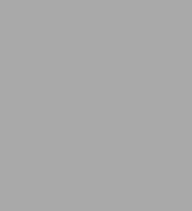
Military, Naval and Civil Airships Since 1783: The History and Development of the Dirigible Airship in Peace and War
304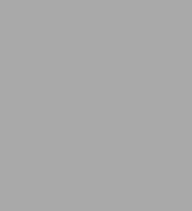
Military, Naval and Civil Airships Since 1783: The History and Development of the Dirigible Airship in Peace and War
304eBook
Available on Compatible NOOK devices, the free NOOK App and in My Digital Library.
Related collections and offers
Overview
An exploration of the history and development of the dirigible airship from its humble beginnings in the late eighteenth century through to its current role as military command posts among other uses.
Starting out as an unreliable experimental aircraft whilst aeronauts first began to learn the secrets of aerial navigation, the airship was remodelled in 1900 by Count Zeppelin to become a potent weapon of war. It was then transformed again into a short-lived solution to long-distance passenger air travel. With over 100 technical drawings and contemporary images of dirigible aircraft, Ridley-Kitts presents a comprehensive and fascinating history of the airship.
Military, Naval and Civil Airships is a must read for those that wish to delve into the development of the aircraft for the first time and for airship specialists alike.
Product Details
ISBN-13: | 9780752490373 |
---|---|
Publisher: | The History Press |
Publication date: | 03/01/2012 |
Sold by: | Barnes & Noble |
Format: | eBook |
Pages: | 304 |
File size: | 31 MB |
Note: | This product may take a few minutes to download. |
Age Range: | 18 Years |
About the Author
Read an Excerpt
Military, Naval and Civil Airships Since 1783
The History and Development of the Dirigible Airship in Peace and War
By Daniel George Ridley-Kitts
The History Press
Copyright © 2012 Daniel George Ridley-KittsAll rights reserved.
ISBN: 978-0-7524-9037-3
CHAPTER 1
The Airship: An Explanation
The principle by which an airship maintains itself in the air is, of course, displacement: whereby a measured volume of air is replaced by an equal volume of a lighter gas, such as helium or hydrogen, which imparts a static lift to such a craft.
In the case of a hydrogen-filled airship, 1,000cu ft of air at standard pressure and temperature weighs 80lb, while an equal volume of hydrogen will weigh 65.5lb, the difference in weight between these two gases giving a resultant lifting force of 14.5lb per 1,000cu ft of hydrogen. In reality, the complex equation required to calculate the lift of an airship includes such variables as ambient air temperature, gas pressure, humidity, barometric pressure and gas purity (that is to say, to what degree the hydrogen has been contaminated with common air due to osmosis through the cell walls). These calculations assume a standard pressure and temperature at sea level with the cells 95 per cent full and the craft fully laden with crew, petrol, water ballast and its useful load of cargo or weapons.
During the First World War, hydrogen was the sole lifting agent – an explosive element first identified by the English physicist Cavendish in 1742. Hydrogen and later coal gas (a cheaper alternative that, although affording less lift, was more readily available in Victorian times from town gas supplies) were used by the early balloonists for their aerial voyages. Whilst they provided the necessary lifting force, they were both extremely dangerous to use in practice, a fault which, in the end, militated against their employment for both civilian and military use.
The use of helium – an inert and, therefore, safe gas – was introduced in 1922 by the Americans for use in their airships, following a series of fatal fires involving hydrogen-filled airships in the United States and Europe. The Americans had a monopoly of this rare gas; first detected in the spectrum of the sun before being discovered on earth in the 1900s in Texan oil wells, from where it was produced by a complex and expensive refining process.
Helium was not only some ten times more expensive to produce than hydrogen in those early days, but also possessed only 85 per cent of the lifting capacity compared with an equal volume of hydrogen. Consequently, a helium-filled airship would have to be proportionally larger than a hydrogen-filled craft in order to lift the same load.
The efficiency of an airship is measured by a comparison between the 'gross lift', or the total lifting power, and the 'useful or disposable lift'. This represents the difference between the fixed weights of the structure, framework, gas cells, outer cover, engines and equipment, and the useful payload in terms of crew, passengers, cargo, fuel and water ballast. In most early airships the useful load was in the order of 25 per cent of the total lift, although in the 'height climber' class produced by the Zeppelin Company towards the end of the Great War, efficiencies in excess of 60 per cent were achieved.
These savings were only accomplished at the cost of lightening the structure to such an extent that structural failure was an ever-present danger, which could and did occur when these lightly built ships were subjected to high-speed manoeuvres in the denser air at low altitude.
Airships fall into three categories that relate to their structure and the method of construction employed. The first type is the pressure airship, or blimp. This is, in effect, a motorised balloon – from which they were first developed effectively in the mid-nineteenth century – initially using steam, then electricity and finally the internal combustion engine as prime mover.
In its simplest form the pressure airship consists of an elongated balloon of streamline form, in order to present a surface of least resistance to the airflow, and containing the lifting agent which would have been hydrogen before and during the First World War period. Below the envelope would be suspended a car containing the crew, controls, engines, ballast and bombs etc., with the fuel tanks either suspended above the car or situated within the envelope. Steerable control surfaces were fitted to the rear portion of the envelope in order to achieve directional control in flight.
1. Francesco de Lana's aerial ship proposal, 1680.
In a pressure airship, the shape of the gas-tight envelope is maintained by the internal gas pressure being at a higher value than that of the supporting air, so maintaining the integrity of the structure under varying conditions of air pressure relative to altitude. Also, the temperature differential caused by meteorological conditions will affect the density and volume of the lifting agent, and therefore its lifting capacity.
As previously stated, the displacement of the heavier air by the lighter hydrogen or helium provides the static lift that enables the airship to float in the air. Around 1,000cu ft of hydrogen will lift 14.5lb at standard pressure and temperature. However, barometric and atmospheric conditions can adversely affect the lift equation with great rapidity. For example, the shadow of a cloud falling on the nose of an airship can cause an instant cooling effect on the gas, contracting the volume and lessening its lift, causing the nose to pitch down. Conversely, with a ship emerging from a cloud into warm sunshine, an instant heating effect will expand the gas: increasing the lifting capacity and causing the nose to rise.
A colder gas temperature regime within the envelope, in relation to the ambient air temperature, affords a greater percentage of lift than a warmer, more rarefied gas regime. On the other hand, if the gas contained in the cells is warmer than the outside air, this will result in less lift being created from an equal volume of gas. Paradoxically, more lift can be temporarily generated in a heavy airship by driving the craft down rapidly from a higher altitude: so compressing the gas molecules and producing heat, which leads to a temporary increase in lift. This method was often employed by airships when returning to base following a long patrol, having expended much of their lifting gas and ballast, in an 'exhausted' condition, and in danger of making a heavy landing.
In pressure airships it was (and still is) necessary to inflate the envelope to a higher pressure than that of the surrounding air in order to maintain the required degree of rigidity against the airflow – this pressure being in the order of 5lb/sq ft. This positive pressure is maintained by the introduction within the envelope of air-filled ballonets, usually two or more in number, occupying around a fifth to a quarter of the total volume of the envelope.
The ballonets are maintained at a positive pressure by an aluminium tube positioned in the wake of the propeller slipstream, allowing air to enter the ballonet via a one-way valve known as a crabpot. Any excess air is valved off through automatic air valves as the hydrogen expands in response to changes in pressure when the ship rises into less dense air. The ballonets are also employed to adjust the longitudinal trim of the craft, by partially filling or emptying the fore and aft compartments about the airship's centre of gravity (CG) to tilt the ship up or down, relative to the line of flight.
In order to prevent the fabric of the bow being pressed in under the pressure of the airflow, it was common practice to reinforce the bow-section cone with stiffening rods, usually made from bamboo radiating from a central aluminium nose cone and secured to the envelope by fabric pockets.
In pressure airships the fixed weights – that is to say the distribution of engines, control car, fins etc. – are supported from the envelope by a system of rigging wires, carried either internally or externally, and designed to spread the load evenly while maintaining the aerodynamic shape of the envelope and the trim of the ship. During the First World War, the British predominated in the development of this type of craft, building and operating over 200 very efficient and handy airships that were credited with some notable performances.
At the present time, the pressure airship remains almost the sole surviving type of dirigible airship that is still operating.
The second type of airship for consideration is the semi-rigid, which, as its name implies, is possessed of some elements of a rigid structure in the form of a metal keel. This keel is either attached to, or suspended from, the envelope, which, as well as acting as a walkway within the ship, also assists in absorbing the stresses acting on the structure imposed by aerodynamic loading in flight.
In some examples of this type of ship, a substantial tubular metal nose cone is fitted at the bow, while a cruciform girder work is incorporated in the rear keel structure to carry the external control surfaces. The gas was contained within the fabric envelope and this typically included a system of ballonets, as found in the pressure airships, to maintain the external shape. Semi-rigids were generally of a larger size than pressure airships, and the gas space was usually divided transversely into a series of separate compartments by fabric curtains to prevent gas from surging to either end of the envelope and upsetting the trim of the craft.
The first successful semi-rigids were those built in France in the 1900s, most importantly the creations of two brothers, Paul and Pierre Lebaudy, sugar refiners who, from their factory at Moisson, Seine en Oise, supplied a series of ships to the French army.
During and after the war period, it was the Italians who were to develop this particular type of craft, becoming the undisputed experts in this type of construction, which culminated in the remarkable flight in 1926 of the polar dirigible Norge. This noteable airship was captained by its designer, Umberto Nobile, and carrying the famed Norwegian explorer Roald Amundsen. The ship flew from Spitsbergen to Teller in Alaska, directly over the North Pole, in a continuous flight of seventy hours forty minutes, covering 3,180 nautical miles – a distance equivalent to the R34's transatlantic flight by an airship of one-third its capacity.
The third category of airship is the rigid, characterised by a metal or wooden framework forming a system of braced ring girder bulkheads, or transverse frames, interspersed with lighter intermediate transverse frames enclosing the separate gas cells, and connected by longitudinal latticed girders. The purpose of the girders is to absorb the powerful bending moments imposed on this long and relatively fragile craft. The completed framework is then covered by a stout, tightly doped fabric outer cover, in order to protect the fragile gas cells from the effects of weather and abrupt temperature variation.
In all rigids, the loads are distributed as evenly as is practical throughout the framework, so as to maintain the longitudinal trim and equalise the stresses acting on the structure. The heavier weights, such as engine cars and control gondola, are suspended from the main transverse frames, whilst petrol tanks, water ballast bags and other movable weights are arranged along the internal keel. These, in turn, are either attached directly to the frame structure or suspended from the radial wiring of the transverse frame bulkheads.
The lifting force developed within the gas cells is transmitted through the wire mesh netting enclosing the cells, through a system of lift wires attached to catenary wiring (similar in principle to the support cable in a suspension bridge) and connected to the transverse frames and longitudinal girders to spread the lift evenly throughout the entire structure. Another set of diagonal shear wiring holds the cells in place within the framework to minimise chafing against the girder work, while at each mainframe radial diagonal shear wiring forms a bulkhead to prevent cell surging.
Running from bow to stern, passing through the centre of each gas cell and anchored to the radial shear wiring bulkheads at each frame, runs the axial cable – designed to absorb and spread the static and aerodynamic loading exerted on the framework. Should a gas cell become deflated, the axial cable will compensate, to a degree, for the hogging effect on the adjacent structural members.
The control car was typically attached directly, or suspended below the framework at a position near the bow, containing at the front the steering position from where the commander could pilot the ship; directing the steering and height coxswains and controlling the engine-room telegraphs, ballast and gas valve controls etc. Behind this area was the navigation room, wireless room and a companionway giving access into the body of the ship.
The navigation of an airship was akin to that of a seagoing ship, with the use of dead reckoning and astral navigation, supplemented by an early form of wireless fixes from shore stations and ships, being the main aids to these early voyagers of the air.
Before the days of controlled air routes and modern navigational aids, the commander of an airship was required to make minute-to-minute decisions regarding course, speed and altitude, forever keeping an eye on the developing weather situation in order to guide his craft safely through the sky. The steering coxswain would be facing forward with his eye fixed on his compass course, while the elevator coxswain, the most experienced helmsman, would be standing sideways to the direction of flight watching the inclinometer and was responsible for the trim, attitude and altitude of the airship.
The engine cars were mounted along the sides of the ship in small streamlined nacelles, each with its own engineer who responded to the instructions received from the control car, via the engine room telegraphs, to control the speed of the engines.
At the stern of the ship, horizontal and vertical stabilisers were fitted. Originally these were of a flat externally braced non-aerodynamic section. These were later superseded by cantilever aerofoil-shaped fins, which were attached to the rear of the ship with strong cruciform girders traversing the face of the main ring structure – to provide a strong attachment point for the rudder and elevator controls.
This part of the structure needed to be of great strength due to the powerful aerodynamic forces and bending moments acting on the rudders and elevators in flight; many airships over the years experienced structural failure of the fins in flight due to inadequate stressing of the structure of these surfaces.
In rigid airships, ballonets were not employed as the gas pressure of the cells was equivalent to the external air pressure relative to the height. In early rigids, the cells were open at the bottom to allow for expansion of the excess gas, this being allowed to disperse through the outer cover. Later, automatic gas valves were fitted to the bottom of the cells and connected to fabric exhaust trunking. This ran between the gas cells to discharge through hoods on the upper surface of the hull; a safer option than discharging gas in the vicinity of hot engine exhausts.
Another means of discharging gas was by a series of 'manoeuvring valves', whereby measured quantities of gas could be released from the control car during flight or when landing.
Airships required large handling crews to bring them in and out of their massive sheds, sometimes in the order 200 men would be required to bring out or hold down a large rigid in a brisk breeze. While the Germans relied on large crews for ground handling, the British in particular utilised the mooring mast, pioneering this method towards the end of the Great War. Most British rigids were fitted with mooring cones which helped to alleviate the problems of handling large rigids on the ground.
The Americans later favoured the use of the 'stub mast', where the ship was moored on the ground with a car attached to the aft gondola, so that the airship could vane in the wind on a circular track. This arrangement was superior from the point of view of accessibility and servicing, and is the method still in use.
The process of the preparation of an airship for a voyage during the First World War period was a complex and labour-intensive operation, and a process always constrained by the vagaries of the weather. An airship was able to operate in only the most favourable conditions, sometimes unable to either enter or leave its shed due to strong crosswinds or beset by adverse meteorological conditions en route that could hinder its progress or, if severe enough, endanger the craft.
2. Montgolfier hot air balloon, Paris, 1783.
In the case of a rigid, at the commencement of a voyage – once all the cells had been filled with hydrogen, fuel tanks filled and water ballast, oil, war load, provisions and crew had boarded – it was customary for the ship to be 'weighed-off' in the shed. That is, to put the ship into a state of neutral buoyancy with the cells 95 per cent full, so that in this state the ship was just floating and could be lifted easily by the ground crew.
(Continues...)
Excerpted from Military, Naval and Civil Airships Since 1783 by Daniel George Ridley-Kitts. Copyright © 2012 Daniel George Ridley-Kitts. Excerpted by permission of The History Press.
All rights reserved. No part of this excerpt may be reproduced or reprinted without permission in writing from the publisher.
Excerpts are provided by Dial-A-Book Inc. solely for the personal use of visitors to this web site.
Table of Contents
Contents
Title,Dedication,
Acknowledgements,
Introduction – The Conquest of the Air,
1 The Airship: An Explanation,
2 The Development of the Dirigible 1783–1914,
3 Early British Efforts 1878–1914,
4 The First British Rigid: Mayfly,
5 Interim 1912–14: The Astra-Torres, Parseval & Forlanini Airships,
6 The Second Navy Rigid: R9,
7 War at Sea: Sea Scouts vs the U-boat,
8 War at Sea: Airships with the Grand Fleet,
9 Air Defence of Great Britain: Opening Moves,
10 Air Defence of Great Britain: Nemesis,
11 Zeppeline Gegen England Und Weiter 1915–18,
12 The R23 & 23X Classes,
13 Short Brothers' Wooden-Framed Y Type: R31 & R32,
14 R34 Across the Atlantic,
15 The Last of the Aerial Cruisers: R36 & Shenandoah,
16 The Vickers R80, Airship Sheds & Mooring Masts,
17 The Admiralty A Type: R38/ZR2,
18 The Airship vs the Flying Boat,
19 Airship Development in the Interwar Years,
20 From the Second World War to the Present Day,
Appendix 1 The R34's Transatlantic Flight,
Appendix 2 Airship Development in Great Britain 1900–30,
Appendix 3 The LZ-127 Graf Zeppelin,
Appendix 4 The First Zeppelin Raid on the British Isles,
Bibliography,
Copyright,