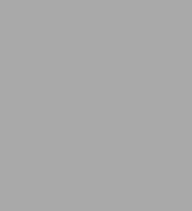
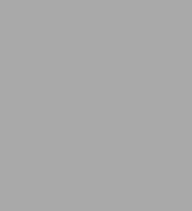
eBook
Available on Compatible NOOK devices, the free NOOK App and in My Digital Library.
Related collections and offers
Overview
Scientific American's daily Sixty-Second Science podcast was such an unexpected success, with millions of downloads, that a spin-off site was created around the concept of bite-sized science. This new series of books will tackle the biggest topics in science by breaking them up into quick and easy two- to four-page spreads. Topics in each book will take the light and accessible tone of the 60-Second Science podcasts and blog. INSTANT EGGHEAD PHYSICS will explore quantum physics, relativity, and light. It will break down complex ideas and explore why Einstein made some big blunders, how the ipod came to be, and what it would take to make teleportation possible.
Product Details
ISBN-13: | 9781429968928 |
---|---|
Publisher: | St. Martin's Publishing Group |
Publication date: | 11/10/2009 |
Series: | Instant Egghead Guides |
Sold by: | Macmillan |
Format: | eBook |
Pages: | 240 |
File size: | 442 KB |
About the Author
Brian Clegg is the author of A Brief History of Infinity, The First Scientist: A Life of Roger Bacon, and Light Years: The Extraordinary Story of Mankind's Fascination with Light, and Upgrade Me: Our Search for Human 2.0. He holds a physics degree from Cambridge and has written regular columns, features, and reviews for numerous magazines. He lives in Wiltshire, England, with his wife and two children.
BRIAN CLEGG is the author of Ten Billion Tomorrows, Final Frontier, Extra Sensory, Gravity, How to Build a Time Machine, Armageddon Science, Before the Big Bang, Upgrade Me, and The God Effect among others. He holds a physics degree from Cambridge and has written regular columns, features, and reviews for numerous magazines. He lives in Wiltshire, England, with his wife and two children.
Scientific American is the award-winning authoritative source for the science discoveries and technology innovations that matter.
Read an Excerpt
CHAPTER 1
MATTER
STUFF
THE BASICS
Physics, the topic of this book, is the fundamental science. The great physicist Ernest Rutherford once said, "All science is either physics or stamp collecting." He meant that most other science at the time was about collecting and categorizing information. Physics explained how the universe works.
In this section, the focus is stuff. What's this book — or you — made of? Is everything made of the same kind of stuff? Why is some stuff hard and some fluid? How do you turn one kind of stuff into another?
To the modern mind, stuff is obviously made up of atoms, assembled out of tiny components like a vast LEGO structure. Yet this isn't obvious. Look at a glass of water. Both the glass and the water inside seem to be continuous substance, quite unlike anything we're familiar with that's made up of small components. To understand matter we have to go beyond the obvious, to see in our minds what we can't experience with our senses, and that's part of the fun of physics.
ON THE FRONTIER
An atom is the single smallest particle of an element. It is as small as you can get and still have a bit of that substance. Molecules contain more than one atom, joined together. This can be a pure element. For example, a molecule of oxygen contains two oxygen atoms, joined together. But it can also contain several elements, whether it's a simple molecule such as sodium chloride or the immensely long DNA chains that make up the complex molecules of life.
COCKTAIL PARTY TIDBITS
* One ancient Greek theory on matter suggested that you could cut stuff up into smaller and smaller pieces until eventually you could cut no more. What was left was uncuttable — in Greek, atomos — atoms.
* If you could squeeze all the matter in your body together, removing the empty space, it would pack into a cube less than a thousandth of an inch per side.
BROWNIAN MOTION
THE BASICS
Atoms are like small children: they are never entirely still. It's a remarkable contrast between the visible world and the world of the very small. Look at a glass of water. That water appears to be motionless, yet within the liquid the water molecules are frantically dancing.
In 1827 a Scottish botanist called Robert Brown was studying pollen grains of an evening primrose plant, suspended in a drop of water under a microscope. The tiny specks of pollen jumped about, constantly in motion.
There seemed to be no order to the motion, no rules for the way they moved. Instead the pollen grains' dancing was chaotic. This jerky dance was named Brownian motion, but remained little more than an oddity until Albert Einstein linked it to the behavior of atoms.
ON THE FRONTIER
Einstein produced three great papers in 1905. His works on relativity and the photoelectric effect get the glory, but his third paper on Brownian motion was just as significant. Until that time, the concept of atoms was entirely theoretical. But Einstein showed that the dance of the pollen grains was caused by the random impact of billions of water molecules. Einstein used Brownian motion to show that the liquid the grains floated in was composed of many billions of gyrating molecules.
COCKTAIL PARTY TIDBITS
* When Robert Brown first saw Brownian motion he suspected it was the life source of a plant in action. It was only when he tried stone dust and soot and found the same effect with particles that were never alive that he confirmed that the size of the pollen grains was responsible for their motion.
* It wasn't until 1912 that French physicist Jean Perrin firmly established the existence of atoms. Until then, many scientists denied they existed.
ATOMIC STRUCTURE
THE BASICS
It was surprisingly soon after finding proof that atoms existed that the name "atom" proved to be inaccurate. Even as Brownian motion was showing atoms and molecules to be real, it was becoming obvious that atoms weren't uncuttable.
A British scientist, J. J. Thomson, discovered in 1895 that there was a negatively charged particle within the atom, which would be named the electron. He imagined atoms were like a pudding with raisins spread through it. The raisins were the electrons and the rest of the pudding was positively charged, balancing this out so the atom had no charge itself.
ON THE FRONTIER
Thomson's pudding model was blasted apart by New Zealand scientist Ernest Rutherford. Rutherford had the idea of firing other particles into an atom and seeing how they reacted, like throwing a ball repeatedly at an invisible object and working out its structure by seeing how the ball bounces off. The ball he used was an alpha particle, the nucleus of the helium atom. These particles could be detected when they hit screens painted with fluorescent material. If the atom had been as Thomson imagined, the powerful alpha particles should plow through. Most did, but occasionally one bounced back. This unexpected result made Rutherford realize that atoms have a small, dense, positively charged core to deflect the alpha particles. He established the familiar idea of the atom being like a solar system with a positive nucleus at the center and negative electrons floating around it.
COCKTAIL PARTY TIDBITS
* The nucleus is so much smaller than the atom — 10,000 times smaller than the whole — that it has been described as being like a fly in a cathedral.
* Remarkably, some scientists have seen an individual atom directly. In 1980 Hans Dehmelt of the University of Washington isolated a barium ion (an ion is an atom with electrons missing, or extra electrons added, giving it an electrical charge). When illuminated by the right color of laser light, the ion was visible to the naked eye as a pinprick of brilliance floating in space.
ISOTOPES AND NEUTRONS
THE BASICS
The picture of the atom as a miniature solar system was initially simple. At its heart was a nucleus of positively charged protons providing 99.9% of its weight. Far out from the nucleus flew an equal number of negative electrons. Between them the charge balanced out. It wasn't clear how those protons, which should repel each other, stuck together, but otherwise the model made sense.
In 1932, however, another type of particle was discovered in the nucleus, about the same weight as a proton but with no charge: the neutron. Neutrons proved invaluable in explaining why there are different versions of the same element called isotopes, chemically similar, but weighing different amounts.
The chemical properties of elements are caused by the charged particles. But you can have versions of the same element with differing numbers of neutrons in the nucleus. This explains how chlorine, with 17 protons, can have an atomic weight (roughly the number of protons + neutrons) of 35.45. It doesn't have 181/2 neutrons; it's a mix of versions of the atom, some with 18 neutrons and some with 20.
ON THE FRONTIER
Some isotopes are radioactive, unstable, and spew chunks from their nucleus to become another element. The best known is uranium 235. The numeral "235" is the atomic weight; it has 92 protons and 143 neutrons. When uranium 235 decays, it gives off several neutrons, converting the atom from uranium to thorium 231 (itself unstable). In a nuclear reactor or a nuclear bomb, those neutrons then blast into other uranium nuclei, triggering further fission. Of itself, uranium 235 decays slowly; it takes around 700 million years for half a chunk to decay. It's only with such a chain reaction that nuclear energy can be useful.
COCKTAIL PARTY TIDBITS
* There are 92 naturally occurring elements from the lightest, hydrogen, to uranium, the heaviest.
* Since 1972, the remains of 15 natural nuclear reactors have been found. Around 1.7 billion years ago a stable nuclear reaction took place in underground deposits of uranium. Because the amount of uranium 235 in the ground drops as it decays, it is unlikely such natural reactors would be found now.
FORGET THE PLANETS
THE BASICS
If someone draws an image to represent an atom, chances are it will look like a solar system with the electrons neatly orbiting the nucleus. Just take a look at the logo of the International Atomic Energy Agency. The sad thing is, this model is wrong.
When you accelerate an electron it gives off bursts of light. Acceleration is a change in velocity (speed plus direction). The electron's speed would remain the same as it flew around the nucleus, but its direction would be constantly changing, which means it would be accelerating. Problem! Any orbiting electron should give off light, losing energy. It would hurtle into the nucleus to be destroyed, like a moth spiraling into a candle flame. This clearly isn't happening, or all our atoms would implode.
ON THE FRONTIER
Danish scientist Niels Bohr soon recognized this problem. He fixed it by putting his electrons on imaginary tracks. Instead of flying around the nucleus in any orbit, Bohr's tracks limited where an electron could be. Once on a track, the normal rules didn't apply: the imaginary track stopped energy leaking out. Electrons could jump from one orbit toanother — giving out or absorbing a photon of light — but could not live anywhere in between. It wasn't possible for an electron to drift down and crash into the nucleus; they could only make leaps between fixed orbits. These jumps between different tracks, gaining or losing a quantum of energy with each jump, were called quantum leaps. Bohr had taken the atom digital.
COCKTAIL PARTY TIDBITS
* Such was the power of the image of the atom as a miniature solar system that some fiction writers envisaged it as literally true, with each electron a planet that could be inhabited.
ANTIMATTER
THE BASICS
Fans of Star Trek know that the Enterprise is powered by antimatter, but antimatter is not fictional. It is like the familiar stuff that makes up our world, but every charged particle has the opposite charge. Instead of negative electrons, antimatter has positive positrons. Instead of positive protons in the nucleus, an anti-atom has negatively charged anti-protons.
Because it only differs in the placement of electrical charge, it's possible to do anything with antimatter that can be done with ordinary matter. You can build an anti-table or an anti-world. Antimatter has mass and behaves much as ordinary matter does. But don't expect to go out and buy some. Handling antimatter is tricky. When matter and antimatter get together, both are destroyed, converted into pure energy.
The simplest matter/antimatter reaction is when an electron and a positron collide. Their mass is converted into energy as two photons of light (gamma rays) according to Einstein's famous equation E = mc: the energy produced is equal to the mass of the particles multiplied by the square of the speed of light. Because of this tendency to annihilate, very little free antimatter is found.
ON THE FRONTIER
There is still a big debate about why there is so little antimatter. The Big Bang should have produced equal amounts of matter and antimatter, which then would wipe each other out, leaving a universe full of energy. That this didn't happen is usually explained by assuming that subtle differences in the properties of matter and antimatter meant that there was a little extra matter. As little as one particle in a billion may have survived the matter/antimatter wipeout. But that was enough.
Some have speculated that, instead, the universe became segmented, leaving vast pockets of antimatter, perhaps on the same scale as the observable universe.
COCKTAIL PARTY TIDBITS
* The amount of energy generated from matter/antimatter interactions is vast. One kilogram (2.2 pounds) of matter/antimatter would produce around 1017 joules (1 with 17 zeros after it). That's the energy output of a typical power station for six years.
QUARKS
THE BASICS
Protons and neutrons are no longer considered fundamental particles. Each is made up of three smaller particles, quarks. There's a whole mess of quarks distinguished by characteristics known as flavors (no, really): charm, strangeness, top/bottom, and up/down. The proton is two ups and one down; the neutron two downs and one up.
Up quarks have a 2/3 charge and down quarks –1/3, resulting in a positive charge of 1 for the proton and no charge at all for the neutron. We aren't used to nature coming up with quantities in thirds. But the unit of charge is arbitrary. We ought to say that up and down quarks have charges of 2 and – 1 respectively — so a proton has a charge of 3 units — but because protons and electrons were the simplest particles known when the units were established we are stuck with thirds.
ON THE FRONTIER
No one has ever seen a quark, nor broken a proton or neutron into its components. It is difficult to do so, because the force that holds quarks together gets stronger as they move apart. Quarks were first predicted by a purely mathematical formulation of quantum theory. The existence of quarks themselves has since been indicated by experiments that show three constituents in a proton, and by the short-lived production of particles made up of combinations of different quarks.
COCKTAIL PARTY TIDBITS
* Although "quark" is usually pronounced to rhyme with bark, when American physicist Murray Gell-Mann came up with the name he wanted it to rhyme with dork. He says he used the "kwork" sound first without thinking about how to spell it, before coming across the line "three quarks for Muster Mark!" in James Joyce'sUlysses . This sounded apt, but GellMann wanted to keep his original pronunciation.
SOLIDS
THE BASICS
Of the three familiar states of matter, solids have the lowest energy; the atoms or molecules jiggle around less than in a liquid or a gas. In solid form, atoms or molecules link together. Although the solid is primarily empty space, the links between the particles give it a rigidity that distinguishes it from a liquid.
Solids come in several forms. Many are crystals. Here the links between the atoms or molecules form regular patterns, giving a tendency to shear on certain planes. Other solids are disordered. Disordered solids are often more flexible. Glass, for instance, although it can shatter, is a disordered solid, making glass fiber very flexible. There are also solids, often organic, with long chains of molecules linked together, making them particularly strong in one direction.
A single substance can have several solid forms. Carbon, for example, forms crystal planes that slide over each other in soft graphite, extremely hard interlocked crystal structures in diamond, or self-contained molecules shaped like a soccer ball, called buckyballs.
ON THE FRONTIER
We are used to three states of matter — solid, liquid, and gas — but modern physics recognizes five. The most energetic is plasma, of which more later. The least energetic is a Bose-Einstein condensate, dreamed up by Albert Einstein in the 1920s. The Indian physicist Satyendra Bose had devised a way to describe light as if it were a gas. Einstein helped Bose firm up the math, but was also inspired to imagine a state of matter where intense cold or huge pressure forced it to share some of the characteristics of light itself. Such matter is a Bose-Einstein condensate.
COCKTAIL PARTY TIDBITS
* It's sometimes thought that glass is a liquid, because medieval window glass seems to have run down the panes, making them thicker at the bottom, but this merely reflects the way glass was made. Panes were uneven, and it made sense to put the thicker edge at the bottom.
LIQUIDS
THE BASICS
In a solid, the bonds that link atoms or molecules are relatively rigid. But with increased energy (higher temperature) those bonds break. The particles are still attracted to each other, but without a rigid structure. The result is a liquid that can flow and adapt to the shape of a container.
Unlike gases, liquids form surfaces. On these surfaces, almost all the attractive forces between the liquid molecules are inward; this produces a skinlike effect called surface tension. Surface tension is why drops of water form, and why some insects can walk on water. When a liquid rests on a solid, the liquid is attracted to the solid by the same forces, resulting in wetting.
The liquid we are most familiar with, water, is atypical. The positively charged hydrogen in a water molecule is attracted to the negative oxygen in adjacent molecules. (This kind of attraction is called a hydrogen bond.) This bond gives water strange properties, such as expanding as it freezes.
ON THE FRONTIER
Fans of Kurt Vonnegut may have come across the concept of Ice Nine in his novel Cat's Cradle. Vonnegut described a form of ice that melts only at 114 degrees Fahrenheit (45Celsius). If water ever got into an Ice Nine form, under normal weather conditions it would never get out of that form. Should a seed crystal of Ice Nine be dropped into a lake or an ocean it would spread uncontrollably, locking up the water supply and devastating the Earth.
(Continues…)
Excerpted from "Instant Egghead Guide: Physics"
by .
Copyright © 2009 Scientific American.
Excerpted by permission of St. Martin's Press.
All rights reserved. No part of this excerpt may be reproduced or reprinted without permission in writing from the publisher.
Excerpts are provided by Dial-A-Book Inc. solely for the personal use of visitors to this web site.
Table of Contents
CHAPTER ONE MATTER,
CHAPTER TWO QUANTUM THEORY,
CHAPTER THREE LIGHT,
CHAPTER FOUR RELATIVITY,
CHAPTER FIVE FORCES,
CHAPTER SIX ENERGY,
Appendix A: Recommended Reading,
Appendix B: Physics Online,