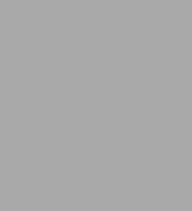
N-Heterocyclic Carbenes: From Laboratory Curiosities to Efficient Synthetic Tools
636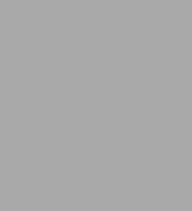
N-Heterocyclic Carbenes: From Laboratory Curiosities to Efficient Synthetic Tools
636Hardcover(2nd ed.)
-
SHIP THIS ITEMNot Eligible for Free ShippingPICK UP IN STORECheck Availability at Nearby Stores
Available within 2 business hours
Related collections and offers
Overview
About the Author
Read an Excerpt
N-Heterocyclic Carbenes
From Laboratory Curiosities to Efficient Synthetic Tools
By Silvia Díez-González
The Royal Society of Chemistry
Copyright © 2017 The Royal Society of ChemistryAll rights reserved.
ISBN: 978-1-78262-423-3
CHAPTER 1
Introduction to N-Heterocyclic Carbenes: Synthesis and Stereoelectronic Parameters
MAREIKE C. JAHNKE AND F. EKKEHARDT HAHN
1.1 Introduction
Chemists have been fascinated with carbenes for more than 150 years. The simplest member of this class of compounds, possessing a neutral divalent carbon atom and six electrons in its valence shell, is methylene, CH2. Numerous attempts to isolate methylene or related compounds failed, although "carbenic reactivity" of methylene derivatives was described in connection with cyclopropanation reactions as early as 1953.
Even if free carbenes could not be isolated, carbene complexes have been known for a long time. The first complex with a heteroatom-stabilized carbene ligand, most likely unrecognized as such, was prepared as early as 1925 by Tschugajeff (English transcription Chugaev). Tschugajeff's "red salt" 1 was obtained by the reaction of the tetrakis(methylisocyanide) platinum(II) cation with hydrazine. The "yellow salt" 2 was formed upon treatment of 1 with HCl in a reversible reaction. The determination of the molecular structures of 1 and 2 in 1970 demonstrated that these compounds were correctly described as diaminocarbene complexes (Scheme 1.1).
In 1964, Fischer prepared and characterized unambiguously the first metal carbene complex 3 obtained by nucleophilic attack of phenyl lithium at tungsten hexacarbonyl followed by O-alkylation. This was followed by Schrock's synthesis of a high oxidation state metal alkylidene complex 4 obtained by α-hydrogen abstraction from tris(neopentyl) tantalum(v) dichloride (Scheme 1.1).
Parallel to these efforts, Wanzlick tried to prepare a stable N- heterocyclic carbene by α-elimination of chloroform from 5. The free carbene, however, could not be isolated and instead its dimer, the entetraamine 6=6, was always obtained (Scheme 1.2). Wanzlick's initially postulated cleavage of the entetraamine according to 6=6 [right arrow] 2 x 6 could not be demonstrated conclusively. Cross metathesis experiments with differently N,N'-substituted entetraamines failed, excluding an equilibrium between the monomer 6 and the dimer 6=6 (Scheme 1.2).
Around 1960, it was already known that unsaturated heterocyclic azolium cations reacted in a base-catalyzed H,D-exchange reaction. Hoping that the delocalization of the six π-electrons in such derivatives might stabilize the intermediately formed carbene species, Wanzlick attempted to prepare free carbene 7 by deprotonation of tetraphenylimidazolium perchlorate with KOt-Bu [eqn (1.1)]. Again, he could not isolate free 7 but its intermediate formation was demonstrated indirectly by identification of some of its reaction products with water or with [Hg(Oac)2]. Almost three decades later, Arduengo succeeded in the preparation of free by the deprotonation method originally suggested by Wanzlick].
[FORMULA OMITTED] (1.1)
While, up to 1990, all attempts to isolate a stable N-heterocyclic carbene failed, metal complexes of unsaturated imidazol-2-ylidenes were known as early as 1968. The first complexes of this type were obtained by in situ deprotonation of imidazolium salts using mercury(II) acetate or dimethylimidazolium hydridopentacarbonylchromate(-II) followed by coordination of the carbene to the metal center (Scheme 1.3). Shortly thereafter, the stabilization of the saturated imidazolin-2-ylidene in a metal complex was described by Lappert who treated electron-rich entetraamines of type 6=6 with coordinatively unsaturated transition metal complexes to obtain complexes with imidazolin-2-ylidene ligands (Scheme 1.3).
While Bertrand and co-workers described in 1988 the stable λ3-phosphinocarbene 8,, which did not act as a ligand, Arduengo et al. prepared in 1991 the first free and stable "bottleable" N-heterocyclic carbene 9 by deprotonation of the corresponding imidazolium salt (Scheme 1.4). This deprotonation method was later supplemented by Kuhn, who intro- duced the reductive desulfurization of thiones for the preparation of stable imidazol-2[-ylidenes.
The isolation of compound 9 demonstrated that free carbenes were not invariably unstable intermediates. Its isolation initiated an intensive search for additional stable N-heterocyclic carbenes (NHCs), leading to the isolation of derivatives with different heteroatoms in the carbene ring and different N-heterocyclic ring sizes. General aspects of the synthesis of NHCsand their coordination chemistry with transition metals, coinage metals, f-block metals, Group 1 and 2 elements, main group elements and carbene-stabilized main group radicals have already been reviewed. Additional reviews deal with selected classes of polydentate ligands containing NHC donor functions and chiral NHC ligands or NHCs as organo-catalysts. Important aspects of carbene dimerization and the application of NHC complexes in different catalytic reactions, even in aqueous media, or of heterometallic NHC complexes as tandem catalyst have also been reviewed.
1.2 Electronic Structure and Stabilization of N-Heterocyclic Carbenes
Carbenes are defined as neutral compounds of divalent carbon where the carbon atom possesses only six valence electrons. If methylene, CH2, is considered the simplest carbene, a linear or bent geometry at the carbene carbon atom can be considered. The linear geometry is based on a sp-hybridized carbene carbon atom, leading to two energetically degenerated p orbitals (px, py). This geometry constitutes an extreme, and most carbenes contain a sp-hybridized carbon atom with a non-linear geometry at this atom. The energy of the non-bonding p orbital (py), conventionally called pπ pafter sp2-hybridization, practically does not change upon the sp -> sp2 transition. The sp2-hybrid orbital, normally described as the σ orbital, possesses partial s-character and is thus energetically stabilized relative to the original px orbital (Figure 1.1).
The two non-bonding electrons at the sp2-hybridized carbene carbon atom can occupy the two empty orbitals (pπ and σ) with a parallel spin orientation, leading to a triplet ground state (σ1pπ1, 3B1 state, Figure 1.1). Alternatively, the two electrons occupy the c orbital with an antiparallel spin orientation (σ2pπ0, 1A1 state). An additional, generally less stable, singlet state (σ0pπ2, 1A1 state) and an excited singlet state with an antiparallel occupation of the pπ and σ orbitals (σ1pπ1, 1B1 state) are conceivable but of no relevance for the present discussion.
The multiplicity of the ground state determines the properties and the reactivity of a carbene. Singlet carbenes possessing a filled σ and an empty pπ orbital exhibit an ambiphilic behavior, while triplet carbenes can be considered as diradicals. The multiplicity of the ground state is determined by the relative energies of the σ and pπ orbitals, as depicted in Figure 1.1. Quantum chemical calculations showed that an energy difference of about 2 eV is required for the stabilization of the singlet ground state (1A1), while an energy difference of less than 1.5 eV between the relative energies of the σ and pπ orbitals favored the triplet ground state (3B1).
Steric and electronic effects of the α substituents at the carbene carbon atom control the multiplicity of the ground state. It is generally accepted that the singlet ground state is stabilized by σ-electron withdrawing, generally more electronegative, substituents. This negative inductive effect causes a lowering of the relative energy of the non-bonding σ orbital, while the relative energy of the pπ orbital remains essentially unchanged. Substituents with σ-electron donating properties decrease the energy gap between the σ and the pπ orbital and thus stabilize the triplet ground state.
In addition, mesomeric effects play a crucial role. The substituents at the carbene carbon atom can be classified into different categories depending on their π-donor/π-acceptor properties. Singlet carbenes of type X2C:, substituted with two π donors X, are strongly bent at the carbene carbon atom. The interaction of the π-electron pairs at the α substituents with the pπ orbital at the carbene carbon atom raises the relative energy of this orbital. The relative energy of the σ orbital at the carbene carbon atom is not affected by the π-interaction. Consequently, the σ-pπ energy gap becomes larger, leading to a further stabilization of the bent singlet ground state. The interaction of the π electrons of the substituents with the pπ orbital at the carbene carbon atom leads to some extent to the formation of a four-electron three-center π-system where the X-C bonds acquire partial double bond character (Figure 1.2). Important members of this class of compounds are the dimethoxycarbenes and the dihalocarbenes. The most important singlet carbenes stabilized by two n-donors are the NHCs. The bonding situation in NHCs derived from five-membered heterocycles has been discussed in detail.
1.3 N-Heterocyclic Carbene Ligands
The isolation of the first stable NHCs and their successful use as ancillary ligands for the preparation of various metal complexes initiated an intensive search for new NHC ligands by variation of the size of the heterocycle, the heteroatoms within the cycle and the substituents at the nitrogen atoms of the heterocycle and the heterocycle itself. Access to NHCs is largely controlled by the availability of suitable NHC precursors. Most NHCs are prepared by deprotonation of azolium cations found in imidazolium, triazolium, benzimidazolium, imidazolinium or thiazolium salts or by reductive desulfurization of imidazol-, benzimidazol- and imidazolin-2-thiones. In addition, stable NHCs have also been obtained from various imidazolidines by thermally induced α-elimination reactions (Scheme 1.5). the preparation of suitable azolium salts, 2-thi-ones and imidazolines is presented in this section, followed by the description of methods for the liberation of the free NHCs from these compounds. imidazol-2-ylidenes are the most widely used NHC ligands and therefore special emphasis is placed on synthetic procedures leading to these unsaturated NHC ligands featuring a five-membered diazaheterocycle.
1.3.1 Synthesis of NHC Precursors
Several methods for the preparation of imidazolium salts 10 (Scheme 1.6) have been described. The two most common routes are the alkylation of the nitrogen atoms of imidazole and the multi-component reactions of primary amines, glyoxal and formaldehyde giving symmetrical N,N'-substituted azolium salts (Scheme 1.6a and b). The second method is particularly useful for the synthesis of imidazolium salts bearing aromatic, very bulky or functionalized N,N'-substituents. Unsymmetrically substituted imidazolium salts can be obtained by either stepwise alkylation of imidazole (Scheme 1.6a) or by combination of a multi-component cyclization with a subsequent N-alkylation reaction (Scheme 1.6c). N-Aryl imidazole derivatives can also be prepared from imidazole via a copper-catalyzed Ullman-coupling reaction. Imidazolium salts bearing two different aryl substituents at the nitrogen atoms or bisoxazoline derived imidazolium salts leading to NHC ligands with a flexible steric bulk have also been described.
The saturated imidazolinium salts of type 11 (Scheme 1.7) can be obtained by alkylation of dihydroimidazole or by the cyclization reactions between N,N'-dialkyl-α,β-ethyldiamines and orthoesters (Scheme 1.7a). A multi-component reaction leading to unsymmetrical derivatives of type 11, which is particularly interesting for the synthesis of imidazolinium salts with substituents at the C4 and C5 positions of the heterocycle, was reported by Orru and co-workers (Scheme 1.7b). The reaction of stable N-(2-iodoethyl) arylammonium salts with an amine and triethyl orthoformate was reported to yield various imidazolinium salts of type 11 (Scheme 1.7c). Precursor 12 for a N-heterocyclic diaminocarbene possessing a six-membered heterocycle was obtained from the reaction of a suitable 1,3-diaminopropane with triethyl orthoformate (Scheme 1.7d). Related compounds with an aromatic backbone were obtained from diaminonaphthalene. While these methods are based on the ring closure by introduction of a CH+ fragment, Bertrand and co-workers presented a different approach based on the reaction of a 1,3-diazaallyl anion with compounds featuring two leaving groups. Amidinium salts with six-, seven- or even eight-membered heterocycles (12-14) were obtained by this method (Scheme 1.7e), which was further developed by Cavell and others to yield a variety of derivatives with different ring sizes and substituents at the nitrogen atoms.
Alternative precursors for the synthesis of NHCs are thiourea derivatives of type 15. Kuhn and Kratz first reported a facile method for the synthesis of symmetrically substituted imidazol-2-thiones by the reaction of 3-hydroxy-2-butanone with suitable thiourea derivatives (Scheme 1.8a). Related saturated imidazolin-2-thiones 16 or benzimidazol-2-thiones 17 were obtained by reaction of aliphatic or aromatic 1,2-diamino compounds with thiophosgene (Scheme 1.8b). Unsymmetrically substituted imidazolin-2-thiones 18 were obtained by the reaction of lithium-N-lithiomethyldithiocarbamates with aldimines and ketimines, respectively (Scheme 1.8c).
N-Heterocyclic carbenes can also be prepared by α-elimination from imidazolidines (see Scheme 1.5). Differently substituted imidazolidines or benzimidazolines were prepared by addition of alkali metal alkoxides to azolium salts or by condensation of suitable diamines with benzaldehydes bearing fluorinated aromatic rings. Some remarkable diaminocarbene precursors, such as 1,3-dimethyltetrahydropyrimidin-2-ium chloride, were described by Bertrand and co-workers.
Reports on N,N'-donor-functionalized, chiral and polydentate NHCs appeared almost immediately after the reports on the preparation of the first stable NHCs. Herrmann's report on the first donor-functionalized imidazolium cations 19 and 20 (Figure 1.3) from 1996 was followed by a large number of publications dealing with imidazolium precursors for donor-functionalized carbene ligands." The preparation of NHCs from imidazolium precursors bearing acidic N-substituents such as alcohols or secondary amines was also attempted. Studies by Arnold and co-workers demonstrated that alcohol-functionalized imidazolium salts 21 (Figure 1.3) were readily accessible by nucleophilic opening of epoxides. Imidazolium salts 22 bearing N-s-amine substituents were also prepared. Fryzuk and co-workers succeeded with the synthesis of an N,N'-di(s-amine) substituted imidazolium salt. Indenyl- (23) and fluorenyl- (24) substituted imidazolium cations were also described (Figure 1.3).
A large number of differently alkylene-bridged diazolium salts of type 25 (Figure 1.4) has been described, next to the similar dibenzimidazolium salts. The phenylene-bridged diazolium salt 26 functions, depending on the transition metal used, as a suitable precursor for the synthesis of either dinuclear double-stranded tetracarbene complexes or doubly orthometalated heterobimetallic complexes. Compound 27, the precursor for an interesting tridentate ligand containing carbene and alcoholato donor functions, was prepared by the reaction of two equivalents of an N-alkylimidazole with a functionalized epichlorhydrin (Figure 1.4). Peris and co-workers prepared the Y-shaped tris(imidazolium) salt 28 and described the formation of homo- and heterobinuclear complexes derived from this NHC precursor, together with a successful application of these complexes in tandem catalysis. Tris(imidazolium) salt 29 was first described by Dias and Jin, shortly followed by the description of triazolium salts of type 30. Additional tripodal tris(imidazolium) salts of types 31 and 32 and their NHC complexes were also prepared. A number of phenylene-bridged polyazolium salts has been described. Both homo- and heteronuclear NHC complexes have been obtained from the phenylene-linked tris(imidazolium) salts 33 and 34. The 1,3,5-phenylene-linked tris(imidazolium) salt 34 as well as the phenylene-linked tetra- (35) and hexakis(imidazolium) salts 36 are also capable of forming molecular cylinders by connecting two polycarbene ligands via linear bound coinage metals. These cylinders have been prepared by treatment of the polyazolium salts with Ag2O followed by transmetallation to gold or copper. This reaction proceeds with retention of the metalosupramolecular structure, and no formation of heteronuclear complexes was observed. Applying this procedure, access to nanometer-sized molecular cylinders is also possible by metalation of the 1,3,5-triphenylbenzene-bridged tris(imidazolium) salt 37. A similar tris(imidazolium) salt has been used for the generation of supported and catalytically active palladium nanoparticles.
(Continues...)
Excerpted from N-Heterocyclic Carbenes by Silvia Díez-González. Copyright © 2017 The Royal Society of Chemistry. Excerpted by permission of The Royal Society of Chemistry.
All rights reserved. No part of this excerpt may be reproduced or reprinted without permission in writing from the publisher.
Excerpts are provided by Dial-A-Book Inc. solely for the personal use of visitors to this web site.