboratory shifted to private industry for operations, he joined the faculty of the School of Petroleum and Geological Engineering at the University of Oklahoma as associate professor. Since retiring from the university in 1990, he has consulted for various oil companies, universities, and U.S. agencies including: the Environmental Protection Agency, the U.S. Navy Ordinance Center, King Fahd Research Institute of Saudi Arabia, and companies in the U.S., Brazil, Venezuela, Bolivia, and Thailand.
Read an Excerpt
Petrophysics
Theory and Practice of Measuring Reservoir Rock and Fluid Transport Properties
By Djebbar Tiab, Erle C. Donaldson
Elsevier
Copyright © 2012 Elsevier Inc.All rights reserved.
ISBN: 978-0-12-383849-0
CHAPTER 1
Introduction to Mineralogy
Petrophysics is the study of rock properties and their interactions with fluids (gases, liquid hydrocarbons, and aqueous solutions). The geologic material forming a reservoir for the accumulation of hydrocarbons in the subsurface must contain a three-dimensional network of interconnected pores in order to store the fluids and allow for their movement within the reservoir. Thus, the porosity of the reservoir rocks and their permeability are the most fundamental physical properties with respect to the storage and transmission of fluids. Accurate knowledge of these two properties for any hydrocarbon reservoir, together with the fluid properties, is required for efficient development, management, and prediction of future performance of the oilfield.
The purpose of this book is to provide a basic understanding of the physical properties of porous geologic materials, and the interactions of various fluids with the interstitial surfaces and the distribution of pores of various sizes within the porous medium. Procedures for the measurement of petrophysical properties are included as a necessary part of this text. Applications of the fundamental properties to subsurface geologic strata must be made by analyses of the variations of petrophysical properties in the subsurface reservoir.
Emphasis is placed on the testing of small samples of rocks to uncover their physical properties and their interactions with various fluids. A considerable body of knowledge of rocks and their fluid flow properties has been obtained from studies of artificial systems such as networks of pores etched on glass plates, packed columns of glass beads, and from outcrop samples of unconsolidated sands, sandstones, and limestones. These studies have been used to develop an understanding of the petrophysical and fluid transport properties of the more complex subsurface samples of rocks associated with petroleum reservoirs. This body of experimental data and production analyses of artificial systems, surface rocks, and subsurface rocks makes up the accumulated knowledge of petrophysics. Although the emphasis of this text is placed on the analyses of small samples, the data are correlated to the macroscopic performance of the petroleum reservoirs whenever applicable. In considering a reservoir as a whole, one is confronted with the problem of the distribution of these properties within the reservoir and its stratigraphy. The directional distribution of thickness, porosity, permeability, and geologic features that contribute to heterogeneity governs the natural pattern of fluid flow. Knowledge of this natural pattern is sought to design the most efficient injection—production system for economy of energy and maximization of hydrocarbon production.
Petrophysics is intrinsically bound to mineralogy and geology because the majority of the world's petroleum occurs in porous sedimentary rocks. The sedimentary rocks are composed of fragments of other rocks derived from mechanical and chemical deterioration of igneous, metamorphic, and other sedimentary rocks, which is constantly occurring. The particles of erosion are frequently transported to other locations by winds and surface streams and deposited to form new sedimentary rock structures. Petrophysical properties of the rocks depend largely on the depositional environmental conditions that controlled the mineral composition, grain size, orientation or packing, amount of cementation, and compaction.
MINERAL CONSTITUENTS OF ROCKS—A REVIEW
The physical properties of rocks are the consequence of their mineral composition. Minerals are defined here as naturally occurring chemical elements or compounds formed as a result of inorganic processes. The chemical analysis of six sandstones by emission spectrography and X-ray dispersive scanning electron microscopy showed that the rocks are composed of just a few chemical elements. Analysis of the rocks by emission spectroscopy yielded the matrix chemical composition since the rocks were fused with lithium to make all of the elements soluble in water, and then the total emission spectrograph was analyzed. The scanning electron microscope X-ray, however, could only analyze microscopic spots on the broken surface of the rocks. The difference between the chemical analysis of the total sample and the spot surface analysis is significant for consideration of the rock—fluid interactions. The presence of the transition metals on the surface of the rocks induces preferential wetting of the surface by oil through Lewis acid—base type reactions between the polar organic compounds in crude oils and the transition metals exposed in the pores. The high surface concentration of aluminum reported in Table 1.1 is probably due to the ubiquitous presence of clay minerals in sandstones.
The list of elements that are the major constituents of sedimentary rocks (Table 1.1) is confirmed by the averages of thousands of samples of the crust reported by Foster (Table 1.2). Just eight elements make up 99% (by weight) of the minerals that form the solid crust of the Earth; these are the elements, including oxygen, listed in the first seven rows of Table 1.1 from analysis of six sandstones. Although the crust appears to be very heterogeneous with respect to minerals and types of rocks, most of the rock-forming minerals are composed of silicon and oxygen together with aluminum and one or more of the other elements listed in Table 1.2.
The chemical compositions and quantitative descriptions of some minerals are listed in Tables 1.3 and 1.4. Some of the minerals are very complex and their chemical formulas differ in various publications; in such cases the most common formula reported in the list of references was selected.
IGNEOUS ROCKS
Igneous rocks (about 20% of all rocks) are the product of the cooling of molten magma intruding from below the mantle of the crust. Igneous (plutonic) rocks are divided into three easily recognizable rocks, which are subdivided by the rate of cooling (Figure 1.1). The granites are intrusive rocks that cooled slowly (at high temperature) below the surface, whereas gabbro is a rock resulting from more rapid (low temperature) cooling in the subsurface. Diorite is a rock that cooled below the surface at a temperature intermediate between that of granite and gabbro. The minerals differentiate during the slow cooling, forming large recognizable, silica-rich crystals with a rough (phaneritic) texture.
The second classification is extrusive (volcanic) rock that has undergone rapid cooling on or near the surface, forming silica-poor basaltic rocks. Rhyolite, or felsite, is light colored and estimated to be produced on the surface at a lower temperature than the darker andesite that formed at a temperature intermediate between that of rhyolite and the dark-colored basalt. As a result of rapid cooling on the surface, these rocks have a fine (alphanitic) texture with grains that are too small to be seen by the unaided eye.
Minerals precipitating from melted magma, or melt, do not crystallize simultaneously. Generally, a single mineral precipitates first and, as the melt cools slowly, this is joined by a second, third, and so on; thus the earlier-formed minerals react with the ever-changing melt composition. If the reactions are permitted to go to completion, the process is called equilibrium crystallization. If the crystals are completely or partially prevented from reacting with the melt (by settling to the bottom of the melt or by being removed), fractional crystallization takes place and the final melt composition will be different from that predicted by equilibrium crystallization. The mechanism by which crystallization takes place in a slowly cooling basaltic melt was summarized by Bowen as two series of simultaneous reactions; after all of the ferro-magnesium minerals are formed, a third series of minerals begins to crystallize from the melt. From laboratory experiments, Bowen discovered that the first two series of reactions have two branches.
The Bowen series of specific crystallization occurs only for some basaltic magmas (a variety of different reaction series occur within different melts), but the processes discussed by Bowen are significant because they explain the occurrence of rocks with compositions different from that of the original melted magma.
METAMORPHIC ROCKS
The metamorphic rocks (about 14% of all rocks) originate from mechanical, thermal, and chemical changes of igneous rocks. Mechanical changes on or near the surface are due to the expansion of water in cracks and pores, tree roots, and burrowing animals. If the igneous rocks undergo deep burial due to subsidence and sedimentation, the pressure exerted by the overlying rocks, shear stress from tectonic events, and the increased temperature result in mechanical fracturing. When unequal shear stress is applied to the rocks as a result of continental motion of other force fields, cleavage of the rocks (fracturing) occurs; alternatively, slippage of a regional mass of rocks and sediments (faulting) occurs. The pressure produced by overlying rocks is approximately 1.0 psi per foot of depth (21 kPa per meter of depth). The changes induced by overburden pressure occur at great depth in conjunction with other agents of metamorphism.
Chemical metamorphosis of igneous intrusive rocks, aided by high pressure, temperature, and the presence of water, results in chemical rearrangement of the elements into new minerals. This produces foliated rocks with regularly oriented bands of mineral grains because the new crystals tend to grow laterally in the directions of least stress. This chemical metamorphism of granite yields gneiss: a foliated granite with large recognizable crystals of banded feldspars. Gabbro changes to amphibolite, whose main constituent is the complex mineral known as hornblende.
The chemical metamorphosis of the extrusive rocks, rhyolite, basalt, etc., produces changes to easily recognizable rocks. Rhyolite, light-colored volcanic rock, undergoes change principally to three types of metamorphic rocks, depending on the environmental conditions inducing the changes: (1) gneiss, which has foliated bands of feldspars; (2) schist or mica; and (3) slate, which is a fine-grained smooth-textured rock. Basalt, the dark-colored volcanic rock, produces two main types of metamorphic rocks: (1) amphibolite and (2) greenschist, or green mica, as illustrated in Figure 1.1.
On a regional scale, the distribution pattern of igneous and metamorphic rocks is belt-like and often parallel to the borders of the continents. For example, the granitic rocks that form the core of the Appalachian mountains in eastern United States are parallel to the east coast and those in the Sierra Nevada are parallel to the west coast.
Igneous and metamorphic rocks are not involved in the origin of petroleum as source rocks. In some cases, they do serve as reservoirs, or parts of reservoirs, where they are highly fractured or have acquired porosity by surface weathering prior to burial and formation into a trap for oil accumulated by tectonic events.
SEDIMENTARY ROCKS
All of the sedimentary rocks (about 66% of all rocks) are important to the study of petrophysics and petroleum reservoir engineering. It is possible to interpret them by considering the processes of rock degradation. The principal sedimentary rocks may be organized according to their origin (mechanical, chemical, and biological) and their composition, as illustrated in Table 1.3.
Mechanical weathering is responsible for breaking large preexisting rocks into small fragments. The most important mechanism is the expansion of water upon freezing, which results in a 9% increase of volume. The large forces produced by freezing of water in cracks and pores results in fragmentation of the rocks. Mechanical degradation of rocks also occurs when a buried rock is uplifted and the surrounding overburden is removed by erosion. The top layers of the rock expand when the overburden pressure is relieved, forming cracks and joints that are then further fragmented by water. Mechanical weathering produces boulder-size rocks, gravel, sand grains, silt, and clay from igneous and metamorphic rocks. These fragments remain in the local area, or they may be transported by winds and water to other sites to enter into the formation of conglomerates, sandstones, etc., as shown in Table 1.3.
(Continues...)
Excerpted from Petrophysics by Djebbar Tiab, Erle C. Donaldson. Copyright © 2012 Elsevier Inc.. Excerpted by permission of Elsevier.
All rights reserved. No part of this excerpt may be reproduced or reprinted without permission in writing from the publisher.
Excerpts are provided by Dial-A-Book Inc. solely for the personal use of visitors to this web site.
Table of Contents
Contents
Preface.................... xiii
About the Authors.................... xv
Acknowledgment.................... xvii
Units.................... xix
1. Introduction to Mineralogy....................
2. Introduction to Petroleum Geology....................
3. Porosity and Permeability....................
4. Formation Resistivity and Water Saturation....................
5. Capillary Pressure....................
6. Wettability....................
7. Applications of Darcy's Law....................
8. Naturally Fractured Reservoirs....................
9. Effect of Stress on Reservoir Rock Properties....................
10. Reservoir Characterization....................
11. FluidÀRock Interactions....................
12. Basic Well-Log Interpretation....................
Appendix.................... 829
Index.................... 913
What People are Saying About This
Provides users with a resource that will help them understand reservoir performance, including shale