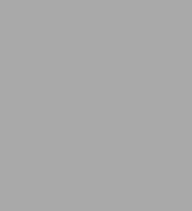
Medicinal Applications of Coordination Chemistry
353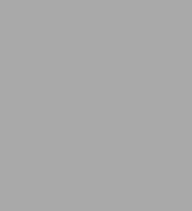
Medicinal Applications of Coordination Chemistry
353Hardcover
-
SHIP THIS ITEMNot Eligible for Free ShippingPICK UP IN STORECheck Availability at Nearby Stores
Available within 2 business hours
Related collections and offers
Overview
Product Details
ISBN-13: | 9780854045969 |
---|---|
Publisher: | RSC |
Publication date: | 02/12/2007 |
Series: | Rsc Paperbacks |
Pages: | 353 |
Product dimensions: | 6.14(w) x 9.21(h) x (d) |
About the Author
Read an Excerpt
Medicinal Applications of Coordination Chemistry
By Chris Jones, John Thornback
The Royal Society of Chemistry
Copyright © 2007 The Royal Society of ChemistryAll rights reserved.
ISBN: 978-0-85404-596-9
CHAPTER 1
Introduction
1.1 Metals in Medicine – A Historical Perspective
The use of particular metals, or their compounds, in medicinal preparations can be traced back for thousands of years. Copper sulfate and alums were among the many substances used by the ancient Egyptians to prepare potions, possibly because they had a sterilising effect on the concoction produced. In Arabia and China gold preparations appear to have been used by physicians as long ago as 2500 BC and, more recently, mercury was used to treat syphilis during the European epidemic of the late 15th and early 16th centuries. Aqueous suspensions of gold flakes known as Goldschlager or Geldwasser have also been used in medicinal preparations, although there is no proven medical value in the ingestion of metallic gold. Koch's observation of the bactericidal action of gold cyanide in 1890 offered a more scientific basis for the use of gold in pharmacy. However, gold compounds were subsequently found to be ineffective in the treatment of pulmonary tuberculosis. A more successful application followed in the 1930s when gold drugs were used to treat rheumatoid arthritis. In this case double-blind studies showed them to be effective for many, though not all, patients. Earlier, in 1909, Erlich had introduced the arsenic compound Salvar-san for treating syphilis. This was followed by another arsenic compound, mapharsen, and in 1921 bismuth compounds were also introduced and used in combination with mapharsen to treat syphilis. These pharmaceuticals, particularly those involving arsenic, could have severe side effects and no doubt this contributed to a common perception that metals are generally toxic and not well suited to use in pharmaceuticals.
In the second half of the 20th century, two elements in particular played a large part in arousing much greater interest in the medicinal use of metal compounds; one of these was platinum and the other technetium. In Michigan State University in 1964, Barnett Rosenberg was investigating the effect of electric fields on the growth of bacteria and made a quite serendipitous discovery that some platinum compounds could inhibit cell division. This observation led on to the development of the platinum compound cisplatin, which was approved by the US Food and Drug Administration (FDA) in 1978 for use in the treatment of ovarian and testicular cancer. Cisplatin had been known for over 100 years previously but its medicinal potential remained unrecognised until Rosenberg's investigations. Since then other second generation platinum drugs have followed, including compounds suitable for oral administration. The discovery of cisplatin has also stimulated research into a variety of other metal compounds with tumorocidal properties and the potential to become clinically useful anticancer drugs.
Beyond these innovations in therapy, the man-made element technetium began to make an important contribution to diagnostic medicine during the later part of the 20th century. Technetium was first identified by C. Perrier and E. Segré in 1937, being found in molybdenum targets after bombardment with deuterium nuclei in a cyclotron. All forms of technetium are radioactive and one form in particular has nuclear properties which make it particularly suitable for use in diagnostic medicine. This form emits γ-rays which, when originating from a source within the body, pass through living tissues and can be detected externally. This allows an image to be created of the distribution of the technetium γ-ray source within the body. Fortuitously, technetium also has a rich and versatile chemistry allowing it to be incorporated into many different kinds of compound. This allows the use of chemical compounds with affinities for different specific organs or tissue types to selectively transport technetium to specific locations in the body. In this way images of diseased or damaged regions can be obtained without the need for invasive surgical examinations. Other radioactive elements can also be used in non-invasive diagnostic imaging procedures but technetium has become pre-eminent in this application.
The use of radioactive elements as components of drugs suitable for use in therapeutic medicine offers a rather greater technical challenge than that posed by diagnostic imaging applications. However, recently the chemistry of metals has even begun to bear fruit in this difficult arena. It is over 100 years since Paul Erlich envisioned the development of a 'magic bullet', a dye carrying a toxic heavy metal which would target disease causing agents while leaving healthy tissue unharmed. He had developed the technique of staining tissue types with dyes (1877-1890), shown that a dye could kill trypanosomes infecting blood (1907) and prepared the arsenic compound Salvarsan to kill syphilis spirochetes (1909). The 'magic bullet' idea was visionary extension of these developments but the means to properly realise it did not exist at that time. Radioactivity was still a newly discovered phenomenon at the end of the 19th century, although its potential for use in therapeutic medicine was recognised around 1911. Radium preparations were used to treat various ailments including tumors, e.g. when inserted in vials for cervical cancer treatment at the Holt Radium Institute in Manchester. It was almost half a century later (1953) before Korngold and Pressman showed that antibodies labelled with radioactive iodine could be localised in tumors in rats. The use of radioactive emissions to kill tumors, rather than using a chemical toxin, offered the advantage that the pharmaceutical did not have to be internalised by the cell to exert its toxic effect. However, the necessary tumor specific antibodies were difficult to obtain in any quantity, restricting the clinical viability of the approach. It was further quarter of a century before Köhler and Milstein (1975) fused B-cells producing antigen specific antibody with myeloma cells to form hybrid cells expressing antibodies specific to a single target. Even then the development of immortalised monoclonal antibody cell lines did not address the problems of loading sufficient antibody onto the target tissue within an acceptable timescale, and with sufficiently rapid clearance from non-target tissue. Further developments in immunology, including the ability to manipulate fragment antibodies to obtain improved rates of uptake, were necessary for the 'nuclear magic bullet' approach to become viable. Unsurprisingly the first approvals for the clinical use of radiolabelled antibodies, or their fragments, were for diagnostic imaging purposes involving relatively low radiation doses to tissues. However, finally in 2002 Zevalin® (Ibritumomab) received FDA approval for treating types of B-cell non-Hodgkin's lymphoma. This compound contains a monoclonal antibody labelled with radioactive yttrium and heralds the clinical application of what is known as radioimmunotherapy using a radioactive metallic element. It seems that, for one type of disease at least, Erlich's 'magic bullet' had finally arrived.
Current medical practice has access to a variety of metal containing phar-maceuticals. In addition to the continued use of gold drugs to treat rheumatoid arthritis, lithium is now used to treat depression, platinum to treat certain types of cancer, bismuth to treat stomach ulcers, vanadium to treat some cases of diabetes, iron to treat anaemia, iron compounds to control blood pressure, cobalt in vitamin B to treat pernicious anaemia and certain radioactive metals to alleviate the pain of bone cancer. Beyond these therapeutic uses metals have also become important in diagnostic medicine, particularly diagnostic imaging applications. In addition to technetium, radioactive forms of thallium, gallium and indium are also used routinely for diagnostic imaging purposes. Another important diagnostic imaging technique, developed more recently, uses what is known as magnetic resonance to produce images of internal organs by examining the water content of the tissues involved. Metals with magnetic properties, particularly gadolinium, are finding use as a means of enhancing some of the images produced by this method. In addition to these examples various other metal compounds, still in a preclinical research and development phase, show promise for clinical use in therapeutic and diagnostic applications.
1.2 Metals and Human Biochemistry
The special chemical properties of metals have long been exploited by biological systems and various metals are essential for human health. However, despite their biological importance, metals nonetheless constitute a rather small proportion of living organisms. The human body is mostly water and an elemental analysis of a typical individual (Figure 1) reveals that hydrogen, oxygen, carbon and nitrogen together account for just over 99% of the atoms present. Calcium and phosphorus make up much of the remainder being the primary constituents of bone, together with oxygen and some hydrogen. A particular characteristic of metals in aqueous media, contributing to their biological importance, is that they carry a positive charge. This distinguishes them from most organic species which, even when uncharged, tend to be more negative in character often attracting positively charged species in solution.
The metals present in the human body can be crudely divided into two types, bulk metals and trace metals. These two types share some chemical similarities but the trace metals introduce additional chemical characteristics beyond those displayed by the bulk metals sodium, potassium, magnesium and calcium. The bulk metals are present in relatively larger amounts compared to the trace metals (Figure 1) and have various biological rôles. Apart from its structural function in bone calcium, together with sodium and potassium, is involved in signal transmission through nerves. Calcium is also important in triggering actions, e.g. in muscles, while magnesium is found in some phosphohydrolase and phosphotransferase enzymes. Sodium and calcium are important extracellular bulk metals while potassium and magnesium are the major cellular metals. As a group, the bulk metals share broadly similar chemical properties but differ in the sizes and charges of the forms in which they exist in aqueous media. These differences are reflected in the properties of the metals so that sodium, for example, can be discriminated from potassium, which is larger in size, and from calcium which has a higher charge. The bulk metals are chemically promiscuous and generally do not form long-term associations with any particular entity, such as a protein. Rather they will usually move between hosts being quite rapidly exchanged. Compared to the trace metals their chemical reactivity is more limited and they remain in the same positively charged state throughout their time in the body.
In contrast with bulk metals, the trace metals show more varied chemical reactivity and, with the exception of zinc, can change the magnitude of their positive charge under the right conditions. The trace metals are usually found incorporated in a biological host entity such as a protein with which they will have a comparatively long-term relationship. Taken together the trace metals iron, zinc, copper, nickel, chromium, manganese, molybdenum, cobalt and vanadium barely constitute 0.01% by mass of the human body (Table 1). Despite this they are essential elements and any deficiency can have serious adverse consequences for health. Trace metals are involved in a wide variety of biochemical processes including the transport and storage of dioxygen, the dissolution of carbon dioxide in blood, the extraction of energy from foodstuffs, the breakdown of proteins, the removal of harmful species such as superoxide and many other chemical transformations. They can also have structural functions such as controlling the folding of proteins. While sharing some characteristics with the bulk metals as a result of their positive charges, the trace metals also possess additional chemical capabilities enabling them to participate in a wider range of chemical processes. For example, zinc is present in the enzyme carbonic anhydrase to catalyse the conversion of gaseous carbon dioxide to dissolved carbonic acid, while iron is present in hemoglobin and myoglobin to reversibly bind dioxygen. Iron is also found in systems, which add oxygen to organic substrates or transfer energy between biological components in metabolic energy transfer chains. Cobalt, in the form of vitamin B coenzyme, is used to carry out chemical transformations associated with structural changes in carbon-compounds, and copper is present in some super-oxide dismutase enzymes to convert superoxide to dioxygen and peroxide. In each case the metal brings its own distinctive properties to the task and these are exploited by the biochemical system in which the metal participates.
Living organisms require efficient systems for managing metals. The bulk metals tend to be quite freely mobile but selective means of transporting them across cell membranes are necessary to maintain a delicate electrolyte balance and for nerve cells to function efficiently. Trace metals may be transported and stored by proteins. In particular iron is transported by transferrin and stored in ferritin while copper can be transported and stored in ceruloplasmin. Metals are also stored in the proteins, e.g. cytochromes or enzymes, within which they express their biological function. Some clinical applications of the chemistry of metals are concerned with the management of metals in the body. Metal containing pharmaceutical formulations can be used to treat deficiencies of a particular metal, e.g. iron for anaemia or cobalt as vitamin B12 for pernicious anaemia. Conversely the chemistry of metals may be exploited in treating problems arising from an excess of a metal in tissues, e.g. copper in Wilson disease or iron overload in patients receiving repeated blood transfusions. One approach to the treatment of iron overload exploits a natural microbial iron-sequestering agent. Since iron in the environment is generally found in a rather insoluble and inaccessible form, some micro-organisms excrete agents known as siderophores which strongly bind iron and facilitate its absorption into the micro-organism. Agents of this type can be adapted to bind iron in the body and promote its excretion providing an example of the application of bio-inorganic chemical knowledge in a clinical application.
Beyond clinical applications which relate to the natural utilisation of trace metals, others exploit quite unnatural and non-physiological features of metal chemistry. As examples the toxicity of platinum complexes is managed and targeted in treatments involving anticancer drugs such as cisplatin while, in diagnostic medicine, the differing biodistributions of various technetium complexes can be exploited in imaging applications.
1.3 Metallopharmaceuticals
1.3.1 General Requirements
Metals may not seem an obvious choice as components of pharmaceuticals and it is a common perception that metal compounds are toxic, unstable and generally not well suited for pharmaceutical applications. Certainly in some historic uses of metal compounds, the treatment may have been as dangerous as the disease and early beneficial applications exploited the microbiocidal properties of metals such as mercury, arsenic and bismuth. Pharmaceuticals are more usually expected to be organic carbon based compounds, e.g. aspirin or penicillin, these being relatively unreactive chemically, often uncharged and amenable to structural variation to optimise their medicinal properties. However, over the past 30 years the particular chemical reactivities of metals, their magnetic and nuclear properties and the structural variety of their compounds, have become important in a variety of medical applications. Although not exactly Erlich's 'magic bullet', the organ specific uptake of technetium radio-pharmaceuticals and the highly specific nature of the binding of cisplatin to DNA demonstrate the potential of this class of compound in specific medicinal applications.
In order to be useful in medicine, chemical compounds need to meet a variety of criteria. The most obvious requirement is that they must exhibit a medically beneficial effect with minimal toxic side effects. The relative benefit of a drug compared to its toxicity can be expressed in terms of its therapeutic index. This can be defined as the ratio of the dose required to kill 50% of test animals (LD50) to the dose required to produce an effective therapeutic response in 50% of test animals (ED50), i.e. Therapeutic Index = LD 50/ED50. A high therapeutic index is clearly desirable, indicating that a relatively large dose is required to produce a toxic effect compared to that required to produce the therapeutic effect. However, because LD50 values cannot be obtained using human subjects, the therapeutic index can only have limited value for comparing different test compounds as it cannot be based on wholly human data.
As the dose of a compound with some medically beneficial effect is increased, and its in vivo concentration in the subject rises, it may be expected that the therapeutic benefit will increase. However, at some point this benefit will start to be negated by toxic effects until the dosage becomes so high as to be positively detrimental to the patient (Figure 2). Thus there will be a particular concentration range, associated with a particular dosage regime, which maintains the desired therapeutic effect. (A similar argument could be applied to foodstuffs. As an example carrots, a source of vitamin A, will produce a beneficial effect in normal dietary amounts. However, excessive consumption can lead to chronic or acute toxic effects, particularly in the liver where vitamin A accumulates. Those with liver damage, e.g. from over consumption of alcohol, are especially at risk). Typically the concentration of a drug will rise after administration then fall again as the compound is processed in the body and excreted. The timescale over which this process takes place, and any tendency for the drug to accumulate, will affect the dose regime necessary to maintain concentrations within the optimal therapeutic range.
(Continues...)
Excerpted from Medicinal Applications of Coordination Chemistry by Chris Jones, John Thornback. Copyright © 2007 The Royal Society of Chemistry. Excerpted by permission of The Royal Society of Chemistry.
All rights reserved. No part of this excerpt may be reproduced or reprinted without permission in writing from the publisher.
Excerpts are provided by Dial-A-Book Inc. solely for the personal use of visitors to this web site.
Table of Contents
Preface; Chapter 1: INTRODUCTION; 1.1: METALS IN MEDICINE - A HISTORICAL PERSPECTIVE; 1.2: METALS AND HUMAN BIOCHEMISTRY; 1.3: METALLOPHARMACEUTICALS; 1.4: THE SPECIAL PROPERTIES OF METALS; Chapter 2: THE CHEMISTRY OF METALS IN A NUTSHELL; 2.1: THE STRUCTURE OF MATERIALS; 2.2: THE STRUCTURES AND PROPERTIES OF ATOMS; 2.3: THE FORMATION OF COMPOUNDS; 2.4: THE CHEMISTRY OF METALS - AN OVERVIEW; 2.5: BONDING IN METAL COMPLEXES; 2.6: THE MAGNETISM AND SPECTROSCOPY OF METAL COMPLEXES; 2.7: THE ENERGETICS OF METAL COMPLEX FORMATION; 2.8: REACTIONS AT METAL CENTRES; 2.9: REDOX POTENTIALS; 2.10: CONCLUSIONS; Chapter 3: DIAGNOSTIC MEDICINE; 3.1: INTRODUCTION; 3.2: ANATOMICAL IMAGING; 3.3: FUNCTIONAL IMAGING; 3.4: In vitro APPLICATIONS; Chapter 4: THERAPEUTIC MEDICINE; 4.1: THE USE OF METAL COMPOUNDS IN THERAPY; 4.2: CHELATION THERAPY; 4.3: CANCER THERAPY; 4.4: RHEUMATOID ARTHRITIS; 4.5: DIABETES; 4.6: CARDIOVASCULAR SYSTEM; 4.7: THERAPEUTIC RADIOPHARMACEUTICALS; Chapter 5: METALLOPHARMACEUTICAL DESIGN; 5.1: INTRODUCTION; 5.2: STRUCTURE ACTIVITY RELATIONSHIPS; 5.3: FUTURE DIRECTIONSWhat People are Saying About This
The section on platinum, like the others, is in general well illustrated with line diagrams of chemical structures, and gives a good coverage of new developments, such as active trans complexes and potent di- and tri-Pt anticancer complexes. This book will serve a useful role in teaching inorganic chemistry to both undergraduate and postgraduate students. The topic helps to 'bring inorganic chemistry to life' and in my experience does arouse their curiosity at all levels. It will be a useful addition to libraries.