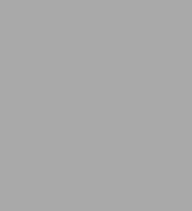
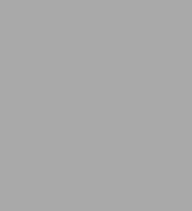
Hardcover
-
PICK UP IN STORECheck Availability at Nearby Stores
Available within 2 business hours
Related collections and offers
Overview
Product Details
ISBN-13: | 9780854045709 |
---|---|
Publisher: | RSC |
Publication date: | 12/10/1997 |
Series: | RSC Analytical Spectroscopy Series , #5 |
Pages: | 186 |
Product dimensions: | 6.40(w) x 9.30(h) x 0.70(d) |
Read an Excerpt
Ionization Methods in Organic Mass Spectrometry
By Alison E. Ashcroft
The Royal Society of Chemistry
Copyright © 1997 The Royal Society of ChemistryAll rights reserved.
ISBN: 978-0-85404-570-9
CHAPTER 1
Introduction
1 An Introduction to Mass Spectrometers
Although it is beyond the scope of this book to delve deeply into the theory and physics of mass spectrometers, a brief introduction would appear to be necessary, not only to clarify the nomenclature used for the various techniques and hardware described in the remainder of this monograph, but also to encourage the reader to turn to more complete texts on the subject.
A mass spectrometer, like Caesar's Gaul, can be divided into three fundamental parts, namely the ionization source, the analyser, and the detector (see Figure 1.1). Mass spectrometers are used primarily to provide information concerning the molecular weight of a compound, and in order to achieve this, the sample under investigation has to be introduced into the ionization source of the instrument. In the source, the sample molecules are ionized (because ions are easier to manipulate than neutral species) and these ions are extracted into the analyser region of the mass spectrometer where they are separated according to their mass (m) to charge (z) ratios (m/z). The separated ions are detected and the signal fed to a data system where the results can be studied, processed, and printed out. The whole of the mass spectrometer (except for Atmospheric Pressure Ionization sources) is maintained under vacuum to give the ions a good chance of travelling from one end of the instrument to the other without any interference or hindrance. Nowadays the entire operation of the mass spectrometer and often the sample introduction process are usually under complete data system control and the operator hardly needs to move away from the computer terminal to perform the sample analyses.
Many ionization methods are available and each has its own advantages and disadvantages. The method of ionization used depends on the sample under investigation, the type of mass spectrometer being used, and the available equipment. This book describes the more commonly encountered ionization methods, and aims to provide an account of their set-up and basic operation. Once the ionization method has been set up and has been shown to be operating at its optimum performance, then the operator can start to develop the technique for the particular samples under scrutiny. The optimum performance of any ionization method will depend on the performance and condition of the mass spectrometer, the reliability of any other equipment and materials involved, including gas and liquid chromatographs and chromatography columns, the purity of any solvents or gases used, and the quality of the standard samples. However, it should be remembered that the optimum performance must be established before any sample analyses are undertaken, and this performance should be verified every day, or more frequently if laboratory procedures dictate or if problems are suspected. If the performance is not as good as expected then steps should be taken to retrieve any losses in sensitivity or resolution.
As well as there being a good choice of ionization methods, there are also many different ways of introducing samples into the ionization source depending on the ionization method being used and the type of samples under investigation. For example, single-substance samples can be inserted directly into the ionization source by means of a probe whereas complex mixtures will benefit from some kind of chromatographic separation en route to the ionization source, and this could involve interfacing liquid chromatography (LC), gas chromatography (GC), supercritical fluid chromatography (SFC), or capillary electrophoresis (CE) to the mass spectrometer. The methods of interfacing to the various ionization methods are described in more detail in the relevant chapters.
After the ionization source, the ions proceed to the analyser region, and a mass spectrometer is generally classified by the type of analyser it accommodates. There is a variety of analysers, and the ones referred to in this book are those that are most frequently encountered in organic mass spectrometry, namely the magnetic sector, the quadrupole, and the time-of-flight. Each will be discussed in a little more detail later in this Chapter (see Chapter 1, Section 2). Not all ionization methods are compatible with all of these analysers, as will be revealed where appropriate in the text.
The detector could be one of several possibilities including inter alia photo-multipliers, electron multipliers, microchannel plates, and diode array detectors. On a day-to-day basis, the detector gain should be set at the appropriate level for acquiring data.
The remainder of this Chapter aims to provide a brief overview of a range of mass spectrometers and indicate which ionization methods are appropriate. I have tried to summarize the various ionization methods, instruments, and sample introduction methods in several different ways so that these summaries can be referred to at a later date when reading the more detailed chapters to help put the various topics in perspective. The summaries may appear to overlap, and if this is so, I apologize; it was simply my intention to display the data in a readily accessible manner, emphasizing different significant aspects so that the text would appeal to a variety of readers.
2 The Mass Analyser
Introduction
Resolution
The main function of the mass analyser is to separate, or resolve, the ions formed in the ionization source by their mass to charge ratios (m/z).
The resolution (R) of a mass analyser, or its ability to separate two peaks, is defined as the ratio of the mass of a peak (M1) to the difference in mass between this peak and the adjacent peak of higher mass (M2) (see Figure 1.2), i.e.:
R = M1/M2 - M1 (1.1)
where R = resolution,
M1 = the mass of a peak, and
M2 = the mass of an adjacent, higher mass peak.
In the simplest terms, a singly charged ion at m/z 1000 could be separated from another singly charged ion at m/z 1001 if a resolution of 1000 is available. Similarly, a singly charged ion at m/z 2000 would require a resolution of 2000 to separate it from a second singly charged ion at m/z 2001, whereas a singly charged ion at m/z 100 would need only 100 resolution to separate it from another singly charged ion at m/z 101.
Resolution, when referring to magnetic sector mass spectrometers, is often described by the 'valley definition' where a 'resolution of 10% valley' (see Figure 1.2) means that two peaks of equal intensity are considered resolved when the height of the valley between the peaks is 10% of the peak height. Alternatively, and less frequently, one may allude to a resolution of 50% valley. Quadrupole and time-of-flight mass spectrometers are generally less able to provide high (or better than unit) resolution, although recent advances with time-of-flight instruments have led to improvements. In such cases, a peak width can be described instead; for example, one might say the sample was analysed with a peak width of 0.5 amu measured at half of the maximum height of the peak, or 0.5 amu FWHM (full width half maximum).
Isotope Distributions
In general the resolution actually required for most analyses is such that the singly charged isotope patterns of the detected ions are readily discernible, and for applications involving molecular weights ca. 1500 da or less, this can be provided by magnetic sector, quadrupole, and time-of-flight mass spectrometers.
If one considers a small organic compound of molecular formula C19H38O2, then under electron impact (EI) ionization conditions (see Chapter 3) with unit resolution set for the analyser, a molecular ion (M+*) is generated at m/z 298 (see Figure 1.3) which relates to the intact molecules (less one electron) in which all the atoms are the lowest mass (and in this case the most abundant) isotopes (i.e.12C, 1H, and 16O). This value can be taken to be the molecular weight of the compound. There will also be lower intensity ions at m/z 299, which correspond to molecules of the same compound in which one 12C atom has been replaced by a less abundant, and therefore less probable, 13C isotope. The relative intensities of these two ions should relate to the natural abundances of the isotopes multiplied by the number of carbon atoms in the molecule. In other words, the intensity of the m/z 299 ion compared to the m/z 298 ion should be equal to 1.11 (because the natural abundance of 13C is 1.11% of the natural abundance of 12C) multiplied by 19, which equals 21.09%. For higher molecular weight samples which contain more carbon atoms, the probability of one of the 12C atoms having been replaced by a 13C atom increases, and indeed when the number of carbon atoms in a molecule reaches 90, it becomes more probable to find a molecule with one 12C atom replaced by a 13C atom, than to find a molecule with all its carbon atoms of the 12C type. The isotope distribution for a compound of theoretical molecular formula C100H202 is shown in Figure 1.4 to illustrate this.
If the sample under investigation is already known, then the theoretical molecular weight can be calculated from the molecular formula of the compound. If the average atomic masses from the periodic table are used for this purpose, an accurate, but average molecular weight of 298.5095 daltons (da) results for the above sample of molecular formula C19H38O2. If unit resolution has been set, this will not be the mass of the ion detected and reported by the mass spectrometer. Remember that because mass spectrometers separate ions according to their m/z ratio, so the isotopes of the atoms should be taken into account when calculating the molecular formula of a compound. The dominant ion in this particular molecular weight cluster is the 12C191H3816O2 ion, whose accurate but monoisotopic molecular weight is 298.2872 da. Figure 1.5 presents a list of some of the most commonly encountered atoms together with their monoisotopic and average masses.
If a mass spectrometer has been properly calibrated, then the mass accuracy should be good to at least 0.1 da. In the sample described previously, C19H38O2, the difference between the average and monoisotopic molecular weights is not great and indeed both have the same nominal mass; in this case the spectrum could have been interpreted equally well regardless of whether the operator had used monoisotopic or average values for the calculations. This is not always the case though, and so care should be exercised, especially when dealing with high molecular weight samples (> 2000 da), or with samples that exhibit irregular isotope patterns such as those containing chlorine, bromine, or transition metal atoms such as nickel and zinc. As an example, if the average and monoisotopic accurate masses are calculated for a sample of molecular formula C18H12Cl2FNO4S, values of 428.2674 and 426.9848 respectively are obtained. Now there is a significant difference between the two calculations, and a mass spectrum that produced a molecular ion at m/z 427 would quite correctly be consistent with the monoisotopic calculation, but would indicate (mistakenly) that the sample was not as expected if the average masses had been used.
The two halides chlorine and bromine each have two isotopes separated by two mass units; chlorine consists of 35Cl and 37Cl in the approximate ratio 3:1, and bromine consists of 79Br and 81Br in approximately equal ratios. This produces in both cases a distinctive and readily recognisable pattern which is a good aid for compound identification. If a compound has more than one bromine or chlorine atom, or one or more of each, then the isotope pattern increases in complexity and distinction, as shown in Figure 1.6.
Finally, the expected isotope pattern for an organometallic compound of molecular formula C24H54Br2NiP2 is illustrated in Figure 1.7 to give an idea of the complexity involved with some samples, and to emphasize the necessity for correctly calculating the masses of the isotopes in order to be able to interpret the data properly.
Most mass spectrometers will resolve ions with unit resolution up to at least 2000 da, and so monoisotopic atomic masses are used in these cases. Above 2000 da, the resolution should be checked and if it is insufficient to resolve adjacent isotopes, then average atomic masses are used in calculations.
Accurate Mass Measurements
Occasionally the nominal molecular weight of a sample, as determined with an accuracy of say, 0.1 da from the mass spectrum, is not sufficient to characterize the sample. This is especially true if the sample is an original one whose molecular formula has to be validated, or if there is a chance that the sample could have one or more structures which have the same nominal but different accurate masses. For example, the formulae C21H3603 and C19H32N2O3 have monoisotopic masses of 336.2664 and 336.2413, respectively. The mass spectrum for this sample could indicate a molecular weight for the compound of 336.2 da, but from this information, it is not possible to say which formula is the correct one; both fit the data equally well. Therefore an accurate mass measurement is required, which should provide a measurement within 5 parts per million (ppm) error of the correct answer. Accurate mass measurements require due care and attention in their operation. A suitable reference material needs to be used, and a means of maintaining the reference in the source simultaneously with the sample must be sought. For electron impact analyses a volatile reference material such as heptacosa is often admitted into the ionization source through a permanently sited reference inlet, whilst the sample is introduced into the source by means of a probe, or eluting from a GC column.
If two alternative formulae can be proposed from the nominal molecular weight obtained from the mass spectrum of the compound, and if both are expected to be present in the same sample, then the resolution required for their m/z separation should be calculated so that the resolution on the mass spectrometer can be set before the experiment is initiated. In the example above, the resolution R needed is given by:
336.2413/336.2664-336.2413 = 13 396
The mass spectrometer should be set to provide at least this amount of resolution if the experiment is to separate these two structures. In general, resolution above 2000 (10% valley definition) requires the use of a magnetic sector mass spectrometer.
Accurate mass measurements can be made at any resolution; resolution is the criterion to be considered when separating masses.
Methods of Using the Mass Analyser
There are different methods of acquiring data when using a mass spectrometer, and these should be taken into account when designing an experiment. The most usual method of acquiring data is by scanning the mass analyser over an appropriate m/z range, thus producing a mass spectrum from which (hopefully) molecular or quasimolecular (molecular related) ions will provide an indication of the molecular weight of the sample. If the sample has fragmented (fallen to pieces) in the ionization source, then these ions will also have been collected, and often the fragment ions can be studied and information regarding the structure of the sample pieced together. Almost all samples are analysed with a full scanning experiment initially to produce as much information as possible about the sample, and full scanning acquisitions are possible with magnetic sector, quadrupole, and time-of-flight mass spectrometers. Under appropriate conditions, accurate mass measurements can also be carried out.
If the analyst is investigating known compounds which have been characterized previously, and wants to ascertain whether or not the expected compound is present, or needs to determine the concentration level of the sample in a biological or ecological matrix, then often a selected ion recording (SIR) analysis is performed. Before this can be carried out, one or more significant and characteristic ions from the sample must be specified in the acquisition parameters. These ions could be the molecular or quasimolecular ions, for example, and/or intense, diagnostic fragment ions. The mass analyser will then monitor the specified ions by switching from one to the next. This technique is more sensitive than a full scanning one, because all the available time is spent on the ions of interest rather than monitoring all the ions over a stipulated m/z range. The sensitivity is highest if only one ion is monitored, but care must be taken to ensure that no other isomeric or isobaric compounds are present in the same sample. A good compromise is to monitor two or three ions for each compound under scrutiny, as this gives good sensitivity while providing more credence to the results.
(Continues...)
Excerpted from Ionization Methods in Organic Mass Spectrometry by Alison E. Ashcroft. Copyright © 1997 The Royal Society of Chemistry. Excerpted by permission of The Royal Society of Chemistry.
All rights reserved. No part of this excerpt may be reproduced or reprinted without permission in writing from the publisher.
Excerpts are provided by Dial-A-Book Inc. solely for the personal use of visitors to this web site.