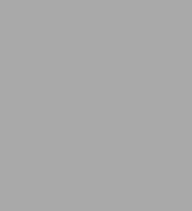
Molecular Aspects of Innate and Adaptive Immunity
297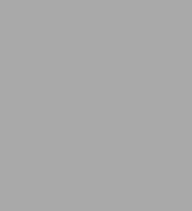
Molecular Aspects of Innate and Adaptive Immunity
297Hardcover
-
PICK UP IN STORECheck Availability at Nearby Stores
Available within 2 business hours
Related collections and offers
Overview
Product Details
ISBN-13: | 9780854046980 |
---|---|
Publisher: | RSC |
Publication date: | 09/29/2008 |
Pages: | 297 |
Product dimensions: | 6.30(w) x 9.30(h) x 0.90(d) |
About the Author
Read an Excerpt
Molecular Aspects of Innate and Adaptive Immunity
By Kenneth BM Reid, Robert B Sim
The Royal Society of Chemistry
Copyright © 2008 Royal Society of ChemistryAll rights reserved.
ISBN: 978-0-85404-698-0
CHAPTER 1
R. R. Porter and the Structure of Antibodies
LISA A. STEINER AND JULIAN B. FLEISCHMAN
1.1 Introduction
In 1967 Rodney R. Porter was invited to succeed Sir Hans Krebs as Whitley Professor of Biochemistry at Oxford. Porter founded and headed the MRC Immunochemistry Unit there, which now celebrates its 40th anniversary.
Porter's background and his classic work in the field of immunochemistry had begun well before his arrival at Oxford. As a student of Fred Sanger at Cambridge he was introduced to the structural basis of protein function, a topic that caught his fancy and pointed him toward immunology. Porter was intrigued by Landsteiner's work on the specificity of antibodies and the biochemical basis for the 'antibody paradox', namely how a diverse group of proteins, such as antibodies, could have remarkably different specificities yet have apparently similar structures. In 1958 Porter was a cofounder of the Antibody Workshop, a small international group of researchers that met regularly in a series of informal and often lively sessions over a period of seven years to discuss fundamental research problems in immunology. This chapter outlines Porter's fundamental contributions to our present knowledge of antibody structure and the biochemical basis of their specificity. In recognition of this work, Porter shared the Nobel Prize in Physiology or Medicine with Gerald Edelman in 1972.
The early demonstration of the passive transfer of immunity by serum had paved the way for establishing the molecular nature of antibodies. Methods still were needed to fractionate serum and purify its constituents, and it is, indeed, striking that the understanding of antibody structure closely followed advances in methods to purify and characterize proteins. In the 1930s a centre that developed such methodologies was the Svedberg laboratory in Uppsala, and it was there that the first steps were taken toward determining the component of serum that carried antibody activity. Ultracentrifugation indicated that antibodies sedimented either at 17–19 S or at 6–7 S, corresponding to molecular weights of about one million and 160000, respectively. With the technique of free boundary electrophoresis, Tiselius and Kabat demonstrated that the antibody activity in a rabbit antiserum to ovalbumin was confined to the γ-globulin region (fraction migrating slowest toward the anode). Sometimes, however, antibodies migrated faster on electrophoresis. Furthermore, some proteins without antibody activity (e.g., properdin, a protein of the alternative complement pathway) also migrated in the γ-globulin fraction. Subsequently it was realized that all antibodies, even those of different classes [e.g., immunoglobulin G (IgG), IgM, IgA, see below], share many basic structural features, despite differences in size or in electrophoretic properties. Eventually the term 'immunoglobulin' was introduced to include the set of all proteins that share the essential structural features of antibodies. The term immunoglobulin refers to the antibody as a protein, regardless of its antigen-binding activity.
1.2 The Papain Fragments
In the 1950s the pioneering work of Fred Sanger in developing methods to establish the amino acid sequence of insulin ushered in an era of rapid advances in the determination of the sequences of proteins. Rodney Porter, a PhD student of Sanger, was interested in understanding the chemical basis for antibody activity. At that time the only proteins for which amino acid sequences had been determined (insulin, ribonuclease, lysozyme) were at least an order of magnitude smaller than antibody molecules. An even more formidable problem was that different preparations of antibody, known to bind to the same antigenic determinant, varied measurably in molecular properties (e.g., as demonstrated by electrophoresis), and were impossible to fractionate into homogeneous constituents. Nevertheless, heterogeneity did not obscure the substantial similarity among all immunoglobulin molecules. The major distinction between antibodies was in their recognition of different antigens, not in their overall molecular structure. As Porter noted in his Nobel lecture, "This combination of an apparently infinite range of antibody combining specificity associated with what appeared to be a nearly homogeneous group of proteins astonished me and indeed still does". Thus antibodies contrasted strikingly with enzymes, which typically differ substantially from one another in structure as well as in specificity.
Porter's plan was to simplify the sequencing problem by breaking the antibody molecule into fragments, hoping that one or more of the smaller pieces would retain specificity for antigen. He was influenced by the work of Landsteiner who had shown that in many cases only a small part of an antigen was able to bind to its antibody, suggesting that the combining site of the antibody also may be smaller than the whole antibody molecule. The plan also assumed that the heterogeneity of an antibody preparation would not prevent the isolation of its constituent fragments.
Porter's approach was to digest the antibody with papain. His initial efforts and those of others had shown that treatment with proteolytic enzymes yielded fragments of lower molecular weight that still could bind to antigen, but these products had not been isolated or characterized in detail. These early experiments were limited by the lack of pure enzymes and effective methods to fractionate mixtures of proteins. However, by the late 1950s these materials and techniques had improved significantly. Thus the availability of highly purified papain meant that the specific digestion products would not be substantially contaminated by enzyme. In addition, the newly introduced carboxymethyl-cellulose ion-exchange resins efficiently separated the digestion products.
In a renewed effort, Porter treated several rabbit antibodies, each specific for a different antigen, with crystalline papain; the resulting digests were fractionated on columns of carboxymethylcellulose. In each case, three fractions of approximately equal size were obtained. Porter named them fractions I, II and III (Figure 1.1), in order of their elution; these fractions together accounted for almost all of the original antibody molecule and were resistant to further digestion with papain. None of the fractions precipitated with the corresponding antigen, but fractions I and II, which were very similar in size and amino acid composition, specifically inhibited the precipitation of the antigen by the homologous antiserum. This suggested that the original antibody was 'multivalent' and could cross-link molecules of antigen, but that fractions I and II were 'univalent', i.e. they still could specifically bind to antigen molecules but could not cross-link them. Fraction III, which could not specifically inhibit precipitation, was shown to contain structures that enabled the transmission of the antibody across the placenta, the binding of antibody to guinea pig skin permitting anaphylactic reactions upon antigenic challenge, and the activation of complement.
Fraction III crystallized readily when dialyzed against buffers of neutral pH, an unexpected result since the starting antibody preparation did not crystallize. This suggested that this fragment of the antibody might be more structurally homogeneous than the original material. It was further inferred from these results that the IgG molecule may consist of three tightly folded globular segments that are resistant to further digestion by papain, whereas the polypeptide chain(s) connecting these segments are more exposed to proteolytic digestion.
In later years Porter enjoyed recalling that he first believed that the crystals, which appeared upon dialysis of the papain digest in the cold, consisted of cystine, the oxidation product of the cysteine that had been used to activate papain. Accordingly, for several months he discarded the crystals in the sink. As he noted in his Nobel lecture, it was fortunate that his neighbour at the National Institute for Medical Research at that time was Olga Kennard, a crystallographer. When Porter finally asked her opinion about the crystals, she remarked that they looked like crystals of protein, not cystine. They then were identified as the fraction III obtained by separation of the papain digestion products of the antibody on carboxymethyl-cellulose.
Fractions I and II are similar. Their separation on carboxymethylcellulose was fortuitous and merely reflected chemical heterogeneity, a result of their overall charge and the column elution conditions. Fragments I and II later were renamed as fragment antigen-binding (Fab) and the crystallizable piece III was renamed as fragment crystallizable (Fc); see Ceppellini et al. for a summary of immunoglobulin nomenclature. The striking differences between the Fab and Fc fragments were an early clue to the solution of the antibody paradox. The two Fab fragments on a single antibody molecule are identical to one another and contain the combining sites for antigen, but they differ from one antibody molecule to another. The Fc fragments are shared by all IgG molecules.
Another interesting proteolytic fragment of antibodies called Facb (for fragment antigen and complement binding) subsequently was discovered at the MRC Immunochemistry Unit at Oxford. It consists of an IgG molecule from which only part of the Fc had been removed. The Facb fragment is bivalent and therefore can still precipitate and agglutinate antigens and it also retains complement-fixing activity.
1.3 The Four-chain Model
Another approach to analyzing the structure of antibodies was taken by Gerald Edelman while he was still a graduate student at the Rockefeller Institute. Edelman found that when IgG was reduced with a mercaptan in the presence of a dissociating solvent such as 6 M urea, its molecular weight dropped significantly, demonstrating that it consists of a number of polypeptide chains, cross-linked by disulfide bridges. But these results disagreed with Porter's earlier end-group analyses that had shown approximately one free amino-terminal residue per antibody molecule, consistent with a single-chain protein. To resolve the discrepancy and to obtain products that might retain some biological activity, Porter and colleagues modified the conditions of reduction and chain separation. A key step was the use of mild reduction conditions, which had been shown by Cecil and Wake to preferentially cleave interchain disulfide bonds. The chains prepared in this way remained soluble after separation by gel filtration in 1 M propionic acid.
By good fortune, Julian Fleischman, a postdoctoral fellow who joined the Porter laboratory at that time, brought antisera to the Fab and Fc fragments which he had helped prepare in Melvin Cohn's laboratory at Stanford. A simple double immunodiffusion experiment established the relation between the polypeptide chains and the Fab and Fc fragments. Porter first proposed the four-chain model for IgG at a meeting in New York. Some initial uncertainty about the molecular weights of the separated chains meant the possibility that the molecule consisted of only two or even three chains was also considered. Re-determination of the molecular weights confirmed that there were two heavy and two light chains per molecule. The now-familiar four-chain model was presented by Fleischman et al.
As pointed out by Fleischman, a critical finding for the final model was the observation of Alfred Nisonoff and colleagues that pepsin digestion of the IgG molecule at pH ~4.5 degrades the Fc fragment but leaves the two Fab-like fragments intact and linked to one another by a disulfide bond; the resulting bivalent fragment is called F(ab')2. Thus previous models, in which the combining sites were placed at the distal ends of a cigar-shaped molecule, were no longer tenable.
The four-chain IgG model, with minor modification mainly in the number and location of interchain disulfide bridges, has stood the test of time. It also formed the basic structure for the other immunoglobulin classes. In IgG there are two identical heavy chains and two identical light chains of molecular weight 50000 and 23 000, respectively. Each Fab fragment consists of one entire light chain plus the amino-terminal half of one heavy chain; the Fc fragment consists of the remainder of both heavy chains. The presence of two Fab fragments, each of which binds antigen, was consistent with the bivalence of IgG antibodies. The model was reconciled with Porter's end-group analyses when it was realized that the heavy chains have a blocked amino-terminus and that the light chains are heterogeneous at the amino-terminus; thus the yield of a single end-group per molecule did not reflect the actual number of chains.
1.4 V and C Regions of Immunoglobulin Chains
Once the four-chain structure of IgG had been established, Betty Press (E. M. Press) and others in Porter's laboratory turned to amino acid sequence determination in immunoglobulins to help elucidate the structural basis of antibody specificity. The most suitable materials at hand were the myeloma immunoglobulins because of their abundance and homogeneity. Although the combining specificities of these immunoglobulins were unknown, their primary structures might suggest the ways in which conventional antibodies would combine with antigens. For their analysis they chose the Fd fragment, the segment of heavy chain in Fab. This sequence work had begun at St Mary's and much of it continued in Porter's laboratory at the MRC Unit in Oxford.
Amino acid sequence studies of immunoglobulin light chains from two different myeloma immunoglobulins had revealed that they consisted of a variable (V) segment and a constant (C) segment, each comprising about half of the chain. The amino acid sequences of the Fd fragments of two human IgG myeloma immunoglobulins showed that there were also V segments in heavy chains similar in length to those of the light chains. This dramatically confirmed that antibodies contained sequences specific for particular antigens (the V regions) and sequences common to all immunoglobulins of a particular class (the C regions). The structural basis underlying the antibody paradox, first suggested in Porter's identification of the Fab and Fc fragments of the antibody molecule, had been revealed, and it resided in the primary structure, the amino acid sequences, of the antibody polypeptide chains themselves.
The presence of V and C regions in immunoglobulins was established largely by analysis of myeloma proteins. However, Porter also investigated actual antibodies with known specificity for antigen. Although rabbit antibodies were heterogeneous and therefore problematic for amino acid sequence determination, partial amino acid sequences of rabbit immunoglobulin heavy chains were obtained in Porter's laboratory and they led to similar conclusions. Porter also was interested in the genetic basis for antibody diversity, and rabbit anti-bodies were potentially useful for such studies because they expressed inherited variants of antibody molecules called allotypes. In 1969 Fleischman had purified a single, relatively homogeneous rabbit antibody to streptococcal carbohydrate and in Porter's laboratory at the MRC Immunochemistry Unit at Oxford he determined partial amino acid sequences in the V region of its heavy chain. The results, together with previous work, suggested that allotype-related amino acid sequences were probably present in the V region, and thus V region diversity was likely to be related to structural genes carried in the germ line.
Following his sequence studies on immunoglobulins, Porter turned his attention to the structures and genetics of the complex complement proteins described in other chapters in this volume. Porter's landmark career in immunochemistry ended abruptly with his untimely death in a road accident in 1985, only a few weeks after his retirement from the Whitley Chair of Biochemistry. A special symposium had just been held at Oxford in his honour to commemorate that occasion, and the proceedings published as Biochemical Society Volume 51 – Genes & Proteins in Immunity. Porter had planned to continue his research as director of the MRC Immunochemistry Unit. His loss was deeply felt by his family and by all of his students and colleagues. His achievements in science stand as a tribute to his life and his work.
(Continues...)
Excerpted from Molecular Aspects of Innate and Adaptive Immunity by Kenneth BM Reid, Robert B Sim. Copyright © 2008 Royal Society of Chemistry. Excerpted by permission of The Royal Society of Chemistry.
All rights reserved. No part of this excerpt may be reproduced or reprinted without permission in writing from the publisher.
Excerpts are provided by Dial-A-Book Inc. solely for the personal use of visitors to this web site.