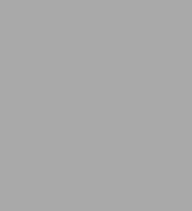
How Loud Can You Burp?: More Extremely Important Questions (and Answers)
288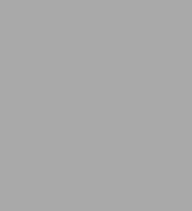
How Loud Can You Burp?: More Extremely Important Questions (and Answers)
288Paperback(Original)
-
PICK UP IN STORECheck Availability at Nearby Stores
Available within 2 business hours
Related collections and offers
Overview
Product Details
ISBN-13: | 9781596435063 |
---|---|
Publisher: | Roaring Brook Press |
Publication date: | 09/01/2009 |
Edition description: | Original |
Pages: | 288 |
Product dimensions: | 5.40(w) x 8.20(h) x 0.90(d) |
Lexile: | NC960L (what's this?) |
Age Range: | 8 - 12 Years |
About the Author
Read an Excerpt
How Loud Can You Burp?
Science of Me
From sunburn to snot, from breathing to burping, few of us ever stop wondering about how our bodies work and what makes us tick. Sure, we study parts of the body at school, and learn all about where our kidneys are, what our lungs do, and stuff like that. But what about goose bumps, hiccups, and hay fever? Why do we have eyelids but not earlids or noselids?
And what is that thing that dangles down the back of your throat called? 1
This chapter is, literally, all about you. And you guys had a lot of questions about yourselves, let me tell ya. Some, of course, were easier to answer than others.
You got a question from: Name: James Question: What would happen if you ate poop?
You'd almost certainly throw up, James. Unless you actually like eating poop, that is. 2
And now, on to the rest ...
How loud can you burp?
The loudest burp on record is around 105 decibelslouder than a motorcycle or chainsaw, and loud enough to cause real pain to anyone close enough to it. But don't try these at homethey could be dangerous!
Louder than a motorcycle?! No way!
Yup. The world-record burp measured 104.9 decibels (decibels, or dB for short, are the units used to measure volume). And that was from over 8 feet away! Close up, the world champion burper claims to be able to reach 118 dB or more. The average motorcycle roars away at around 90 dBa full 28 units lower!
So who did it?
A British guy named Paul Hunn. He smashed the previous burping record in July 2004, and no one has topped it yet.
How could he burp so loud?
Well, like all sounds, burps are just waves of air pressure, and, if you make these waves big enough, any sound can become loud. To create a sound, an objectlike a bell or guitar stringis made to vibrate back and forth very fast by striking it, plucking it, or rubbing something against it. In turn, the object compresses the air molecules around it, making waves or vibrations that are carried through the air. When they reach your ears, these pressure waves vibrate your eardrums. From there, the vibrations are amplified by a set of little bones, picked up by a set of tiny hairs in your cochlea (which is a long, thin tube filled with fluid and lined with hairsall coiled up like a snail shell in your inner ear). Here the vibrations are finally translated into nerve signals that your brain interprets as sounds, such as "bell," "guitar string," or whatever.
But what about burps?
In the case of burps, the vibrating object is a fleshy flap called the cardia, which closes off the stomach from the food tube, or esophagus. When air is swallowed (either by accident while you're eating, or on purpose if you're trying to force a burp), it gets trapped in the stomach. As the stomach fills with food, liquid, and gas, the pressure builds up and the air bursts through the flap, vibrating it on the way out and creating that deep, satisfying BRRRRRRRRRRRRRRPPPPP sound as it goes. Of course, if you want to force the burp out, you can squeeze your stomach by contracting your stomach muscles and diaphragm (which is the flat sheet of muscle underneath your stomach and lungs). This is how Mr. Hunn made his burp so loud. Millions of kids around the world use the same method to force loud burps. He's just much better at it than anyone else. Oh, and he also swallowed lots of soda first.
Yeahwhy does soda make you burp like that?
Soda is made bubbly and fizzy by adding carbon dioxide gas under pressure. So when you drink it, you swallow the gas. The gas builds up in your stomach, annnnd ... you can figure out the rest.
Is it dangerous to make yourself burp like that?
Well, drinking lots of soda isn't very good for you, and swallowing air on purpose won't do your stomach any good, but that's not really what makes burping as loud as Mr. Hunn does dangerous. It's after the burp leaves the body that it becomes a danger to you and others.
But if a burp is just air and sound, how could it be dangerous to anyone?
If they're loud enough and at the right frequency, sounds can be very powerful and dangerous. Ever hear of opera singers who can shatter glass with just their voices? Well, that's true. All they have to do is hit the right pitch, and sing the note loud enough, and the glass will vibrate and shake itself to pieces. And the U.S. military has even developed a "sound weapon" that fires waves of air pressure and sound instead of bullets. The Vortex Ring Gun shoots a ring of vibrating air that can knock down a grown man over 30 feet away.
So if you burped loud enough, could you crack a person's glasses? Or knock a bunch of people over? That'd be sweet!
Err ... no. Not quite. Even the most accomplished burper, like Mr. Hunn, couldn't produce enough air pressure to knock someone down. And his burps are too low-pitched to crack glass. But he could burp loud enough to hurt your ears, or even damage them permanently.
Really?
Yupreally. Mr. Hunn burps at between 105 and 118 dB; 85 dB is enough to temporarily damage your hearing. Builders using jackhammers (which thump away at around 120 dB) wear earplugs or ear muffs to avoid damaging their hearing. If you burped at 165 dB, that would be the same as a gunshot going off right next to your head. So burp this loud and you could deafen yourself and other people!
Yeah, and what a letdown too.
How come?
Just think, you can burp as loud as a gunshot, but after the first time no one can hear it. Not even you.
Err ... yeah ... that'd be a real tragedy.
Just one more thing ...
What's that?
Do brussels sprouts make you burp?
I don't ... think so, no. Why do you ask?
They should. 'Cause they come from Belch'um.
Oh man, that was bad.
Heh, heh. BUUUURRRRRRRRRRRRRPPPPPPPPPP!!!!
Sci-facts: noisy stuff
The volume of a sound wave is related to its air pressure and measured in decibels (dB). On the decibel scale, 0 dB marks the softest sound most people can hear (although some people can hear sounds at -10 dB or lower). Here's how some common (and uncommon) noises measure up:
Why do we get hiccups, and how do you stop them?
Hiccups are funny. We know what they are, we know what they do to our bodies, and, for the most part, we know how to get rid of them. But we can't say for sure where they come from, or what they actually do in the body.
What is a hiccup, anyway?
A hiccupor singultus, as medical scientists call itis a kind of forced intake of breath, caused by muscle spasms in your chest and throat. There are over a hundred causes of hiccups, but the most common is irritation of the stomach or the esophagusthe food tube that leads to the stomach. The "hic" noise comes when the breath is cut off by the snapping shut of your glottis, which is like a fleshy lid or trapdoor that separates the food and air tubes in your throat.
So why are they called hiccups rather than singultuses or something?
Good question. That's because the word hiccup is an example of onomatopoeiaa word that sounds like the thing it describes. So hiccups were named after the noise they make.
They weren't always called hiccups. In sixteenth-century English, they were called hickops. By the seventeenth century, they'd become hiccoughs, and by the eighteenth they were known as hickets or hyckocks. The hic part seems pretty universal, as foreign words for hiccup sound similar. The French get hoquets and the Japanese get hyakkari. The Germans suffer with the much wetter-sounding schluckaufs. Still, it's nice to know that everyone gets them, whatever they're called.
So if everyone gets them, what are they for, exactly?
The truth is, we're not really sure what they're for. According to some scientists, hiccups don't really do anythingthey have no function in the body at all (other than to make us look silly). Instead, they think hiccups might be a kind of malfunction in the nerves that control the breathing muscles and glottis, which happens when the nerves get irritated or damaged.
Then hiccups are useless?
Maybe, but then again maybe not. It could be that they're useless to us now, but they once served a purpose in the animals we evolved from. Another idea is that the hiccup evolved to help our four-legged ancestors to swallow food that got stuck in their throats. Where we humans have the luxury of gravity helping food down, quadrupeds (animals that walk on all fours) have to shift their food horizontally to get it from their mouths to their stomachs. This means it's easier for lumps of food to get stuck in their throats. Some scientists think that lumps lodged like this might press down on a nerve in the throat that triggers the hiccup. Thesharp breath in then creates a vacuum behind the food, and helps the animal suck down the lump. This might explain why dogs (not known for eating their food slowly) seem so prone to hiccups; they "wolf" their food down in big lumps that they have to clear by hiccupping.
OK, but then why don't hiccups stop once you stop eating?
Well, sometimes they do, and sometimes they don't. Most cases of the hiccups are cured (or go away by themselves) within a few minutes. Others can go on for weeks, or even years. In fact, doctors give names to different classes of hiccups, depending on how long they go on. Common hiccups are gone within an hour. Persistent hiccups can go on for up to forty-eight hours but are usually harmless (although very annoying!). Acute hiccups go on for more than forty-eight hours and are usually caused by drugs, but they can also occur naturally. For example, in January 2007, a teenager from Florida named Jennifer Mee hiccupped for five weeks straight, for no known reason! If hiccups go on for longer than two months, they're classed as intractable or diabolic hiccups, and they're usually the sign of a serious illness.
Two months? That sounds like a nightmare! Is that the longest they can go on for?
Well, the world record stands at 68 years, with a guy named Charles Osbourne (from the United States) who hiccupped continuously from 1922 to 1990. The poor guy basically had hiccups for life.
OKI have to know. How do you get rid of hiccups? I heard that if you stand on your head and drink a glass of water ... no, waityou have to eat a raw chili pepper, right?
For common hiccups, there are literally hundreds of recommended "cures" out there. Some involve eating or drinking things, others tell you to hold your breath. Some tell you to drink a glass of water in a certain way, others to get a friend to distract you. In reality, the ones that actually work (and many don't) do so by helping you get c your breathing. So it doesn't really matter what you eat, or how you drink the water; it's just the interruption of your breathing pattern that does the trick. Holding your breath usually works best, since it's the most direct way of controlling your breathing muscles.
What about getting a friend to scare you?
That usually only works if the hiccups are psychosomaticwhen you're setting off the jerky contraction in the breathing muscles yourself, but you're not aware that you're doing itkind of like imagining each hiccup into existence. It's not always easy to tell when this is happening, but getting someone to distract you can snap you out of it long enough for them to stop. Of course, none of these remedies is likely to work on acute or intractable hiccups. Since they're usually startedby drugs or nerve damage, they're often only treatable with more drugs. That said, some researchers have claimed success treating hiccups with needles, massage, and radiation.
Needles and radiation? Yikes! I think I'd rather have hiccups!
Me, too! Or perhaps I'd give one of those crazy cures a try instead ... .
Top 10 weird hiccup cures (that may or may not work)
Why do we breathe, and why do we need lungs to do it?
We breathe to capture oxygen, which helps us convert food into energy in our cells, and to get rid of carbon dioxidethe waste gas that is formed as this happens. Our lungs pump these gases in and out of our bodies, and help us dissolve them in and out of our blood.
So let me get this straightwe breathe so that our cells can eat food?
Not exactly, but close. It's more like "we breathe so that our cells can breathe, and eat so that our cells can eat."
What? They breathe too? Now I'm really confused ... .
OK, let's back up. If you think about it, cells are like little units of life. The smallest living things, bacteria, are just individual cells swimming around eating stuff. They absorb sugars or other nutrients from the air or liquid around them and turn this "food" into energy, which they can use to grow, multiply, and squidge around looking for more food.
Got it.
OK. Now some of these bacteria eventually evolved into bigger creatures like fish, frogs, lizards, monkeys, and human beings. Those particular types of bacteria are called aerobic bacteria, which means ...
... that they wear tracksuits and exercise a lot?
Err ... no. It means they have to "breathe" (or take in) oxygen in order to turn their food into energy.
Oh, yeah, of course. I knew that. I mean, nobody makes tracksuits that small, for starters.
Err ... right. Anyway, these bacteria and the animal cells they evolved into all need to take in oxygen for that same reasonto fuel their food processors and produce usable energy.
But why do they need oxygen to do that? Couldn't they do it without oxygen?
Well, they can for a while. But the main power source for aerobic bacteria and cells comes from a chain reaction that uses oxygen, so they can't survive without it for long. Oxygen and nutrients go into this reaction, then energy and carbon dioxide come out. The energy is stored and moved around in special molecules, while the carbon dioxide has to be removed from the cell, since it forms an acid if too much of it builds up. So, in a way, aerobic bacteria "breathe in" oxygen and "breathe out" carbon dioxide. These bacteria evolved into complex animals by clustering together and eventually developing into the cells, tissues, organs, and systems that make whole animalbodies. But, since each cell still needs nutrients and oxygen for energy, the whole animal has to keep eating and breathing just to supply them.
OK, that kind of makes sense. But why do bigger animals need lungs to breathe?
Well, if you think about it, the bigger an animal gets, the more cells it has, and the further away from the air its insides are. Up to a certain size, animals can absorb oxygen through their skin and let it spread through their tissues. But most things bigger than flatworms need air tubes of some kind to get air to the inner parts of the body (that works for insects and spiders, at least). For larger, more complex or more active animals, you need some kind of gas-exchanging air pump. That's where lungs come in.
But not all animals have lungs, right? I mean, fish don't, do they?
Rightthey don't. Fish have gills instead. They swallow water and use their gills to absorb the oxygen dissolved in it. Then thegills dump carbon dioxide back into the water before pushing it out. That's what's happening when you see the gills "flapping" on a fish.
So why can't we just swallow oxygen and burp out the carbon dioxide?
Well, then you'd be swallowing continuously, every few seconds, all day long. Like a big, walking air-fish. Not much fun, and kind of tricky to hold conversations. Plus it wouldn't work anyway, since swallowed air goes to your stomach, which is already specialized for digesting food and is too small for exchanging breathing gases.
Why does size matter?
You need a large surface area for the gas exchange to happen quickly enough between the air you take in and your bloodstream, which carries it to cells throughout the body. That's where lungs come in handy. They're more than just air sacks; they're like giant air sponges that soak up and exchange gases very quickly through thousands of tiny pompoms, called alveoli.
You're telling me I have pasta in my lungs?
No. That's ravioli.
Oh. I knew that.
Alveoli are the clustered, berrylike pompoms found at the end of the branching air tubes deep in your lungs. They help increase the surface area of your lungs so much that if you flattened them all out into one sheet, it'd cover about 250 square feetroughly the size of a tennis court. When you breathe in, muscles around and beneath the lungs help them suck air into the alveoli. These are covered in tiny blood vessels so that oxygen can move into the bloodstream and be carried around the body. Carbon dioxide gas moves the other way: The bloodstream carries it out of organs and tissues and back to the air inside the alveoli, ready to be pumped out again as you breathe out. And there you have it. You and all your cells have all the oxygen they need, provided you keep breathing air and avoid damaging all your delicate breathing equipment with dangerous fumes or cigarette smoke.
Got it. Don't breathe cigarette smokeor pastaand your cells can keep doing aerobics.
Something like that.
Sci-Facts: lungs and stuff
* The animal with the largest lungs on Earth is the blue whale, which may also be the largest animal ever to have lived. At up to 98 feet long and weighing 148 tons, the blue whale is even larger than the biggest dinosaurs ever found. A blue whale's lungs hold up to 530 gallons of air, compared with about 1 gallon for the average human.
* Elephant seals and sperm whales can hold a single breath for up to two hours! The human world record stands at 17 minutes and 4 seconds. achieved by illusionist and "endurance artist" David Blaine in April 2008.
The lungs are the largest organs in the human body (unless you count the skin, which can be thought of as an organ or a tissue). The average male lungs weigh about 35 ounces, while the average weight for female lungs is 33 ounces. This doesn't explain, however, why boys are usually louder ... .
Why do we have eyelids but not earlids?
Because eyelids helped usor rather our animal ancestorsto survive, whereas earlids would've made little difference to whether they survived or not.
How could eyelids help us survive? I mean, what makes them so important?
Well, what makes any part of your body important? What about your hands? What good are they?
That's easy. We can use them to pick things up and make things. Use tools and stuff.
OK, and how would that have helped our early human ancestors survive?
They could build shelters. And catch food. And make fires for cooking it.
Exactly. But how many of those things could they have done easily without being able to see?
Err ... I dunno. I guess they could have just about done them. But it would've been much harder. But we're talking about eyelids, not eyes. You can still see without eyelids, can't you?
Yes, you can. But, if you're a land animal, then not for long.
Why not?
Think about it, what are eyelids really for?
Stopping stuff from getting in your eyes?
Like what?
Dust. Muck. Shampoo.
Right, but none of those things alone is particularly dangerous. It's the bacteria that hitchhike on them (wellon the dust and muck, not the shampoo) that are the problem. Once they get into your eyes, they can cause an infection, whichbefore the days of antiseptics and antibioticscould leave a person or animal blind. Eyelids form part of a system (along with the glands and ducts that make tears) evolved by land animals that not only shields the eyes but also keeps them moist and sweeps them cleara little like windshield washers and wipers on a car. Tears even contain special proteins called lysozymes that destroy bacteria by busting them open. Without this eyeballwashing system, most animals would go blind within days, as their eyes would quickly dry out and become infected.
So, then, do all animals have eyelids?
Not all of them. Most mammals, birds, reptiles, and amphibians do, but fish do not since they don't really need to keep their eyes moist underwater. Animals that evolved to live on land but then later returned to the water (like seals and otters) generally do have eyelids, but some whales do not. They've long since lost them, since they were no longer needed to ward off airborne bacteria. Dolphins, though, do still have eyelids. Maybe because they swim much faster, they need eyelids to help protect their eyes against debris whipping by them in the water.
OK, so eyelids are pretty important. But wouldn't earlids be useful too?
Possibly. But not so useful that without them earlidless animals would die out, leaving mostly earlidded animals behind. In other words, for earlids to have evolved, they would have had to help mutant earlidded animals survive or reproduce more effectively than those without them. That's how evolution works.
And there aren't any animals with earlids? At all?
Not earlids, exactly, but some animals have closable ears. Desmans (which are kind of like aquatic moles) don't have external ears, but they can close the tiny holes leading to their internal ears when diving underwater. Hippos and sea lions also close their ears underwater. They have external ears that they can rotate and fold flat to their heads to keep the water out. They can also close their nostrils, which is a bit like having noselids, I suppose.
Cool! So could we ever evolve earlids and noselids in the future?
It's possible, I guess. But remember, nothing evolves on purpose. Evolution works by picking out mutations. Mutations happen by chance all the time, but most either have no effect on the life-form or are bad for it. Useful mutations are quite rare. But it's these that allow organisms to evolve. If a mutation proves useful enough, it'll be passed on to more of the mutant's offspring, and over time it becomes a new feature of the species (since most or all animals of that species will end up with the mutation). So for us to evolve earlids or noselids, there would have to be (a) mutant people with earlids and noselids (or at least the beginnings of them); and (b) some sort of pressure in our environment that gradually killed off people without earlids and noselids, or prevented them from having kids. And it would have to happen over a long period of time, as evolution usually works very slowlyover hundreds, thousands, or millions of years, rather than days or months.
What if global warming made all the oceans flood the land, and we had to live in boats and feed only on sea animals? And we had no fishing rods. So we had to dive for shellfish and stuff all the time. Would that do it?
Well, being able to close your ears and nose might help equalize pressure while you're diving, I guess, but ...
Excellent. What about flippers and fishtails? And gills so we can breathe underwater?
I'll leave you to figure that out, I think.
Hmmm ... so first the air gets all poisonous so we can't breathe. No, waitthe surface of the sea freezes over so we can't come up for air, and ...
Why can't you smell your own farts when other people can?
Because they're more sensitive to your particular brand of bottom burp than you are. Or you're just refusing to own up to it.
So some people are better fart-sniffers than others?
Well, yesin a way, I suppose. But it'd be fairer (and more polite) to say that some people are better sniffers than others, generallynot just of farts, but of all kinds of different smells.
How come?
Because people vary in the smell detectors they're born with.
You mean some people have bigger noses than others?
Actually, how big the nose is doesn't have much to do with it. It's more to do with how many special odor-detecting sensors they've got up their nose, and how finely tuned they are to different smells. These special proteins, called chemoreceptors, send signals to the brain when they detect the presence of certain chemicals in the air. Each receptor recognizes the shape of just one type of chemical molecule. When that molecule enters the nose and binds to the correct receptor, it triggers a nerve signal that whizzes to a special region of the brain called the olfactory region (olfaction is the scientific name for smelling, so olfactory just means "used for smelling"). Here, signals from lots of different receptors are decoded, and your brain interprets the result as "grass," "chocolate," "fart," or whatever. How well you can detect smells, and distinguish between similar smells, depends on how many of each different type of receptor you have. This can differ quite a bit between two people, which makes some people far more sensitive to smells (and better able to tell the difference between "your fart" and "my fart") than others.
So if you can't smell your own fart, it means you have a worse sense of smell than people around you?
Not necessarily. Other things can affect your ability to interpret smells too. Catching a cold, for example, produces mucus that clogs the inner passages of the nose and stops the odorcarrying air from reaching the chemoreceptors so easily. This is why you can't smell anything much with a stuffy nose. But even if you have a clear nose and a better sense of smell thanthose around you, you still might not notice your own "trouser coughs" before others do.
Why not? I mean, the fart begins at your bottom, right?
Right.
And it spreads out from there?
Correct. The smelly fart gas released from your bottom gradually spreads outward (or diffuses) to fill the room, reaching closer noses first and more distant noses last. (Unless someone comes to the rescue by opening a window, that is.)
So if your nose is closest to your own bottom, how could you not get the first whiff?
Because even though the fart reaches your nose first, you might not recognize it. Or, more accurately, your nose might recognize it, but decide to ignore it.
Why would it do that?
Because of the way your nose, and other sense organs in the body, are designed. Your sensesincluding sight, hearing, smell, taste, and touchall evolved to detect changes to your surroundings or environment, rather than things that stay the same. So if you constantly or repeatedly experience the same sensationlike a continuous sound, smell, or touchthen your senses can sometimes "tune out" this sensation as "background noise." That's why when you first put a ring on your finger, you can feel its weight and pressure. But after a while this constant sensation is tuned out by your brain,and you can't feel it (or rather don't take any notice of it) anymore. The same thing happens with sounds and smells. You might hear the continuous hum of the new fridge in your kitchen for an hour or so, but after a while you don't hear it at all unless you're trying to. And although the smell of a bad egg or stink bomb seems to quickly go away on its own, it's often still therebut your nose is ignoring it.
... so if you're always dropping your own "bad eggs," then after a while you might not smell them so easily?
Right. If you've been gently farting away all day, then unless this latest "eggy rumble" is notably stinkier or otherwise different from the last, your nose might happily ignore it as part of your ever present "background" smell ... while your friends get the full nasal assault.
Of course, there is a simpler explanation of why you can't smell it and others can.
What's that?
You're lying.
What? No way! Err ... I mean, how would that work?
Quite simple. You've farted, and it's slightly stinky. But apparently nobody's heard it, and you're hoping they haven't smelled it either. Even if they do, they have no proof it was you, so you can blame someone or something else (see list of useful scapegoats here).
As if. I would never do that.
Of course not.
Practical science: things to blame a fart on
If it's noisy
A creaky floorboard A passing motorcycle A neighbor playing the saxophone
If it's stinky
The compost heap in the garden Brussels sprouts boiling in the kitchen Eggs rotting in the garbage
If it's noisy and stinky
The dog Someone else Anyone else
If it's not noisy or stinky
What's the problem? If a fart is neither heard nor smelled, is it really a fart at all? Think about it ... .
Why does pollen give you hay fever?
Because some people's bodies are paranoid and overreact to it. They treat harmless pollen like a dangerous alien invader and launch a full counterattack that clogs up their eyes and noses.
But why do only some people get it?
Because not everybody (or rather, not everybody's body!) reacts to pollen this way. Only people who have become extra sensitive (or hypersensitive) to inhaled pollen get the reaction that causes the symptoms of hay fever. They literally become allergic to pollen.
And how does that happen? Are they just born like that?
For some sufferers, yes. Scientists have recently found a gene that may be involved in hay fever and other allergic reactions. If you're born with a certain version of this gene, you may be much more likely to develop hay fever when you grow up. This particular gene helps manage your body's immune system, which controls your ability to fight off the bacteria and viruses that cause diseases.
So hay fever is caused by a virus?
No, it isn't. But, in a way, people who have hay fever treat pollen as if it is one.
When you inhale a nasty microbea virus or bacterium that could cause diseaseyour body's self-defense system (or immune system) responds to it in several ways. First, immune cells stick to a few of the microbes, chew them up, and leave bits of them (called antigens) sticking out for other cells to examine. These antigens are often pieces of outer shell or coat from the bacterium or virus.
Next, more immune cells encounter these bits, clone themselves, and make thousands of proteins called antibodies. The antibodies recognize the antigens (that's where the name comes fromthey're anti-body generators) and help launch a massive counterattack that destroys all remaining microbes of the same type.
Finally, some of the antibodiesand the cells that create themstick around to ward off future attacks. If the same microbe is inhaled again, these cells and antibodies can launch an even quicker response, and deal with it way before it causes any symptoms of disease. This is why the first time you're infected by something like chickenpox or measles, it causes the nasty symptoms of disease (like spots on the skin, swelling, and fever). After that, you're immune, and you don't even notice your body comfortably fighting off further attacks.
But what does that have to do with hay fever?
In hay fever sufferers, it's thought that their immune systems respond to pollen as if it was a nasty microbe because some immune cells in their noses are made hypersensitive to it. Instead of ignoring pollen in the nose as an irritating (but harmless) bit of muck, these cells launch an over-the-top counterattack. They release a substance called histamine, which helps bring immune cells (as reinforcements) to the area from the bloodstream. Histamine makes the blood vessels swell and leak so that the immune cells can squeeze through the walls to reach the "deadly" pollen particles. Unfortunately, this also leaves the eyes and nose all red, itchy, watery, and clogged with white goo.
Of course, this explains how hay fever happens once you have hypersensitive immune cells. But it doesn't explain how your cells got so in the first place. And that one faulty gene alone can't explain all the hay fever sufferers out there.
How come?
For the last hundred years, the number of hay fever sufferers seems to have been increasing hugely in many places around the world, and that one gene probably can't account for them all. There are just too many of them. Since people (especially children) in cities and towns get hay fever far more than people in the countryside, some scientists thought at first that it might be caused by pollution. But pollution alone probably can't explain all the new cases either.
Why not?
Among other things, it wasn't people living in the most polluted areas that developed hay fever fastest. In many towns and cities, hay fever seemed to crop up most among families with fewer childrenoften those who lived in richer, cleaner areas away from busy roads and factories.
That got scientists thinking that the reason for this sensitivity to pollen might be something else.
Like what?
One theory is that hay fever develops when you encounter too much pollen at once, after never having been exposed to pollen before. So, while people in the countryside breathe in a lot of pollen, they're exposed to it slowly and gradually, and their immune systems become used to it and ignore it. In the cities, some children never encounter pollen in the air until the height of the tree pollination season, when the wind blows a big cloud of it in from the surrounding countryside. Thisshocks the immune cells into thinking it's an all-out microbe assault, making them hypersensitive to pollen from then on.
So you get hay fever because you're suddenly hit with loads of pollen all at once?
Yup. Could be. This "all at once" theory seems to explain a few other mysteries too. Such as why there was little or no hay fever in Japan before 1950, but now most Japanese children and adults suffer from it. This was because millions of cedar trees were planted in Japan after World War II to create timber for building and selling, which exposed millions of Japanese people to huge amounts of cedar pollen for the first time. Since then, the number of cedar trees has stayed the same, but the number of cars on Japanese roads has gone up enormously. So it could be a combination of "pollen shock" and pollution that has given so many Japanese people hay fever. Add the faulty gene to that mix, and this probably explains the overall increase in hay fever worldwide.
Can you stop it once you've got it?
If you have hay fever already, there are lots of good medicines that work by blocking the action of histamine and helping to stop the eyes and nose from watering and itching. If you don't have hay fever, and you live in a big town or city, it might be a good idea to escape the pollution and build up your "pollen immunity" by getting out to parks and the countryside as much as you can. Even if it doesn't keep you from getting hay fever, it'll be healthier than staying indoors or hangingout near roadsfor any number of other reasons. Plus you can learn more outdoors than you can indoors, which will make you a wonderfully intelligent and interesting person. If you don't believe meconsider this:
Stuff you can become an expert on indoors
PlayStation, Xbox, Wii, music, whatever you can find on TV or the Internet
Stuff you can become an expert on outdoors
Football, tennis, volleyball, soccer, baseball, hockey, lacrosse, climbing, hiking, running, cycling, spelunking, skiing, surfing, skating, boarding, kung fu, karate, Tai Chi, scuba diving, swimming, diving, flora, fauna, music (with an iPod)
Why do we get goose bumps when we get cold or scared?
It's a reaction left over from our evolutionary past. Our animal ancestors were much hairier than us, and goose bumps helped them (a) stay warmer, and (b) look bigger and scarier.
What do goose bumps have to do with being hairy?
Everything! Goose bumps are quite literally a hair-raising reaction. The scientific name for a goose bump is a piloerection, which just means "hair standing up" in Latin. And that's exactly what happens. Each hair on the body has a set of tiny muscles beneath it. When stimulated, these tighten up, creating a dent right under the base of the hair and a raised ring of skin around it. This makes the hair stand up straight. Depending on how hairy you are, you might not have an actual hair follicle on that spot any more (or, if you do, it might be too small or too fair to see). But the muscles are still there under the skin, so when stimulated they will still contract, making a bump (or, more accurately, a raised cratera bit like a tiny skin volcano).
Why are they called goose bumps?
Because on hairless skin a whole set of these bumps look a bit like the knobby skin of a goose (or any other bird, for that matter) after it has been plucked for cooking. Eating geese isn't so common anymore, but the name comes from a time when it was, and has stuck around.
So you could rename them chicken bumps or ostrich bumps?
If it made you feel better, yes. Although "chicken bumps" sounds a bit too much like chicken pox, which is something else entirely, and "ostrich bumps" makes them sound like especially large, super-sized goose bumps ... .
Maybe you get them when you're really cold, or really scared. Yeah, ostrich bumps ...
OK ... if you say so. Call 'em what you like.
But how does having your hairs stick up help you stay warm? Wouldn't that let more cold air onto your skin?
Well, keeping warm is less about "keeping the cold out" and more about "keeping the heat in." When you feel cold, it's actually because you're losing heat from your skin to the colder air around you. If you have a lot of body hair (like our early humanlike ancestors and the apelike animals before them), then raising a whole carpet of hairs traps a layer of warm air next to your skin. This slows down the rate of heat loss from the skin's surface, keeping you warmer. Of course, most humans aren't nearly hairy enough for this to have much ofan effect now, so the hair-raising reaction is pretty useless. Instead, we wear clothes next to our skin, and the clothing fibers help trap warm air and slow heat loss instead.
So, if we'd never started wearing clothes, we'd still be mega-hairy all over?
Well, not mega-hairy, but hairier maybe. Our ancestors didn't start making or wearing clothes until they'd lost much of their body hair already. It was probably making fires and building shelters to keep warm that reduced the need for a thick coat of all-over body hair in early humans. Plus there are many places in the world (such as parts of the tropics, and some regions near the equator) where it stays fairly warm all the time. People from those regions tend to wear fewer clothes and have far less body hair than those from, say, northern Europe or Asia. So we may have gradually lost our body hair even without clothes.
OK, so for a while goose bumps helped us to keep warm ... but why do you get them at other times, like when you're scared or excited?
Again, we haven't got enough hair now for this to make any difference, but for our hairy animal ancestors the hair-raising reaction made them look bigger and scarier. If you've ever seen a startled cat, you'll have seen this in action. When surprised or alarmed by another animal, a cat arches its back, stretches up to stand as tall as it can, and its fur stands on end. This makes the cat look quite a bit bigger than it really is, making the other animal think twice about attacking it. Lots of hairyanimals use a similar tactic, including apes such as gorillas and chimpanzees, which are not too different from the animals we evolved from. Now, of course, this reaction is no use to us at all. But that doesn't stop goose bumps from happening when we're scared. Even if we're just watching a creepy movie.
But sometimes you get them when you're not scared at all, just excited or nervous. What's up with that?
That's because the hair-raising reaction can happen in two different ways. When you're cold, your nervous system senses the temperature and sends signals to each hair follicle, telling the muscles beneath them to contract as an automatic reflex.But when you're scared, excited, or emotional, something else happens. A hormone (or chemical signal carried through the bloodstream) called adrenalin is released from a pair of glands that sit on top of your kidneys. This affects your body in a number of ways that prepares it to do battle or run away (it's sometimes called a "fight or flight" reaction, for that reason). This includes speeding up your heart rate, increasing blood flow to your muscles, and triggering the hair-raising muscles in an attempt to make you look big and scary.
Cool! I've got to try this out. I'm off to startle the cat.
Well, if you want to see your own reaction, you'll need someone to startle you.
What do you m
BOO!!
Yaaaaggghhhhh!!
Heh, heh.
That wasn't funny. I nearlyoh, waitgoose bumps!
Told ya.
Why do spicy things taste hot, even when they're cold?
Because the family of protein sensors that detect hot and cold things on your skin and tongue can also be triggered by chemicals found in spicy things.
Hold on, you've lost me. What's a protein sensor?
It's a clever biological molecule that changes shape when it's activated by a specific chemical or by a temperature change. This starts a chain reaction that sends a nerve signal to your brain, telling it the chemical (or temperature change) has been detected. So it acts like a chemical or temperature sensor.
How does protein do all that? I thought protein was just something you ate to help you grow and make you strong and brainy.
Well, it is. But did you ever think about why? Or, for that matter, why you need to eat food at all?
For energy?
That's part of it. But it's also to build structures and biological machinery within your body. Different foods are needed for different purposes, and you need a good balance of all of them to stay healthy. The three major types of food molecule are carbohydrates, fats, and proteins. Carbohydrates is the name given to sugars and starches. (Starches are found in "starchy" foods like potatoes and pasta.) Carbohydrates are mostly used as an energy sourcepowering your brain, muscles, and pretty much every type of tissue in your bodybut they also form structural frameworks within and between cells. Fats are used to build walls (or membranes) around cells in your body, for insulation to keep you warm, and as cushioning around your organs to keep them safe. Fats are also used as a slow-burning energy source, kept in reserve and used when your blood sugar dips too low. So carbohydrates and fats are very important as building materials and fuels, but they're fairly simple and can't do much beyond that. Proteins, however, come in an incredible variety of different shapes and sizes, folding themselves into highly complex structures to perform a wide range of functions. It's proteins that do all the really interesting stuff in the body.
Like what?
Some build things by joining other molecules together. Others destroy things by breaking them apart. Hemoglobin proteins carry oxygen from the lungs to the rest of your body. Antibody proteins attack bacteria and viruses, surrounding them or punching holes in them. Transcription proteins read your DNA,and translation proteins decode itdirecting the construction of every last cell in your body and controlling every function that takes place in them. And sensory proteins detect changes both inside and outside your body, relaying all kinds of information to your brain. This includes information about body temperature, blood pressure, the contents of your blood, the contents of the air around you, light, sound, color, smells, tastes, and more. 3
Whoa! So they're pretty clever little things, huh?
I'd say so! Plus some protein families have evolved to do more than one type of job. Like the sensory proteins inside the nerve endings under your skin. One family of these, called "the TRP channels," is used to sense extreme temperature changes on your skinlike when freezing ice or boiling water comes into contact with it. But they're also used for vision, smell, and other purposes.
Like for tasting spicy things?
Well, they didn't really evolve for thatit just so happens that they're triggered, accidentally, by some spicy things. Or, rather, they react to chemicals (or compounds) found in the leaves and seeds of certain plants. Each type of TRP-channel protein senses a different range of temperatures. One called TRP-A1 detects temperatures from 50°-59°F, which is cold enough to cause pain. Another, called TRP-M8, senses the 68°-77°F range, and yet another (TRP-V1) senses temperaturesbetween 104° and 113°F. But these proteins also react to plant compounds, sending the same signal to your brain as if they had been heated up or cooled down. So the plant things make the proteins send false "hot" and "cold" signals to the brain.
The capsaicin compound in chilies triggers the TRP-V1 protein, simulating heat, while the menthol compound in mint triggers the TRP-M8 protein, simulating cold. The effect is worse in sensitive areas with lots of nerve endings (and lots of TRP channels), like on the lips and tongue and around the eyes. That's why chilies burn your tongue, while mint makes it feel cold. Other plant compounds found in garlic, horseradish, and mustard affect TRP proteins too, causing "false hot" or "false cold" effects in a similar way.
But why would plants make chemicals that make our lips burn or our tongues freeze? Are they trying to hurt us?
Not hurt us, but keep us from eating them, maybe. It could be that some plants evolved to produce these "spicy" compounds to keep small mammals from eating them, leaving them to be scarfed by birds instead. Birds don't seem sensitive to spicy foods (perhaps because they have a different set of TRP proteins), so spicy plants may be using a sort of chemical weapon that fights off mammals but doesn't deter birds.
But why would a plant want a bird to eat it instead of a mammal?
Because some plants spread their seeds by getting animals to eat them, carry them around in their stomachs, and eventuallyahemplant them ... .
You mean, poop them out?
Yes, thank you ... some distance away. And since birds fly further than most mammals can walk or scurry, a plant can spread itself over a wider distance via bird than it would via mammal. Spicy plants couldn't have done this on purpose, of course. But once a few of them had started producing mammalrepelling "spicy" compounds, those plants spread further (and so survived better) than those without them. Later the "nonspicy" ones died off, leaving only the "spicy" plants behind. And bingo!a new spicy species had evolved.
So why do we eat spices if they're supposed to put us off?
Partly because in small amounts, we can enjoy the tingly "hot" or "cold" sensations spices bring to our food, and partly because we get less sensitive to them the more we eat them. If we eat enough, the TRP channels stop firing altogether. When this happens, the TRP sensor proteins not only stop reacting to the spice, but they also stop reacting to temperature, pressure, or pain. That's why people who eat spicy food a lot can generally handle the hottest spices. And it's also why your mouth goes numb after eating a very spicy curry, or after using very minty toothpaste or mouthwash. The numbing sensation is so strong, in fact, that chili, garlic, and mint extracts are used in some traditional or natural medicines as painkillers.
You mean rubbing chili or mint into a sore spot to make it go numb? Yowch! No, thanks!
Hey, don't knock it till you've tried it. I used mint chocolate chip ice cream for a stubbed toe yesterday, and that worked.
You mean you put your toe in a tub of ice cream?!
No, I ate it all. But I do feel better ... .
Top 10 spicy peppers
The spiciness of peppers is measured using "heat" units called Scoville units. Here's how some common peppers measure up.
Why does the sun darken your skin but lighten your hair?
Because sunlight does different things to both. It damages skin cells, so your skin darkens to protect itself, but it also stops new hairs from darkening as they grow.
Sunlight damages your skin? What are we, vampires or something? I thought getting out in the sunshine was supposed to be good for you ... .
It is. But, like most things, too much of it can be bad for you too.
But we need sunlight to live, don't we?
That's true, we do. You, I, and pretty much every living thing on the planet depend on the Sun's rays for energy. The Sun warms the Earth, its atmosphere, and its oceans, keeping the whole thing warm enough for animals, plants, fungi, and microorganisms to survive. Plants and bacteria use sunlight to grow, forming an energy source for all the other living things that feed on them. And even things that live their entire lives cut off from sunlightin deep ocean trenches, or deep undergroundfeed on other things that couldn't exist without thewarmth and energy provided by the Sun. In humans, sunlight is particularly important, as it helps us make vitamin D in our skin, helps us keep our sleep cycles4 regular, and even keeps us happyby triggering the release of "happy chemicals" called endorphins in our brains.
There you go. It's good stuff. We need it.
Yup, we sure do. But we also need food, water, and oxygenand those can be bad for you too. In fact, too much of any one of them could kill you.
What? No way!
It's true. Too much food can make you obese, which causes heart disease, diabetes, and other problems. Too much water can kill you through hyperhydration, which is the no-less-deadly opposite of dehydration. And too much oxygen can kill you through oxygen toxicityas, believe it or not, high concentrations of oxygen can damage your cells. (And, if you're wondering why this doesn't happen all the time, it's because the air you breathe is only 21% oxygenmost of the rest of it being nitrogenso this isn't usually a problem.) Just because something is good for you in small doses doesn't mean more of it is even better for you. And, for many of us, that's how it is with sunlight.
So what does sunlight do to your skin?
That depends on your skin type, how long it's exposed to thesun, and whether or not you've used sunscreen. Unless you're lucky enough to have naturally dark skin (and even dark skin can be damaged if exposed long enough), then the ultraviolet radiation within sunlight penetrates your skin cells and damages the DNA inside them.
Yikes!
Thankfully, though, your cells contain a kind of biological DNArepair machine made out of a group of proteins, which usually notices and fixes the damage soon after it's done.5
Phew!
But if your skin-cell DNA sustains too much damage too quicklythrough too much exposure to sunlightthen your repair proteins can't keep up. So your skin cells react by darkening themselves, which they do by producing more of a dark pigment protein called melanin. This partly shields the cells, helping to prevent further damage to the DNA, and buying a little time for the existing damage to repair itself.
So suntans are good, then. They stop your skin from getting damaged by the sun, right?
Unfortunately, no. A suntan is evidence of skin damage, not healthy skin. A light tan that gradually fades is fine. But, unless you're naturally dark-skinned, a constant, dark tan (the kind people sometimes try to keep up using tanning beds) is a sign of constant, ongoing skin damage. If you get sunburned on the way to tanning, then at worst you could eventually developskin cancer. At best, keeping an unnaturally dark tan will make your skin age more quicklymaking it drier, less stretchy, and more wrinkly.
What about your hair? Is too much sun bad for that too?
Not really, since sunlight has a different effect on hair than it does on skin, although it also involves the dark melanin protein that gives you a suntan.
But wouldn't that make your hair go darker in the sun? That's not what happens at all.
True. Over time, some (but not all) types of hair seem to get lightened or bleached by the sun. But when this happens, the hair actually starts out light, and just fails to get darker as it grows.
Huh?
Well, as you probably know, hair grows from the roots, rather than the ends. It's made of long threads of a protein called keratin, which are made by hair cells in your scalp (and elsewhere). These threads are then bundled together into fibers, which start out a bit like soft, transparent rope. Melaninthe "darkening" proteinis added to these soft fibers at the root of each hair follicle, and the newly grown hair is then pushed out into the sunshine. When sunlight hits the hair, the keratin hardens, and you're left with a dark hair (or at least a nonwhite onewithout the melanin, that's what color it would be).
So what difference does more sunshine make?
More or stronger sunlight causes the keratin to harden more quickly, which stops the melanin from binding to it so easily. Hence, more sun means less melanin in each hair, and the less melanin you have, the more blond the hair becomes. This is also why sun-bleached hair darkens again once you're back to "regular" amounts of sunlightthe soft fibers growing from the root start binding melanin once more, and the blond hairs "grow out." (This is also why dark hair bleached with chemicals quickly becomes dark at the roots toounless you keep on bleaching the roots, the hair there keeps growing the way it always did, ignoring the lighter hair above.)
So sunlight isn't bad for your hair, it just changes its color for a while?
Right. Although you can still get sunburned on your scalp through hair, which is why wearing a hat is a good idea if you're out in the sun all day.
OK, so if some sun is good for you, but too much is bad for you ... what are you supposed to do?
Get out in it, but be prepared. If you have fair skin, you can cover up and use sunscreen to prevent getting burned. But if you spend all day covered up, you're not getting the benefits of the sun on your skin either (like making vitamin D in your skin). So the best way is to get a little sun on your skin, but cover up or duck into the shade way before you start to burn. Just ten minutes in direct sunlight is enough to boost your vitamin D levels. How long it takes for you to burn depends onyour own skin type, and how sunny it is where you are. My pale, freckly skin starts to burn after about thirty minutes in the summer sun. If I'm dumb enough to lie in it for an hour, I look like a lobster. For you, it may be different.
So "catching a few rays" = good, "lobster tan" = bad.
Exactly. Just wish I'd learned that a bit earlier. Yowch!
Top 10 worst places to get sunburned
(from pasty Glenn's own experience)
How can two parents without red hair have a red-headed baby?
Because hair-color genes are passed down from parents to children in different combinations, giving a range of possible colors, including red.
What do genes have to do with it?
Genes have something to do with pretty much everything that happens in our bodies. Some features, like hair and eye color, are controlled almost completely by genes. Other things, like height, weight, and even intelligence, are partly controlled by genes, and partly by what you do with your body (like how much you eat, exercise, and learn) as you grow and develop.
But why? What makes genes so important, and how do they work?
Well, that's kind of a long story, but here's the short version. Well, short-ish ...
Every cell in your body has a part in the middle that contains DNA. This is the stuff that genes are made of. The DNA is arranged into forty-six little packages called chromosomes. Each one contains thousands of genes, which together formthe blueprint for how to build and maintain your body. So the chromosomes are like a big set of do-it-yourself books, and each gene is like one page in one book, telling you how to build just one tiny part. Only the pages are written in code, and the instructions have to be decoded or translated before they can be used.
So the instructions tell you how to build, whatan arm, or a leg, or a kidney, or something?
Not quite. It all starts a little smaller than that. The instructions actually describe how to build proteins, which are biological molecules that do all the really interesting stuff in your body. So, once all the decoding and building are done, you end up with a massive variety of different proteins.6
OK ...
Now everyone has the same basic set of DIY books (or chromosomes), but no two people have exactly the same instructions written on every page. So, compared to someone else's set, you might be missing an entire page here and there, which means you'll be missing a specific gene, and a specific type of protein. Or the page might have been copied slightly differently, altering the instructions and leaving you with a slightly different gene (and a slightly different protein) than someone else. When this happens, you can end up with blue eyes instead of green eyes, or brown hair instead of blond hair.
So the feature (or trait) that the protein controlslike hair or eye colorcan differ. And the trait you end up with depends on which genes are passed down to you from your parents' DIY manuals. Got it?
Think so. But if you were a kid with red hair, and neither of your parents had red hair ... then ... like ... who gave you the red-hair gene?
They both did.
Huh? I don't get that at all.
That's because I left a couple of things out of the story. For starters, not all of the forty-six books in the set are different. In fact, it's more like you have two sets of the same twenty-three-volume series. One set of twenty-three you got from your mother, and the other set of twenty-three you got from your father. So now you have two copies of every book and every page. In other words, you have two copies of each gene, and these copies might be slightly different from each other. Let's call them the "mom" version and the "dad" version.
OK. Now what?
Now these two versions of the same gene can interact with each other to produce the hair-color trait. And, depending on which versions you inherit, they might work together or work against each other. So your "mom" version and "dad" version of the gene might cooperate to decide your final hair color, or one might overrule the other and decide on its own.
Sounds like Mom and Dad all right.
They don't actually decide, of course. Genes are just molecules, and they don't think at all. In reality, the genes and the proteins they build interact in complex ways, producing or blocking chemical reactions within the cell. But the outcome is the same. Genes either cooperate with each other, or one takes over, or dominates, the other.
Yup. Still sounds like my parents.
Anyway, here's how it all comes together. The protein that controls whether or not you end up with red hair is called the melanocortin receptor (or MCR) protein, and the instructions for building it are within a gene called MCR1. Got that?
Yup. So far, so good.
Now this protein acts on other proteinschanging their shapes and giving them different functions. In particular, it turns a "hair-reddening" protein (called pheomelanin) into a "hair-darkening" protein (called eumelanin). But remember that you have two versions of each gene.
Mom version, dad version, right?
Right. Now if both versions of your MCR1 gene contain the same, common instructions, then you won't get red hair. This is because you'll make plenty of the MCR protein, which in turn converts the "reddening" stuff into "darkening" stuffdarkening your hair overall. We call these common versions "dominant" and they trump "recessive" versions of the gene,which have altered instructionsa bit like having spelling mistakes in them. So even if your "dad" version of the gene is the common (dominant) one, and your "mom" version has a mistake in it (recessive), you'll still have the correct instructions on board to make the protein that does the darkening jobso no red hair. The "dad" version takes over and makes up for the mistakes in the "mom" version. Got it?
Yeahthink so. Although it's usually my dad who makes the mistakes, and my mom who takes over ...
Gotcha. But, anyway, you get the idea.
But what if both "mom" and "dad" versions have mistakes?
Good question. If that happens, then you have two "recessive" versions, and no dominant versions of the gene to take up the slack. In that case, the MCR protein doesn't get made properly. Without that, you end up with loads of pheomelanin (hair-reddening) protein and no eumelanin (hair-darkening) protein. The un-reddening job isn't done, and you end up with red hair.
So even if your parents have blond and brown hair ...
... then there's still a chance you'll end up with red hair. It just depends which "mom" and "dad" versions of the MCR1 gene you inherit. In short, if both your parents give you the recessive versions of the gene, you get red hair. With one brown-haired and one blond-haired parent, there would be roughly a one in four chance of this happening to each child. Other combinationsof genes would give you blond or brown hair, but not red. That's the basic pattern, anyway. But it can get a bit more complicated. Want me to explain how?
No, thanks! That'll do. Lots of genes, lots of different possibilities. Got it. So do genes control other stuff the same way? Like how tall or how smart you are?
Kind of, only those traits involve even more genes, so it gets a lot more complicated, and there's a wider range of different results (like different heights or IQs) at the end of it all. Plus, as I said at the beginning, these traits also depend on other things, like your diet and learning experiences, because these affect how well the different proteins work and interact.
So if I want to be taller and smarter, could I change my genes to do it?
Not right now, since we don't know enough about which genes are involved, or how they interact. Plus it's nearly impossible to change one gene in every one of the billions of cells in your body once you're half-grown already. And although that might be possible someday, there's a much easier way to get the same result.
What's that?
Eat well, study hard, and make the most of the genes you already have.
But I got mine from my brother, and they're all torn and ripped already.
Err ... that's your jeans, not your genes.
Oh. I knew that.
Practical science: the difference between genes and jeans
Text copyright © 2007 by Glenn Murphy