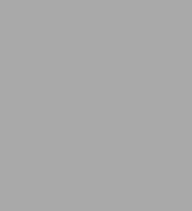
Nanoceramics in Clinical Use: From Materials to Applications
331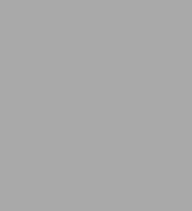
Nanoceramics in Clinical Use: From Materials to Applications
331Hardcover(2nd ed.)
-
SHIP THIS ITEMNot Eligible for Free ShippingPICK UP IN STORECheck Availability at Nearby Stores
Available within 2 business hours
Related collections and offers
Overview
About the Author
Daniel Arcos was born in Madrid, Spain, in 1971. He is holding a postdoctoral research position ('Ramón y Cajal' grant) at the Universidad Complutense de Madrid, Spain. Since completing his PhD in 2002 on the synthesis and evaluation of bioactive glasses and glass-ceramics, he has worked on the structural studies of silicon containing hydroxyapatites. Currently, his research is focused on mesoporous materials for biomedical applications
Read an Excerpt
Nanoceramics in Clinical Use
From Materials to Applications
By Maria Vallet-Regi, Daniel Arcos Navarrete
The Royal Society of Chemistry
Copyright © 2016 M. Vallet-Regí and D. Arcos NavarreteAll rights reserved.
ISBN: 978-1-78262-104-1
CHAPTER 1
Biological Apatites in Bone and Teeth
1.1 Hard-Tissue Biomineralization: How Nature Works
Most biominerals are inorganic/organic composite materials. This is also the case for the bones and teeth of all vertebrates, which are formed by the combination of an inorganic calcium phosphate phase and an organic matrix (Figure 1.1). The inorganic component is a nanocrystalline solid with apatite structure and the chemical composition of a carbonated, basic calcium phosphate, hence it can be termed a carbonate-hydroxy-apatite. It comprises 65% of the total bone mass, with the remaining mass formed by organic matter and water. The benefits that the inorganic component brings to this combination are toughness and the ability to withstand pressure.
In contrast, the organic matrix formed by collagen fibres, glycoproteins and mucopolysaccharides provides elasticity and resistance to stress, bending and fracture. Such symbiosis of two very different compounds, with markedly different properties, confers to the final product (i.e. the biomineral) some properties that would be unattainable for each of its individual components per se. This is a fine example in nature of the advantages that a composite material can exhibit, reaching new properties with added value. In fact due to this evidence, a large portion of the field of modern materials science is currently focused on the development of composite materials.
1.1.1 Bone Formation
The bone exhibits some physical and mechanical properties which are rather unusual. It is able to bear heavy loads, to withstand large forces and to flex without fracture within certain limits. Besides this, the bone also acts as an ion buffer both for cations and anions. From the material point of view, bone could be simplified as a three-phase material formed by organic fibers, an inorganic nanocrystalline phase and a bone matrix. Its unique physical and mechanical properties are the direct consequence of intrinsic atomic and molecular interactions within this very particular natural composite material.
Bone is not uniformly dense. It has a hierarchical structure. Due to its true organic–inorganic composite nature, it is able to adopt different structural arrangements with singular architectures, determined by the properties required from it depending on its specific location in the skeleton. Generally speaking, most bones exhibit a relatively dense outer layer, known as cortical or compact bone, which surrounds a less dense and porous layer, termed trabecular or spongy bone, which is in turn filled with a jelly tissue: the bone marrow. This complex tissue is the body deposit of non-differentiated trunk cells, the precursors of most repairing and regenerating cells produced after formation of the embryonic subject. The bone fulfils critical functions in terms of structural material and ion reservoir. Both functions strongly depend on the size, shape, chemical composition and crystalline structure of the mineral phase, and also on the mineral distribution within the organic matrix.
The main constituents of bone are: water; a mineral phase, calcium phosphate in the form of carbonated apatite with low crystallinity and nanometric dimensions, which accounts for roughly two-thirds of the bone's dry weight; and an organic fraction, formed of several proteins, among which type I collagen is the main component, which represents approximately the remaining one-third of bone dry weight. The other intervening proteins, such as proteoglycans and glycoproteins, total more than 200 different proteins, known as non-collagen proteins; however, their total contribution to the organic constituent falls below 10% of the organic fraction. These bone constituents are hierarchically arranged, with at least five levels of organization. At the molecular level, the polarized triple helix of tropocollagen molecules are grouped in microfibers, with small cavities between their edges, where small apatite crystals – ~5 nm x 30 nm sized – nucleate and grow. These microfibers unite to form larger fibers which constitute the microscopic units of bone tissue. These fibers are arranged according to different structural distributions to form the full bone.
It was traditionally believed that the inorganic phase was mainly amorphous calcium phosphate which, in the aging process, evolved towards nanocrystalline hydroxyapatite. However, results of solid-state 31P nuclear magnetic resonance spectroscopy evidenced that the amorphous phase is never present in large amounts during the bone development process. Besides, this technique did detect acid phosphate groups. Phosphate functions correspond to proteins with O-phosphoserine and O-phosphothreonine groups, which are probably used to link the inorganic mineral component and the organic matrix. Phosphoproteins are arranged in the collagen fibers so that Ca2+ can be bonded at regular intervals, in agreement with the inorganic crystal structure, hence providing a repeating condition which leads to an ordered sequence of the same unit, i.e. the crystallinity of the inorganic phase. The cells responsible for most of the assembling process are termed osteoblasts. When the main assembling process is completed, the osteoblasts keep differentiating in order to form osteocytes, which are responsible for the bone maintenance process. The controlled nucleation and growth of the mineral take place at the microscopic voids formed in the collagen matrix. The type I collagen molecules, segregated by the osteoblasts, are grouped in microfibers with a specific tertiary structure, exhibiting a periodicity of 67 nm and 40 nm cavities or orifices between the edges of the molecules. These orifices constitute microscopic environments with free Ca2+ and PO43- ions, as well as groups of side chains eligible for bonding, with a molecular periodicity that allows the nucleation of the mineral phase in a heterogeneous fashion. Ca2+ ions deposited and stored in the skeleton are constantly renewed with dissolved calcium ions. The bone growth process can only be produced under a relative excess of Ca2+ and its corresponding anions, such as phosphates and carbonates, at the bone matrix. This situation is achieved due to the action of efficient ATP-powered ionic pumps, such as Ca2+ ATPases for active transportation of calcium. In terms of physiology, carbonate and phosphate are present in the forms of HCO3-, HPO42- and H2PO4- anions. When incorporated to the bone, the released protons can move throughout the bone tissue and leave the nucleation and mineralization area. The nucleation of thin, platelet-shaped apatite crystals takes place at the bone within discrete spaces inside the collagen fibers, hence restricting a potential primary growth of these mineral crystals, and imposing their discrete and discontinuous quality (Figure 1.2).
Calcium phosphate nanocrystals in bone, formed as mentioned at the spaces left between the collagen fibers exhibit the particular feature of being monodispersed and nanometer-sized platelets of carbonate-hydroxyl-apatite. There is no other mineral phase present, and the crystallographic axis c of these crystals is arranged in parallel to the collagen fibers and to the largest dimension of the platelet. In the mineral world, the thermodynamically stable form of calcium phosphate under standard conditions is the hydroxyapatite. Generally speaking, this phase grows in needle-like forms, with the c axis parallel to the needle axis. Figure 1.3 shows the crystalline structure of hydroxyapatite, Ca10(PO4)6(OH)2, which belongs to the hexagonal system, space group P63/[m and lattice parameters a = 9.423 Å and c = 6.875 Å.
Besides the main ions Ca2+, PO43- and OH-, the composition of biological apatites always includes CO32- at ~4.5%, and also a series of minority ions, usually including Mg2+, Na+, K+, Cl- and F-. These substitutions modify the lattice parameters of the structure as a consequence of the different size of the substituting ions, as depicted in Figure 1.3. This is an important difference between minerals grown in an inorganic or biological environment.
The continuous formation of bone tissue is performed at a peripheral region, formed by an external crust and an internal layer with connective tissue and osteoblast cells. These osteoblasts are phosphate-rich and exude a jelly-like substance, the osteoid. Due to the gradual deposit of inorganic material, this osteoid becomes stiffer and the osteoblasts are finally confined and transformed in bone cells, the osteocytes. The bone transformation mechanism, and the ability to avoid an excessive bone growth, are both catered for by certain degradation processes which are performed simultaneously to the bone formation. The osteoclasts, which are giant multinucleated cells, are able to catabolyze the bone purportedly using citrates as chelating agent. The control of the osteoclast activity is verified through the action of the parathyroid hormone, a driver for demineralization, and its antagonist, tireocalcitonin.
The collagen distribution with the orifices previously described is necessary for the controlled nucleation and growth of the mineral, but it might not suffice. There are conceptual postulations of various additional organic components, such as the phosphoproteins, as an integral part of the nucleation core and hence directly involved in the nucleation mechanism. Several immuno-cyto-chemical studies of bone, using techniques such as optical microscopy and high-resolution electron microscopy have clearly shown that the phosphoproteins are restricted, or at least largely concentrated at the initial mineralization location, intimately related to the collagen fibers. It seems that the phosphoproteins are enzymatically phosphored prior to the mineralization.
The crystallization of the complex and hardly soluble apatite structures evolves favourably through the kinetically controlled formation of metastable intermediate products. Under in vitro conditions, amorphous calcium phosphate is transformed into octacalcium phosphate which, in turn, evolves to carbonate hydroxyapatite; at lower pH values, the intermediate phase seems to be dehydrated dicalcium phosphate.
The mechanisms of bone formation are highly regulated processes, which seem to verify the following statements:
- Mineralization is restricted to those specific locations where crystals are constrained in size by a compartmental strategy.
- The mineral formed exhibits specific chemical composition, crystalline structure, crystallographic orientation and shape. The chemical phase obtained is controlled during the stages of bone formation. In vertebrates, said chemical phase is a hydroxyl-carbonate-apatite, even though the thermodynamically stable form of calcium phosphate in the world of minerals, under standard conditions, is hydroxyapatite.
- Since the mineral deposits onto a biodegradable organic support, complex macroscopic forms are generated with pores and cavities. The assembling and remodelling of the structure are achieved by cell activity, which builds or erodes the structure layer by layer.
Without a careful integration of the whole process, bone formation would be an impossible task. The slightest planning mistake by the body, for instance in its genetic coding or cell messengers, is enough to provoke building errors that would weaken the osseous structure.
The hard tissues in vertebrates are bones and teeth. The differences between them reside in the amounts and types of organic phases present, the water content, the size and shape of the inorganic phase nanocrystals and the concentration of minor elements present in the inorganic phase, such as CO32-, Mg2+, Na+, etc. The definitive set of teeth in higher order vertebrates has an outer shell of dental enamel that, in an adult subject, does not contain any living cells. Up to 90% of said enamel can be inorganic material, mainly carbonate-hydroxyl-apatite. Enamel is the material that undergoes more changes during the tooth development process. At the initial stage, it is deposited with a mineral content of only 10–20%, with the remaining 80–90% proteins and special matrix fluids. In subsequent development stages, the organic components of the enamel are almost fully replaced by inorganic material. The special features of dental enamel when compared with bone material are its much larger crystal domains, with prismatic shapes and strongly oriented, made of carbonate-hydroxyl-apatite (Figure 1.4). There is no biological material that could be compared to enamel in terms of hardness and long life. However, it cannot be regenerated.
The bones, the body-supporting scaffold, can exhibit different types of integration between organic and inorganic materials, leading to significant variations in their mechanic properties. The ratio of both components reflects the compromise between toughness (high inorganic content) and resiliency or fracture strength (low inorganic content). All attempts to synthesize bone replacement materials for clinical applications featuring physiological tolerance, biocompatibility and long-term stability have, up to now, had only relative success; the superiority and complexity of the natural structure shows where, for instance, the human femur can withstand loads of up to 1650 kg.
The bones of vertebrates, as opposed to the shells of molluscs, can be considered as living biominerals, since there are cells inside them under permanent activity. Bone also constitutes a storage and hauling mechanism for two essential elements, phosphorus and calcium, which are mainly stored in the bones. Most of what has been described up to this point regarding the nature of bone tissue, could be summed up stating that the bone is a highly structured porous matrix, made of nanocrystalline and non-stoichiometric apatite, calcium deficient and carbonated, intertwined with collagen fibers and blood vessels.
Bone functions are controlled by a series of hormones and bone growth factors. Figure 1.5 attempts to depict these phenomena in a projection from our macro scale point of view, to the 'invisible' nano scale.
Bone's rigidity, resistance and toughness are directly related to its mineral content. Although resistance and rigidity increase linearly with the mineral content, toughness does not exhibit the same trend, hence there is an optimum mineral concentration which leads to a maximum in bone toughness. This tendency is clearly the reason why the bone exhibits a restricted amount of mineral within the organic matrix. But there are other issues affecting the mechanical properties of bone, derived from the microstructural arrangement of its components. In this sense, the three main components of bone exhibit radically different properties. From this point of view, the biomineral is clearly a composite. The organic scaffold exhibits a fibrous structure with three levels: the individual triple helix molecules, the small fibrils and its fiber-forming aggregates. These fibers can be packed in many different ways; they host the platelet-shaped hydroxyl-carbonate-apatite crystals. In this sense, the bone could be described as a composite reinforced with platelets, but the order–disorder balance determines the microstructure and, as a consequence, the mechanical properties of each bone. In fact, bones from different parts of the body show different arrangements, depending on their specific purpose.
Bone crystals are extremely small, with an average length of 50 nm (in the 20–150 nm range), 25 nm in average width (10–80 nm range) and thickness of just 2–5 nm. As a remarkable consequence, a large part of each crystal is surface; hence their ability to interact with the environment is outstanding.
Apatite phase contains 4–8% in weight of carbonate, properly described as dahllite. Mineral composition varies with age and it is always calcium deficient, with phosphate and carbonate ions in the crystal lattice. The formula Ca8.3(PO4)4.3(CO)3x (HPO4)y(OH)0.3 represents the average composition of bone, where y decreases and x increases with age, while the sum x + y remains constant and equal to 1.7. Mineral crystals grow under a specific orientation, with the c axes of the crystals approximately parallel to the long axes of the collagen fibers where they are deposited. Electron microscopy techniques enabled this information to be obtained.
(Continues...)
Excerpted from Nanoceramics in Clinical Use by Maria Vallet-Regi, Daniel Arcos Navarrete. Copyright © 2016 M. Vallet-Regí and D. Arcos Navarrete. Excerpted by permission of The Royal Society of Chemistry.
All rights reserved. No part of this excerpt may be reproduced or reprinted without permission in writing from the publisher.
Excerpts are provided by Dial-A-Book Inc. solely for the personal use of visitors to this web site.