Molecularly Imprinted Polymers for Analytical Chemistry Applications / Edition 1 available in Hardcover, eBook
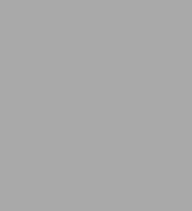
Molecularly Imprinted Polymers for Analytical Chemistry Applications / Edition 1
- ISBN-10:
- 1782626476
- ISBN-13:
- 9781782626473
- Pub. Date:
- 04/26/2018
- Publisher:
- RSC
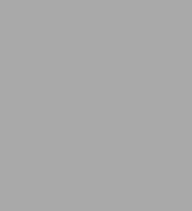
Molecularly Imprinted Polymers for Analytical Chemistry Applications / Edition 1
Buy New
$250.00Overview
The book summarizes the latest developments and applications of molecular imprinting for selective chemical sensing with each chapter devoted to different analytical applications of molecularly imprinted polymers. Specific chapters include: designing of molecular cavities aided by computational modelling, application of molecularly imprinted polymers for separation as well as sensing of pharmaceuticals and nucleotides.
The book is suitable for academics, postgraduates, and industrial researchers active in analytical chemistry, synthetic organic chemistry, molecular recognition, electrochemistry, and spectroscopy.
Read an Excerpt
CHAPTER 1
Nano-sized Molecularly Imprinted Polymers as Artificial Antibodies
F. CANFAROTTA, A. CECCHINI AND S. PILETSKY
1.1 Molecularly Imprinted Polymers: Different Formats for Different Applications
Molecularly imprinted polymers (MIPs) emerged about 40 years ago, when Wulff and Sahan proposed the strategy of polymerisation performed in the presence of a target-template. Since then, MIPs have been exploited for multiple applications thanks to their remarkable properties, such as high affinity and selectivity, and resistance to extremes of temperature, pressure and pH variations. In molecular imprinting, functional and cross-linking monomers are polymerised in an appropriate porogenic solvent in the presence of the compounds to be imprinted (called "templates"). After removal of the template, the polymer matrix retains recognition cavities that are complementary to this template in terms of size, shape and functionality. More precisely, the three-dimensional arrangement of cavity recognizing sites is driven by the structure of the template molecule. Therefore, after template removal the synthesized polymer is capable of binding the target, which can be the analyte itself. Compared with synthesis of monoclonal antibodies (mAbs), the synthesis of MIPs is simpler and cheaper, and can be performed without any preclinical development involving animals. In addition, MIPs show high stability and excellent mechanical properties. Moreover, they can be prepared for a wide variety of targets. Template removal, however, is often difficult and incomplete, with the possible disadvantage of subsequent analyte leaching from the matrix, thus resulting in inaccurate performance in analytical applications. Moreover, it is quite laborious to integrate them with signal transducers in sensors (unless electropolymerisation is involved, see Section 1.3.1.2, below) or to convert the template binding into an electric signal. In general, MIPs can be manufactured in different ways (Table 1.1) and in several formats, for example as films or membranes, microparticles or nanoparticles. Compared to other formats, the nanoparticles present several advantages. In particular, this format allows the system to exhibit a much higher surface-to-volume ratio and larger total surface area per weight unit of polymer. The imprinted cavities are more easily accessible by the analytes, thus improving binding kinetics and template removal and, hence, enhancing their recognition capabilities. Several authors have started developing nanoMIPs for diagnostic and therapeutic applications, for instance as drug delivery systems and sensing elements in assays or sensors. In virtue of their features, nanoMIPs represent an attractive option for a wide range of applications. One interesting characteristic of nanoMIPs is their property of remaining in solution, rendering them suitable for in vitro studies. However, it is crucial to obtain particle batches with a very narrow size distribution and with high yield, especially for biomedical studies. Two other great advantages of MIPs, compared to natural ligands, are their relatively straightforward preparation and, particularly, their inexpensive fabrication. Indeed, the availability of cheap reagents for MIP syntheses has led researchers to explore novel polymerisation approaches for devising smaller and monodispersed MIPs, from the most intuitive techniques (i.e. precipitation polymerisation) to more sophisticated ones (i.e. the use of solid-phase polymerisation and automated reactors).
1.2 Advances in the Synthesis of NanoMIPs; Different Approaches to Preparation of MIPs as Nanoparticles
Molecular imprinting involves three main steps; i.e. (i) the formation of the monomer–template complexes, (ii) polymerisation, and (iii) removal of the template and collection of the MIPs. In general, MIPs can be fabricated by means of two main approaches: covalent and non-covalent imprinting. In the former, developed by Wulff, reversible chemical bonds are created between the monomer and the template during the polymerisation, and the same bonds are then re-formed in the analyte binding step. The advantage is that only the monomer's functional groups interact with the template and cavities with more homogeneous recognizing sites are generated. However, not many compounds are suitable for this approach to be used and, therefore, they need preliminary derivatization with the monomer. Furthermore, the template removal is quite difficult and the analyte binding step is slower compared to that of other approaches. In the non-covalent approach pioneered by Mosbach and co-workers, hydrogen bonding as well as electrostatic and hydrophobic interactions are involved in the formation of the monomer–template pre-polymerisation complexes, as well as in the following analyte recognition. Since weak interactions are involved, an excess of monomer is usually employed to stabilise the monomer–template complex. This method is easier and more versatile than the covalent approach, although issues related to heterogeneity of the binding sites within cavities generated might arise. Considering the advantages of the aforementioned two approaches, some authors have combined them, thus using a template covalently linked to the monomer and the following analyte binding step designed in a non-covalent way, thus introducing the concept of semi-covalent imprinting. In this section, we will explore the main polymerisation modalities optimised so far for the synthesis of MIP nanoparticles (nanoMIPs), by focusing on (i) precipitation polymerisation, (ii) mini-/micropolymerisation and core–shell polymerisation (Scheme 1.1), (iii) atom transfer radical polymerisation (ATRP) and reversible addition–fragmentation chain transfer polymerisation (RAFT), as well as (iv) solid-phase polymerisation without and with the use of automated reactors, with particular emphasis on pros and cons of each approach (Scheme 1.2).
1.2.1 Precipitation Polymerisation
Among all the techniques used to synthesise nanoMIPs, certainly precipitation polymerisation is the fastest and most straightforward strategy for obtaining monodispersed nanoparticles with high yield. Typically, this approach is based on a free-radical polymerisation occurring in highly diluted mixtures of monomers. The "growing" pre-polymer keeps enriching it with monomers, until it precipitates when its size renders the polymer insoluble. In this manner, spherical and uniform nanoparticles are rapidly produced with high yield and low consumption of reagents. However, the main drawback of this method lies in its strength point. In fact, the diluted solution of monomers may decrease the interaction between the active monomers and the template molecule. This decrease leads to a less efficient formation of the monomer-template pre-polymerisation complex and, therefore, it decreases the selectivity of the resulting nanoMIP. Moreover, the increase of the concentration of monomers leads to larger and less uniform nanoMIPs. In the early 2010s, polymerisation in concentrated monomer solutions was performed. The idea was to stop the polymerisation before the gelation point by abruptly diluting the solution of pre-polymers; subsequently, a poor solvent for the polymer was added for nucleation of the nanoMIPs. Usually, precipitation polymerisation is performed using organic solvents. Nevertheless, it is also possible to precipitate nanoMIPs from aqueous solutions if a surfactant is added (at a low concentration) to the monomer mixture. Obviously, after synthesis it is necessary to remove not only the template but also the surfactant. As discussed above, several parameters play a crucial role in this polymerisation, thus affecting quality of the resulting nanoMIPs. For instance, the ratio of different reagents, the type and concentration of the reagents (particularly the template) and the solvent can affect the resulting nanoMIP and reaction yield. In conclusion, precipitation polymerisation is a cost-effective, time saving, and quick procedure for fabrication of nano-and microsized MIPs. This polymerisation allows the synthesis of uniform spherical nanoMIPs with high yield and the possibility to desirably tune their parameters.
1.2.2 Mini- and Micro-emulsion Polymerisation
As mentioned in Section 1.2.1 above, the polymerisation mixture can be optimised and enriched with different chemical species, e.g. surfactants. Notably, emulsion polymerisation involves organic solvent solutions of template-conjugated functional monomers and organic cross-linking monomers. The solution is then emulsified in an aqueous solution of surfactants and stabilisers (e.g. sodium dodecyl sulphate, SDS), and then the solution is usually stirred and sonicated. Generally, emulsion polymerisation can be classified as mini- and micro-emulsion polymerisation. The mini-emulsion approach exploits co-surfactant or surfactant monomers and, eventually, a stabiliser is added to produce nanoparticles with homogeneous size. The peculiarity of mini-emulsion polymerisation lies in the synthesis of semicovalently imprinted nanoparticles, i.e. the formation of the pre-polymerisation complex of monomers with the template engages covalent bonds while the target binding depends upon non-covalent interactions. NanoMIPs fabricated using this procedure show high affinity and selectivity for the target. On the other hand, however, micro-emulsion polymerisation generally occurs under more complicated conditions, namely, in the presence of water, oil, and one or more surfactants. Moreover, spherical nanoMIPs are obtained using different procedures. A clever approach involves the addition of monomers or peptides conjugated to fatty acid chains to increase the confinement of the template to the surface of the growing nanoMIPs. The main drawback of this technique is related to the presence of SDS and other chemicals. In fact, the presence of surfactant(s) and stabilisers in solution might affect monomer–target recognition and, therefore, it requires several washing steps, which may compromise the yield of the reaction as well as the efficacy and homogeneity of the nanoMIPs. Moreover, the application of these MIPs is limited to in vitro studies because it is impossible to completely remove those chemicals, a crucial step for any in vivo application. Therefore, nanoMIPs produced via emulsion polymerisation are not recommended for biological applications. Nevertheless, mini- and microemulsions allow easy and rapid synthesizing of uniform nanoMIPs exhibiting remarkable recognition properties. Moreover, emulsion polymerisation, together with grafting polymerisation, can be exploited for assembling core–shell nanoMIPs. The most common "core materials" are polymeric structures, silica, or magnetic nanoparticles (i.e. Fe3O4 nanoparticles). As discussed above, multiple advantages of emulsion polymerisation render this synthetic approach particularly suitable for devising core–shell nanoparticles. There are a few interesting strategies, which have been widely employed during the past years. In particular, the fabrication of core–shell nanoparticles is composed of two steps, i.e. (i) formation of the core and (ii) creation of the MIP shell by emulsion polymerisation. In all cases, from the use of latex cores to magnetic ones, the diameter of core–shell nanoMIPs was lower than 100 nm, thus opening up the gates to several different applications. Generally, semi-covalent imprinting is the favourite approach, but also non-covalent imprinting has been exploited and was successful in terms of affinity and selectivity of the composite nanoparticles. Despite the drawbacks listed above, the emulsion polymerisation still remains the most suitable approach for devising core–shell nanoMIPs, thanks to its straightforwardness and high yield.
1.2.3 Atom Transfer Radical Polymerisation (ATRP) and Reversible Addition-fragmentation Chain Transfer Polymerisation (RAFT)
Over the past decades, heat- and UV light-initiated polymerisations have been exploited for the synthesis of MIPs. Nevertheless, the desire to continuously optimise the polymerisation protocols has led to the development of new approaches, such as microwave-assisted synthesis initiation and controlled living radical polymerisation procedures (i.e. ATRP and RAFT). In particular, these novel polymerisation strategies have been largely employed for either the fabrication of MIP films or core–shell nanoMIPs. Indeed, the big advantage of living radical polymerisation, compared to conventional free-radical polymerisation, lies in the control of the thickness of the MIP film, which is a crucial requirement for devising composite nanoparticles. Moreover, the ATRP method exploits metal ion catalysts (usually copper ions) to coordinate the interactions operative in the formation of the monomer–template conjugate and the overall polymerisation reaction. The ATRP mechanism is based on the equilibrium between radical and inactive species. Ions of metals, such as copper, iron, and molybdenum, mediate the entire catalytic process. When these metal ions are in their low oxidation state, they react intermittently with the inactive species. Therefore, "dormant" species act as both activators promoting the formation of the growing polymer and as deactivators, stopping the activity of the radicals, hence re-stabilising the equilibrium between the species in solution. The equilibrium of the reaction and the metal ions employed are the strength point of this method. Moreover, they allow for control of the polymerisation itself. However, one drawback of ATRP is related to the presence of these metal ions. The metal ion catalyst might affect monomer–template recognition and it must be removed after the end of the polymerisation. Therefore, several and sophisticated purification steps are necessary (e.g. ion-exchange resins must be employed). With further optimisation of the polymerisation parameters, the amount of copper can be decreased, which is required for biomedical applications.
(Continues…)
Excerpted from "Molecularly Imprinted Polymers for Analytical Chemistry Applications"
by .
Copyright © 2018 The Royal Society of Chemistry.
Excerpted by permission of The Royal Society of Chemistry.
All rights reserved. No part of this excerpt may be reproduced or reprinted without permission in writing from the publisher.
Excerpts are provided by Dial-A-Book Inc. solely for the personal use of visitors to this web site.