Huygens: The Man behind the Principle available in Hardcover, Paperback
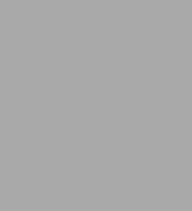
Huygens: The Man behind the Principle
- ISBN-10:
- 0521181437
- ISBN-13:
- 9780521181433
- Pub. Date:
- 02/17/2011
- Publisher:
- Cambridge University Press
- ISBN-10:
- 0521181437
- ISBN-13:
- 9780521181433
- Pub. Date:
- 02/17/2011
- Publisher:
- Cambridge University Press
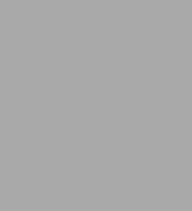
Huygens: The Man behind the Principle
Buy New
$71.99Overview
Product Details
ISBN-13: | 9780521181433 |
---|---|
Publisher: | Cambridge University Press |
Publication date: | 02/17/2011 |
Edition description: | Reissue |
Pages: | 476 |
Product dimensions: | 6.00(w) x 8.90(h) x 1.00(d) |
About the Author
C.D. Andriesse studied physical engineering at the Technical University in Delft and gained his Ph.D. in
1969. His research career moved first into astrophysics at the University of Groningen and then materials research, with a prime interest in nuclear safety and electrical network stability. Since 1989 he has been a professor at the University of Utrecht, researching energy conversions in fuel cells and wind turbines. In addition to his research articles, Professor Andriesse's non-research publications include a handbook on problems with nuclear energy, for the IAEA, essays on the cultural value of technology, diverse historical work and an intellectual autobiography.
Read an Excerpt
Cambridge University Press
0521850908 - Huygens - The Man behind the Principle - by C. D. Andriesse
Excerpt
1 Titan
It was 14 April 1629. The mother was certain that she would give birth to monster a, because just a few days earlier, she had been frightened by the disfigured face of a street urchin. But her son was born without blemish, though extremely heavy. She brought him into the world in the dead of night, and when dawn broke it was Saturday, the day of Saturn the giant, begetter of giants. If he had been born one day later, the father thought, he would have been an Easter child. And in the name of the Father, the Son and the Holy Ghost, he had him baptised Christiaan.1
These are the physical beginnings of our biography of Christiaan Huygens. But if our story is to come to life, we need to know what is so special about the man. If he was truly an exceptional scholar, as many maintain, then he must be worth our exceptional scrutiny. No simple task. What was considered extraordinary three centuries ago may well seem perfectly ordinary to us today. So we must first explore the world of seventeenth-century science.
Christiaan lived in the age wherein (and now we quote):2
Philosophy comes in with a Spring-tide; and the Peripateticks may as well hope to stop the Current of the Tide, or (with Xerxes) to fetter the Ocean, as hinder the overflowing of free Philosophy: Me-thinks, I see how the old Rubbish must be thrown away, and the rotten Buildings be overthrown, and carried away with so powerful an Inundation. These are the days that must lay a Foundation of a more magnificent Philosophy, never to be overthrown: that will Empirically and Sensibly canvass the Phenomena of Nature, deducing the Causes of things from such Originals in Nature, as we observe are producible by Art, and the infallible demonstration of Mechanicks: and certainly, this is the way, and no other, to build a true and permanent Philosophy.
This quotation dates from 1664. The otherwise insignificant writer to whom it is attributed was describing the scientific revolution of the seventeenth century. And in laying the foundations for the science that was both splendid and superior, and 'never to be overthrown', Christiaan Huygens played a key role.
Let us follow the masterly overview that E. J. Dijksterhuis was able to give when all of his works had been published.3 Despite recent arguments that his role was rather peculiar - but what is not peculiar in a fast transition?4 - we think this overview is fitting, and still largely valid.
By the time Christiaan, aged sixteen, went off to study at Leiden University, the scientific revolution was already under way. The professors were well versed in the geometry of ancient Greece, as well as goniometry and symbolic algebra. René Descartes had made improvements in symbolic algebra that would enable it to be used as a simple and powerful tool in mathematics. Together with Blaise Pascal, he demonstrated how this algebra could be applied to geometry, so that the circuitous methods of the Greeks would no longer be necessary. However, because Archimedes was still held in such high esteem, mathematics was mainly grounded in geometry, and was directed at determining curve lengths, surfaces and centres of gravity of flat models with a curved circumference. Scientists were still seeking ways to calculate the tangent to an arbitrary curve, and to estimate the highest and lowest values. Towards the end of the century these labours led to the discovery of the differential calculus. Numerical mathematics evolved quite separately from geometry. At the beginning of the century, Henry Briggs had developed the logarithm, which not only simplified computation but also enabled the progress of pure mathematics. One generation later Pierre de Fermat resuscitated Diophantus' number theory and discovered, in correspondence with Pascal, several fundamentals of the probability theory.
Around 1645, Galileo played the same role in mechanics as Archimedes had done in mathematics. At the beginning of the century he had explained the motion of falling or thrown objects, using precise methods of observation and measurement, and had written a stimulating treatise on the subject. He raised the problem of the origin of motion, which would only be solved at the end of the century. He also demonstrated how principles governing inertia and relativity of motion could be applied. And in connection with these subjects, he defended the heliocentric World System that Johannes Kepler had revealed with his planetary laws. Theologians continued to reject it, but astronomers were inclined to accept this reality, though it would be a long time before they were convinced that it was the Earth that moved. The telescope, an instrument that had evolved from spectacle lenses, led to the discovery of numerous celestial phenomena which supported this heliocentric view of the universe. Kepler provided further insight into the medieval theory on the passage of light through glass (the dioptrics), but refraction, the key to this concept, would only be discovered later, by Willibrord Snel, and represented in Snel's Law. Medieval knowledge of natural history was further enriched by William Gilbert, who studied magnetism, and Evangelista Torricelli, who measured the equilibrium in fluids and gases. Pascal combined these into a single theory, reawakening interest in the molecule theory of ancient Greece, which Pierre Gassend formulated anew.
It was Descartes who invented a revolutionary theory to encompass all these innovations. When Christiaan arrived in Leiden, this theory was just beginning to find support. Descartes believed that all natural phenomena were caused by the collision of invisible tiny particles of matter. Because of their invisibility, the proof of their existence must necessarily be metaphysical, yet he was convinced of his success. All physics could be understood by means of a simple theory of collision and the use of simple mathematics. This theoretical idea held powerful sway because it fitted in so well with Gassend's molecule theory and Cartesian symbolic algebra.
But apart from such theoretical ideas, it was also strongly believed that the study of natural history must develop through reason, inquiry and experiment. Francis Bacon had insisted on this at the beginning of the seventeenth century. But his programme of experiments was not well thought out, and was virtually unworkable. It was only in the second half of the century that his plea for an empirical approach led to a logical and coherent programme, which could be carried out by English and French scientific societies. Instruments would be required, however, such as air pumps and accurate clocks, and these were yet to be developed.
Unlike in our time, in the seventeenth century the study of physics wasn't regarded as something out of the ordinary, but as something familiar - indeed, as a noble task. Descartes expected that his theory would enable people to become 'lord and master of nature', not for the sake of power, but in order 'to enjoy, with neither effort nor exertion, all the fruits of this earth', and to remain in a state of good health, for 'good health provides the basis for all further happiness'. Bacon anticipated that empirical science would enable physicians to lengthen people's lives:
This is her new and most noble task. People will honour doctors, not only because they are necessary, but also because they dispense to man his greatest happiness on earth.
Now that we have set the stage for scientific thought in the seventeenth century, let us consider the role of Christiaan Huygens. He had been sent up to Leiden to read law, but felt drawn towards the study of mathematics. Having familiarised himself with the working methods of the Greeks, he very soon produced solutions that even Archimedes had not thought of. In 1650 he supplemented the classical work on floating bodies with De iis quae liquido supernatant (On Objects which Protrude above the Surface of a Liquid), and in 1654, the classical work on the circle, De circuli magnitudine inventa (On Finding the Circumference of a Circle). He published the second work, but not the first, as if that had been merely a finger exercise.
For Christiaan Huygens, mathematics was a means and not an end, although later on he did devote himself more to mathematics than was strictly necessary. The same applies to his book Van Reckening in Speelen van Geluck (Mathematics in Games of Chance), which consisted of practical rules for playing dice, while at the same time contributing to the theory of probabilities. Similarly, his work on logarithms, intended for the improvement of tuning musical instruments, also deals with their mathematical significance.
He started out, however, with pure mathematics. In 1651 he published a method to calculate the surface of a segment of an ellipse or a hyperbola, thus embarking upon a field now known as integration. He carried on with integrals for several years and finally achieved such interesting results that outstanding mathematicians of the time acknowledged him as their master. Interestingly, they only got to know about these results through his letters, as he published very little. He also revealed very little in his letters as to how he had reached these conclusions.
It was quite normal at the time to be reticent about publication. Some mathematicians actually made their living by keeping their methods secret, such as Gilles Personne de Roberval, who had to sit an exam every three years in order to maintain his professorship. But this wasn't necessary for Christiaan. The reason he didn't publish his findings was because he wasn't easily satisfied with them. Proofs still had to be demonstrated in terms of relationships in geometrical figures according to Archimedes' standard. This proved a handicap for many of the newly emerging problems; it was time-consuming and a waste of talent. The integrals of the parabola, for example, one of his unpublished masterpieces, were also demonstrated by Hendrik van Heuraet, Pierre de Fermat and James Gregory, without any of them being aware either of his work or of each other's. It became a matter of economic necessity to produce these integrals with greater speed.
The process of differentiation that would make this possible had already been set out in 1675. In fact, Christiaan had stumbled upon it when Gottfried Wilhelm Leibniz, who discovered it, had come to him two years earlier to learn mathematics. It took Christiaan twelve years to understand the new method, but even then he found it difficult to apply.
In the field of mathematics, Christiaan was clearly a virtuoso, but not an innovator. The science that he did rejuvenate was physics. In 1652, he wrote the very first physics formula (a mathematical equation for a physical phenomenon). For this alone, he may be regarded as the founder of mathematical physics.
The first phenomena that we encounter in this border area between physics and mathematics are those of equilibrium and motion. Phenomena such as heat and magnetism are more complex and were barely understood in the seventeenth century. It was only logical that Christiaan should turn his attention to the science of mechanics, which had been given new impetus by Galileo with his research into falling and thrown objects. In 1652, thanks to his own mathematical precision, Christiaan discovered the theory of collision, and in 1659, the formula for force. Yet he failed to see the causal connection between motion and force, which made his studies of motion less convincing. Nevertheless, his contribution has been exceptional. In order to understand it fully we have to see it in connection with his practical interest in the pendulum clock.
Galileo had already studied the regular motion of a pendulum. In his later years he had proposed using this to operate a clock, but had not got round to actually making one. Christiaan, who was clever with his hands and technically minded, invented a fork to connect the pendulum to the cogwheel of the clock, allowing it to swing, unimpeded. This discovery ensured the success of the pendulum clock and motivated him to extend his studies in three different directions.
First of all, he tried to adapt this clock for use in shipping, both to measure time and to determine distances at sea. Secondly, he tried to make the oscillation of the pendulum (a bob swinging on the end of a string) independent of deflection by changing the path of the bob from the circular arc. Thirdly, he tried to describe the pendulum more accurately than as just a string with a point mass on the end, meant to represent the bob, because that was incorrect.
His first attempt led to the invention of the clock with a spiral spring, a sort of wound-up pendulum. His second led to the discovery that the path of the bob that guarantees an equal time swing is a cycloid, a curved line that led to his discovery of evolutes. His third attempt yielded a theory to calculate the centre of oscillation of an extended pendulum: the first step down the long road to the dynamics of solid bodies. For this he used Torricelli's proposition that the centre of gravity of a number of interconnected objects, in equilibrium, lies as low as possible. This proposition seemed so obvious to him that he also applied it to single colliding objects. He had already discovered what is now known as the law of conservation of kinetic energy.
Christiaan did eventually publish some of these findings, though he waited a long time and had to be repeatedly exhorted to do so. When Horologium oscillatorium (The Pendulum Clock) finally appeared in 1673, it was regarded as a masterpiece, even by Isaac Newton, whom we shall meet again. But Christiaan's greatest innovation hadn't been included in the work - the theory of centrifugal force and the ingenious deduction of the laws of collision with the aid of a principle of relativity. By locking away this pioneering work in his study, he had far less influence on the development of physics than he might otherwise have done.
As we have seen, Descartes' knowledge of collision theory was the foundation of his revolutionary view of the universe. The philosopher had made these deductions himself and had them published. But right from the start of his own investigations, Christiaan had discovered that the Frenchman's conclusions were wrong. It was not only contrary to the laws of physics, which was forgivable for a philosopher, but also to the laws of logic, which was unforgivable. This was so awkward that Christiaan's professor of Cartesian mathematics asked him not to publish them. He complied. What was even more remarkable: Christiaan was in no way put off Descartes' idea. He simply set himself the task of meticulously rebuilding this edifice, stone by stone.
Christiaan's De coronis et parheliis (On Rings around and near to the Sun) took Descartes' Les Météores (Weather) a step further; his Discours de la cause de la pesanteur (Discussions on the Cause of Gravity) worked out the effect of gravity caused by scattered particles; his Traité de la lumière (Treatise on Light) calculated light to be caused by colliding particles. It was Huygens' collision theory on light that would have the greatest influence on the development of physics, but only a hundred years after its publication. At that time his mathematical understanding of the collision effect proved extremely useful in determining the effect of waves or vibrations. This in turn could be used to explain the interference phenomenon in light. Apart from this, his Traité was highly thought of by his own contemporaries, especially Leibniz.
Just as the pendulum clock constituted the practical side of his theoretical mechanics, the telescope constituted the practical side of his theoretical optics. Together with one of his brothers, he polished lenses and made telescopes. He calculated the image formation of lenses by applying Snel's law of refraction on spherical surfaces. In this way he obtained insight into the magnification of the telescopic image and the distortion of the image known as spherical aberration. With that insight, he was able to design a compound eyepiece with little colour distortion, which became known as the 'Huygens eyepiece' and was frequently copied.
This work, too, remained unpublished. In 1656, however, he published his findings on certain peculiar properties of Saturn, which he had discovered with his first home-made telescope. He reported that this planet had a moon - Titan - and a ring. His discovery of the ring, in particular, was quite unprecedented, and brought Huygens instant fame. Nevertheless, he was not the sort of astronomer to scan the skies night after night, looking for something new, like the fanatical stargazers Johannes Hevelius and Giovanni Cassini had done. He was more interested in putting these celestial phenomena, about which there was so much speculation, into the proper perspective.
In the end, this perspective led to a series of original experiments, which would make him the first to produce a reasonable estimate of the distance between stars. It wasn't until the nineteenth century that this estimate was improved upon. His Cosmotheoros (Contemplation of the Universe), in which he published his findings, is a fascinating cosmological work, remarkable for the stringency of his speculations, its unprecedented popularity in the eighteenth century, and the vilification that followed. Until recent times people have tried to conceal it, for fear that Huygens might be discredited.
In 1687, just as he was embarking upon this later work, Christiaan acquired a copy of Isaac Newton's Principia mathematica. The book made a deep impression on him. But just as he had had difficulty with Leibniz's new mathematics, he also found it hard to grasp Newton's new physics. Newton had solved the problem of motion raised by Galileo with the aid of an inertial force. But he also introduced a gravitational force, which was to work at a distance. Though how, not even Newton could explain. For Christiaan, it was a step backwards into the darkness that he had struggled to overcome. He found it absurd.
That is how our man was depicted in his own time: as an intellectual giant. Yet we search in vain in The Hague for his statue.5 The Hague was the city where he was born and died, but, more important still, it was the city where he made his greatest discoveries. Two attempts have been made to erect a statue in his honour. The first, in 1868, by the Haagsche Maatschappij Diligentia - a society of mathematicians - together with the Royal Society of Sciences in 1868, soon failed, because the two parties were unable to raise enough money among the citizens. The second attempt, in 1905, by the Hollandsche Maatschappij der Wetenschappen, after they had received a legacy of 40 000 guilders for this purpose, actually resulted in a design. The legacy had been left by L. Bleekrode and the design was by the famous Dutch architect P. J. H. Cuypers. It was to be a 20-metre-high monument, rectangular, with a niche on either side for a sculpture of Christiaan Huygens, as well as three depictions of his life. On top of this would be a column surrounded by the signs of the zodiac and crowned with various female figures. The Lange Voorhout, in the heart of The Hague, was suggested as a suitable location. The city council turned it down.
Would we prefer a different image? We have Titan. In June 1655, Christiaan was able to confirm his discovery of a moon in the vicinity of Saturn, for he had observed a starlet travelling four times around the planet. He sent an anagram to astronomers in London and Prague, consisting of a line from Ovid and the letters 'uuuuuuu ccc rr h b q x'. The line was: 'Admovere oculis distantia sidera nostris' ('Faraway stars move towards our eyes'). Those who had an idea as to the meaning of the anagram were able to rearrange these letters to form the sentence: 'Saturno luna sua circumducitur diebus sexdecim horis quator' ('A moon revolves around Saturn in sixteen days and four hours'). This is Titan, the largest moon in our solar system.6 It is the size of a small planet, such as Mercury, it is two-and-a-half times as small and forty times lighter than the Earth, and shrouded in a thick mist of nitrogen and methane, which absorbs most of the light. At a distance of 1.2 million kilometres from Saturn, it completes its orbit in 15 days, 22 hours and 41 minutes. The fact that Titan - together with the ring that he discovered shortly afterwards - is a fitting image for Christiaan Huygens, is borne out by his own words:7
Ingenii vivent monumenta, inscriptaque coelo
Nomina victuri post mea fata canent.
Let them remain as signs of my sagacity, and their names
That I write across the heavens be an echo to my fame.
© Cambridge University Press