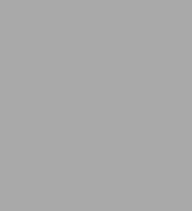
Metal-Organic and Organic Molecular Magnets
332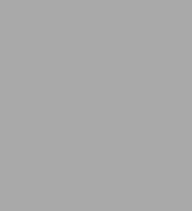
Metal-Organic and Organic Molecular Magnets
332Hardcover
-
SHIP THIS ITEMNot Eligible for Free ShippingPICK UP IN STORECheck Availability at Nearby Stores
Available within 2 business hours
Related collections and offers
Overview
Read an Excerpt
Metal-Organic and Organic Molecular Magnets
By P. Day, A. E. Underhill
The Royal Society of Chemistry
Copyright © 1999 The Royal Society of ChemistryAll rights reserved.
ISBN: 978-0-85404-764-2
CHAPTER 1
Molecular-based magnets: setting the scene
By Peter Day and Allan E. Underhill
Davy Faraday Research Laboratory, The Royal Institution of Great Britain, 21 Albemarle Street, London W1X fBS, UK School of Chemistry, University College of North Wales, Bangor, Gwynedd LL57 2DG, UK
In 1839, when Michael Faraday published the first picture of the lines of magnetic flux around a magnet (Faraday 1832), shown in figure 1, the cylinder of material in the centre of the figure could only have been one material, iron. Over the succeeding 160 years, the number of substances showing spontaneous magnetization has increased enormously, while their variety has broadened dramatically. Yet till quite recently, the field of magnetic materials has been traditionally confined to metals, among which the current 'market leaders' such as lanthanide–cobalt and Nd–Fe–B have achieved large technological significance. Among non-metallic phases, transition metal oxides made an early appearance in the years immediately before and after World War II, and the technologically driven need to understand and optimize their properties led to the phenomenological theories of Néel, complemented later by the microscopic models of Mott (1949), Anderson (1963) and Goodenough (1955). The latter, in particular, set out the orbital symmetry rules that brought the subject of cooperative magnetism firmly within the ambit of the solid-state chemist.
Halides, chalcogenides and pnicnides are for the most part continuous lattice compounds, and, apart from isolated instances that could be regarded more or less as curiosities (such as diethyldithiocarbamato–Fe(III) chloride (Wickman et al. 1967a, b) and Mn phthalocyanine (Barraclough et al. 1970)), magnetic solids built up from molecular coordination or organometallic complexes only arrived on the scene quite recently. Early work by the Dutch school (de Jongh & Miedema 1974) had shown that layer perovskite halide salts of Cu(II) were useful models for ferromagnetism in insulating two-dimensional lattices, while we found that by replacing Cu (S = 1/2) with Cr (S = 3/2) organic intercalated insulating ferromagnets could be synthesized with Curie temperatures up to 50 K (Bellitto & Day 1976; for reviews see Day (1986) and Bellitto & Day (1992)). These Cu(II) and Cr(II) salts were also excellent realizations of the ferromagnetic exchange arising from orthogonality between orbitals on neighbouring metal centres containing the unpaired electrons, of the kind pointed out by Goodenough (1963) and Kanamori (1959) for continuous lattice oxides. Organometallic charge transfer salts and one-dimensional ferrimagnetic coordination polymers followed in the 1980s (Miller et al. 1988; Nakatami et al. 1991), while the early 1990s saw the first ferromagnets made from purely organic molecular building blocks, without any metal atoms at all, heralding a new field of p-electron magnetism to complement that of d- and f-electron materials (Tamura et al. 1991).
Given the truly enormous number of magnetic solids prepared, characterized and exploited in the last century or so, it is legitimate to ask what new features, either experimental or theoretical, the molecular-based materials have brought to this field. There are many. From the point of view of synthesis and processing, the contrast with conventional magnetic materials could not be more stark: substances made at (or close to) ambient temperature, usually from solution compared with high temperature metallurgical or ceramic processes. A real chance exists to make a soluble magnet! Correlating magnets with other properties, it should be pointed out that all known molecular-based magnetic compounds are insulators, the precise inverse of the situation for continuous lattice materials. That simple fact has consequences for many of the accompanying properties, of which the most striking (and potentially one of the most useful) is optical. The molecular-based materials are frequently transparent to infrared and visible light. More than 20 years have passed since we first demonstrated the striking colour change occurring in an insulating ferromagnetic transition metal salt on passing through the Curie temperature as a result of coupling excitons to spin waves (Bellitto & Day 1978; Bellitto et al. 1980). Combining magnetism with properties only found in the molecular solid state, such as mesomorphism or chirality, would appear to be another potentially fruitful source of novel physics, as is the construction of unusual lattice topologies, such as the Kagome lattice, with which to test statistical thermodynamic models of critical behaviour. One apparent drawback of molecular-based magnetic materials, especially in the realm of information storage, is that the large majority are very soft magnets, i.e. they have quite low coercivities. However, even this limitation is now being breached, as two contributions to this issue demonstrate (Kahn, this issue; Kurmoo, this issue).
In all, the design, synthesis and study of molecular-based metal-organic and organic magnets has brought supramolecular and coordination chemistry, as well as purely organic synthetic chemistry to bear on a field previously dominated by condensed matter physics. In doing so, it has given new impetus to these fields and provided physics with new objects for study. While technological spin-off is in its infancy, it can confidently be predicted that new applications not replacing, but complementing, existing materials are not far away. It is to be hoped that the present collection of contributions, stemming from the lively Discussion Meeting organized under the auspices of the Royal Society and held on 24-25 March 1999, will be of wide interest to chemists and physicists, and will serve to broaden appreciation of this fascinating multidisciplinary topic.
CHAPTER 2p-Nitrophenyl nitronyl nitroxide: the first organic ferromagnet
By Minoru Kinoshita
Faculty of Science and Engineering, Science University of Tokyo, Daigaku-dori 1-1-1, Onoda-shi, Yamaguchi 756-0884, Japan
The transition to ferromagnetic order was realized in 1991 with the discovery of a p-nitrophenyl nitronyl nitroxide (C13H16N3O4) crystal. This was the first example of a ferromagnet without metal elements. Its ferromagnetism below the transition temperature of 0.6 K has been established by various experiments such as susceptibility, magnetization, heat capacity, zero-field muon spin rotation, neutron diffraction and ferromagnetic resonance measurements. Details of the results of these experiments are described in this paper.
Keywords: p-nitrophenyl nitronyl nitroxide; p-NPNN; muon spin rotation; pressure effect on ferromagnetism; ferromagnetic to antiferromagnetic transition; charge-transfer mechanism
1. Introduction
Solid-state properties of organic compounds have been extensively studied for several decades and it has been revealed that organic solids possess the potential ability to exhibit various interesting properties. The development of organic conductors and superconductors is one such example. In contrast to these advances, the absence of an organic ferromagnet was one of the most conspicuous problems about 10 years ago. The first explicit theoretical approach for an organic ferromagnet was proposed as early as 1963 by McConnell (1963). However, no purely organic crystal was found to be a three-dimensional 'bulk' ferromagnet, even after extensive studies on the magnetic properties of organic solids.
About 15 years ago, only a few organic radical crystals were known to exhibit an intermolecular ferromagnetic interaction. One of them was the galvinoxyl radical (Mukai et al. 1967; Mukai 1969). We then initiated extensive studies on this compound to search for conditions favouring the ferromagnetic interaction in organic solids. After experimental and theoretical studies over a couple of years, we derived the following conclusion (Awaga et al. 1986a–c, 1987a, b, 1988; Hosokoshi et al. 1997; Kinoshita 1991, 1993a). The requirement for the ferromagnetic intermolecular interaction is twofold, namely:
(a) large spin polarization within a radical molecule; and
(b) small SOMO-SOMO overlap and large SOMO-FOMO overlap between neighbouring radicals.
Here SOMO stands for the singly occupied molecular orbital, and FOMO the fully occupied (or unoccupied) molecular orbitals. Condition (a) states the requirement a radical molecule has to fulfil. The concept of spin polarization was well studied in the 1960s, particularly in odd -alternate organic compounds such as galvinoxyl and nitronyl nitroxide radicals. The spin polarization originates from an exchange interaction in a radical molecule. On the other hand, condition (b) is related to intermolecular interactions and to the relative location of the neighbouring radicals in a crystal. According to these conditions, the ferromagnetic intermolecular interaction originates in the exchange interaction within a molecule, which is always ferromagnetic. If the latter interaction is strong enough, it spreads out over a crystal through intermolecular charge-transfer interaction between SOMOs and FOMOs. The first radical employed fulfilling these conditions was p-nitrophenyl nitronyl nitroxide and it became the first example of an organic ferromagnet (Tamura et al. 1991; Nakazawa et al. 1992; Kinoshita 19936, 1995, 1996).
2. Molecular and electronic structure of p-nitrophenyl nitronyl nitroxide
Figure 1a shows the molecular structure of p-nitrophenyl nitronyl nitroxide (abbreviated as p-NPNN hereafter). The dot near the NO group denotes the unpaired electron that is responsible for the magnetism. The unpaired electron is mobile over the whole molecule, but mostly localizes on the ONCNO moiety and resides to a very small extent on the other parts of the molecule. The highly localized nature of the unpaired electron on the ONCNO moiety assures the molecule of large spin polarization as a result of strong exchange interactions between the unpaired electron and the lone-pair electrons on the hetero atoms. This qualitative prediction is also supported by an unrestricted Hartree-Fock (UHF) calculation of the molecular orbitals. As shown in figure 16, the energy of the molecular orbital containing the unpaired electron is much more stabilized than that of the fourth FOMO containing an electron of the opposite spin direction.
3. Crystal structure and magnetic interactions
There are four polymorphic forms, α-, β-, γ- and δ-phases, known for p-NPNN (Allemand et al. 1991); Awaga et al. 1989a; Turek et al. 1991). The crystallographic data of these phases are summarized in table 1. Among them the orthorhombic β-phase is most stable, and the other phases gradually transform into the β-phase when maintained around room temperature. The molecular arrangement on the ac-plane of the β-phase crystal is shown in figure 2a. The molecules on the [ac]-plane are arranged in a parallel manner with the long molecular axis along the a-axis. Since the crystal belongs to the F2dd space group, the lattice can be divided into two face-centred orthorhombic sublattices, each deviating by 1/4a, 1/4b and 1/4c. Thus the crystal structure is similar to that of diamond or, more precisely, zincblende, as shown schematically in figure 2b, where the radical is denoted by an ellipsoid. All the molecules on the ac-plane at y = 0 are tilted in one way and those at y = 1/4b are tilted in the other way with respect to the ac-plane. The best-fit planes of the ONCNO moiety are tilted by [+ or -] 18.40° from the ac-plane, those of the phenyl rings by [+ or -] 68.45°, and those of the nitro groups by [+ or -] 84.70°.
The crystal structure at 6 K is also known from the neutron diffraction measurements (Zheludev et al. 19946). The crystal contracts thermally maintaining its crystal symmetry, and the lattice constants of a = 12.16, b = 19.01 and c = 10.71 A are reported. The contraction is largest along the c-axis (2.24%). As a result, the molecules are tilted at a greater angle than at room temperature. The tilt angles are [+ or -] 21.7°, [+ or -] 71.2° and [+ or -] 89.1°, respectively. These tilt angles are summarized in figure 2 c. These changes in the tilt angle indicate that the molecules, when the crystal is cooled to 6 K, undergo librational rotation by [+ or -] 3.3° about the a-axis in preservation of the molecular shape, only the nitro groups being further rotated internally by [+ or -] 1.
It is to be noted that the density of the β-phase crystal is, as shown in table 1, the largest of the four polymorphic phases. The density increases to ρc = 1.498 g cm-3 at 6 K.
The expected dominant exchange paths, J12, J13 and J14, are also shown in figure 2b by the broken, full and dotted lines, respectively. Theoretical calculation indicates that the first two paths are ferromagnetic and the third is slightly antiferromagnetic (Okumura et al. 1993).
4. Ferromagnetic interactions
The paramagnetic susceptibility of the β-phase crystal was first measured in 1989 (Awaga & Maruyama 1989). The temperature dependence of magnetic susceptibility is shown in figure 3 for the field direction along the b-axis. The low field susceptibility obeys the Curie- Weiss law χ = C/(T - Θ)) with a Weiss constant of Θ = +1 K in the temperature range above ca. 4 K, suggesting the presence of weak ferromagnetic intermolecular interactions. Since the Weiss constant is very small, the ferromagnetic interaction is checked by measuring the field dependence of the magnetization at low temperatures. As shown in figure 4, the magnetization grows more steeply at lower temperature (Tamura et al. 1991). This indicates that the spins are connected by means of ferromagnetic interaction. In addition, this experiment ensures that the sample is not contaminated with a ferromagnetic impurity.
5. Transition to the ferromagnetic ordered state
In 1991, the transition towards the ferromagnetic ordered state was discovered in a β-phase crystal by the measurements of AC susceptibility and heat capacity (Tamura et al. 1991; Nakazawa et al. 1992). The results of these measurements are shown in figure 5. The heat capacity has a λ-type sharp peak at the critical temperature of Tc = 0.60 K and reveals the existence of a transition. The corresponding entropy amounts to 85% of Rln 2 in the range up to 2 K, and the transition is magnetic and bulk in nature.
As the AC susceptibility diverges at around Tc, the ordered state is, without doubt, a ferromagnetic state. In fact, the magnetization curve at 0.44 K traces a hysteresis loop characteristic of ferromagnetism, as shown in figure 6. The magnetization is almost saturated at an applied field as low as ca. 5 mT and the coercive force is quite small. The rapid saturation suggests a small magnetic anisotropy in the system. The g-factors observed in the paramagnetic resonance experiments are ga = 2.0070, gb 2.0030 and gc = 2.0106. The linewidth is also almost independent of field direction at room temperature.
6. Evidence for ferromagnetism
Further evidence for ferromagnetism has been provided by various experiments such as the measurements of the temperature dependence of heat capacity in applied magnetic fields of various strengths (Nakazawa et al. 1992), the zero-field muon spin rotation (Uemura et al. 1993; Le et al. 1993; Blundell et al. 1995), the ferromagnetic resonance (Oshima et al. 1995a, b), the neutron diffraction (Zheludev et al. 1994a, b) Land the pressure effect on the magnetic properties (Takeda et al. 1995, 1996; Mito et al. 1997).
(a) Heat capacity in a magnetic field
The heat-capacity temperature dependences at various magnetic field strengths are illustrated in figure 7 (Nakazawa et al. 1992). The sharp peak in the zero field is slightly rounded, and is shifted to the higher-temperature side as the magnetic field is increased. This is a feature of ferromagnetic materials. For ferromagnetic substances, the critical temperature cannot be defined in a finite magnetic field. When there is a ferromagnetic interaction among the spins, they have a tendency to align themselves in parallel along the magnetic field at very low temperatures and the spin system is ordered by a weak field slightly above the ferromagnetic transition temperature. Thus the sharp peak of the heat capacity shifts and becomes rounded as in the paramagnetic region. In the case of antiferromagnetic order, the peak remains, up to a certain field strength. Therefore, this experiment ensures the ferromagnetism of the β-phase crystal below 0.6 K.
(Continues...)
Excerpted from Metal-Organic and Organic Molecular Magnets by P. Day, A. E. Underhill. Copyright © 1999 The Royal Society of Chemistry. Excerpted by permission of The Royal Society of Chemistry.
All rights reserved. No part of this excerpt may be reproduced or reprinted without permission in writing from the publisher.
Excerpts are provided by Dial-A-Book Inc. solely for the personal use of visitors to this web site.