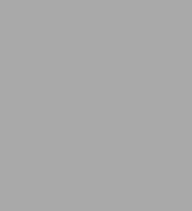
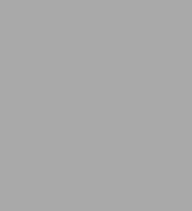
eBook
Available on Compatible NOOK devices, the free NOOK App and in My Digital Library.
Related collections and offers
Overview
Addressing a variety of theoretical cosmological problems, and emphasizing a mathematical approach, this volume nicely complements Peebles' Physical Cosmology (Princeton Series in Physics, 1971).
Ryan and Shepley have concentrated on the structure of models of the universe. By using a modern terminology that emphasizes the operator nature of vectors and tensors, as opposed to their components in a particular coordinate system, the authors develop modern tensor analysis to the point where it can be applied to general relativistic cosmology. They then use it to describe homogeneous cosmologies in considerable detail. Both students and researchers are likely to find these techniques especially useful.
Among their subjects are: spaces with groups of motions; singularities; Taub-NUT-Misner space; Bianchitype models; Hamiltonian cosmology; and perturbations in anisotropic models. A brief section on observations is also included, as is a complete bibliography. A final section presents graded exercises that underscore the potential yet unrealized in this area of study.
Originally published in 1975.
The Princeton Legacy Library uses the latest print-on-demand technology to again make available previously out-of-print books from the distinguished backlist of Princeton University Press. These editions preserve the original texts of these important books while presenting them in durable paperback and hardcover editions. The goal of the Princeton Legacy Library is to vastly increase access to the rich scholarly heritage found in the thousands of books published by Princeton University Press since its founding in 1905.
Product Details
ISBN-13: | 9781400868568 |
---|---|
Publisher: | Princeton University Press |
Publication date: | 03/08/2015 |
Series: | Princeton Series in Physics , #1814 |
Sold by: | Barnes & Noble |
Format: | eBook |
Pages: | 338 |
File size: | 13 MB |
Note: | This product may take a few minutes to download. |
Read an Excerpt
Homogeneous Relativistic Cosmologies
By Michael P. Ryan Jr., Lawrence C. Shepley
PRINCETON UNIVERSITY PRESS
Copyright © 1975 Princeton University PressAll rights reserved.
ISBN: 978-0-691-08146-5
CHAPTER 1
COSMOLOGY: THE STUDY OF UNIVERSES
Who that well his watke beginneth,
The rather a good ende he winneth
– JOHN GOWER
It is difficult to explain to a layman the fact that the universe expands. How, he asks, can the universe expand? Into what is it expanding? We tell him that he does not have the correct picture in mind. Indeed the universe can "expand" in spite of the fact that it is everything and does not in any sense develop into unoccupied space. Instead, we tell him that distances between astronomical objects are becoming larger and larger as time moves forward.
Our layman will then turn to the title of this chapter and ask: If our universe is everything, what need is there to study other universes? And again the answer is very straightforward. We must simplify the study of cosmology; we must attempt to model the real universe in a mathematically tractable structure. In cosmology we study aspects of the real universe and possible aspects of the real universe. It is in fact often instructive to study features which we know not to be present in the real universe. No better example occurs than the study of anisotropic cosmological models, for so far as we can tell the physical universe is completely isotropic. However, an anisotropic model gives an extremely important example of the type of structure which may dominate the very early stages of cosmic expansion.
Our concern is not with the full range of physical and astrophysical cosmology, but rather with the geometrical and mathematical principles of general relativity as applied to cosmology. We shall treat expansion; we shall treat singularities; in short we shall discuss the arena of astrophysics and the boundary of that arena. Our study is limited to the simplest structure – homogeneous cosmologies – for the simplicity evoked by the homogeneity symmetry nonetheless allows very complex models.
The Problem of Fall
The most serious problem of modern theoretical cosmology is the existence of the initial singularity or "big bang." In all cosmological models this singularity appears. It is a region of infinitely dense matter, of infinitely strong gravitational forces. It is the beginning of spacetime, the boundary where our theories of space and time must be false. In the early days of gravitational physics, a problem of similar moment was the problem of fall (Koyre, 1955). The close analogy between the problem of singularity and the problem of fall is instructive.
Galileo established laws of inertia and some properties of gravitation. The question then arose: What is the path of a body falling under the influence of gravity? This question was not trivial, for the detailed law of gravitation had not yet been formulated. Several complicating features arose to slow the solution to the problem of fall: The mathematics (geometry) was not up to the problem; physicists found the mathematical reasoning difficult to apply. The new laws of physics were not well understood; the best mathematicians often had wrong ideas of the concepts involved. Finally, the center of attraction, the earth, is rotating; this rotation complicated the investigations of many researchers.
In the early seventeenth century, before the mathematical and physical ideas of Newton had appeared, the problem of fall was not solved. To introduce this problem in modern terms, imagine that an observer is stationed in the gravitational field of a point particle and throws out a small projectile. The path this projectile will follow can be discovered through step by step calculation making use of the fact that the projectile is always accelerated toward the central attractive point. A compact formula for the path was not available in the seventeenth century, but one special case is easily solved. A requirement of symmetry greatly simplifies the calculation. The initial velocity of the projectile is directly toward the central massive point, this being a naturally symmetric direction. The result is obvious: The projectile will hit the massive center in a finite time. (See Figure 1.1.)
The problem that remained unsolved before Newton was the fate of any other projectile. Will it spiral in to hit the central point? Is there a built-in end-point to the problem of orbit tracing? In this case no planet could have a stable orbit unless non-gravitational forces operated.
The central problem of modern cosmology bears a striking resemblance to the Renaissance problem of fall. Just as one particularly "symmetric" projectile path ends in disaster, so cosmological models chosen by the strongest symmetry requirements exhibit singular beginning points.
The cosmological models dealt with in this book, for example, are required to be symmetric under a three-dimensional group of isometries so that computation of their properties will be simple. Unlike the special projectile path that ended in disaster, however, these cosmological models are very general. Many properties, such as matter rotation, can be arbitrarily chosen (up to seven adjustable parameters). In spite of this generality, every cosmological model evolves a singularity of some sort.
The problem of fall was solved by recognizing that only very special initial conditions lead to destruction of the orbiting particle. This method of escaping trouble does not work in gravitational collapse of stars. Penrose, Hawking, Geroch and others have shown that whatever shape or state of motion a star is in when it starts to collapse, it will unavoidably reach a singular state. In cosmology, also, the relaxation of symmetry does not prevent the singularity. In cosmology this singularity appears as the beginning of the universe, although in some models a second singularity appears as the universe recollapses on itself. It is the beginning singularity that is disturbing, for it is an effect without a cause.
The Beginnings of Modem Cosmology
The problem of fall was an important chapter in the history of gravitation. At that time, too, appeared the very first applications of gravitation to cosmology. To understand the principles, techniques, and problems of modern cosmology it is worthwhile to look at selected incidents over the past several hundred years which have culminated in the theory of general relativistic cosmology.
Whether a static, matter-filled universe is reconcilable with Newton's theory of gravity was the subject of perhaps the earliest correspondence which could be termed modern cosmology. In four letters to Richard Bentley, Newton explored the possibility that matter might be spread evenly throughout an infinite space. It was Bentley's suggestion that this even distribution might be stable, but Newton felt the matter would tend to coagulate into large massive bodies. However, he apparently also thought that these massive bodies themselves could be stably spread throughout all space. Modern Newtonian cosmology, which is remarkably similar to relativistic cosmology, shows that Newton was wrong about this stability. The many other discussions of cosmology in the time of Newton were more of a theological or descriptive nature and do not have what modern researchers feel is the correct outlook: the explanation of cosmic features by use of terrestrial physical laws.
This situation, that the laws of physics known on earth were not applied to cosmological problems, continued with one notable exception. This exception was the effect commonly known as Olbers' Paradox (Jaki, 1969). Olbers' Paradox is the problem of why the night sky is dark. About a century before Olbers both Halley and Cheseaux realized that although the light from a star diminishes as the square of the distance to the star, the number of stars in a spherical shell increases as the square of the radius of the shell. The accumulated effect of the light intensity sould make the night sky as bright as the surface of the sun. In all fairness to Olbers the general scientific community was not able to appreciate fully the work of Halley and Che'seaux because of an insufficient sense of cosmic infinity. The problem is an especially important one, however, in that it depends critically on whether the universe is finite in space, finite in time, or static. The modern resolution of the paradox is that the universe is expanding and that the matter in the universe has a finite age. In particular, it is not a good enough explanation merely to postulate finite space sections in the universe.
The history of contemporary cosmology really began with Einstein's application of the principles of general relativity. Curiously Newtonian gravitation was not employed in cosmological theory until some time after relativistic cosmology was founded. Seeliger (1895) and Neumann (1896) had prepared the way for a cosmological constant in the 1890's, however.
Einstein's cosmology is an example of a slight lack of confidence in general relativity. So strong was his belief that the universe was static that he introduced a cosmological term to modify his original theory. Had he anticipated the work of Friedmann and shown that general relativity required an expanding universe, most researchers of the period would have found his theory unacceptable. Einstein himself at first refused to believe the results of Friedmann and only reluctantly accepted the idea that a non-static cosmological model is possible.
When Hubble completed his redshift survey, the results were astounding. They showed that there is a systematic redshift of light from distant galaxies, increasing as the distance to these galaxies. There have been many attempts to explain this redshift, some of which are quite ingenious. But the most widely accepted explanation, the explanation which now fits several other pieces of data, is that the universe is expanding. When most people accepted the notion of a non-static universe, an enormous number of cosmological models, both relativistic and non-relativistic, appeared. The best expression of the spherically symmetric relativistic cosmological models was presented by Robertson (1929), the mathematical niceties being a product both of Robertson (1935, 1936) and Walker (1936). We will call these models FRW universes to acknowledge the pioneering work of Friedmann. More general cosmologies, including models which result from the application of Newtonian gravitation, were presented by Milne (1934) and Milne and McCrea (1934). It is interesting to note that static cosmological models are not possible in Newtonian theory without the addition of a term much like the cosmological constant of Einstein.
Relativistic cosmologies and Newtonian cosmologies share an important feature. The expansion typically begins with a bang: There is a finite time in the past of any given observer where the density is infinite. This infinite density epoch represents the most fundamental problem of contemporary cosmology. The description of a singularity, whether of infinite density or of another type, and the proof in general of its existence, has only been carried through in recent years. Penrose, Hawking, Geroch, Misner – these are the names of the people who, with many others, have shown that singularities are a common feature of cosmological models. At present the detailed physical interpretation of these singularities remains an unresolved problem.
Although general relativity cosmology was the first cosmological theory, others have come and gone, sometimes without adequate reason for giving them up. Milne's theory, Hoyle's steady state theory, and others have at times been actively investigated but at present are no longer of interest. In the case of the steady state theory there is good, but by no means conclusive evidence that the theory does not meet observation. In particular, the discovery of the 3 K black body background radio emission is accepted by most people as evidence that the universe was significantly different in the past, in violation of the principle of the steady state universe.
Nowhere in this volume do we mention the cosmological principle except here. This principle is a fancy name for a simplifying set of assumptions. Its application results in homogeneous cosmologies, and to some, the principle also implies either a steady state situation or a static model. We prefer to leave the terminology of "principle" to the past, where it was a guide and a solace to researchers. Where we make symmetry assumptions, we state them as mere assumptions to aid in the solution of equations. We do not accept a cosmological principle as a Procrustean law, but leave to the observer the question of whether the universe has chosen to obey any of these assumptions.
Outline
We treat in this book a general consortium of cosmic problems. As a map to our treatment, we have drawn up a flow chart printed on the end papers of this volume. The flow starts with the general mathematical and physical foundations of general relativity and proceeds to relativistic hydrodynamics. The mathematics proceeds to the detailed theory of symmetry and singularity. Detailed applications to homogeneous cosmologies follow. We end with Hamiltonian techniques and with some remarks on astrophysical studies. Our conclusion is "A Call to Arms," for we feel that there are many interesting cosmological problems to be solved.
CHAPTER 2GEOMETRY IN THE LANGUAGE OF FORMS
So if a man's wit be wandering,
let him study the mathematics
– FRANCIS BACON
2.1. Points, Manifolds, and Geometrical Objects
Nothing is so vital to general relativity as the physical reality of an "event," or point, in spacetime, completely separate from coordinate systems used to describe it. On the surface of the earth Moscow is Moscow no matter what latitude or longitude we assign to it. Modern mathematics recognizes this separateness in the concept of a manifold, the set of points on which is placed the geometry of spacetime.
In general relativity the manifold is spacetime. A point of the manifold is identified with a physical event. A sample event is shown in Figure 2.1. As a point in a manifold it is independent of any coordinate system.
This identification of an event with a mathematical point was a daring step due to Newton. Recently, the alternate concept has developed of a sponge-like construct which gives the appearance of smoothness only down to the scale of quantum fluctuations [MATHEMATICAL EXPRESSION NOT REPRODUCIBLE IN ASCII]. At that scale the smoothness vanishes (Weyl, 1949; Wheeler, 1962b; Penrose, 1966). We shall not adopt this alternate viewpoint. Instead we will stay with the classical view of spacetime as a continuous and differentiable manifold.
On this manifold we shall place "geometrical objects" (Veblen and Whitehead, 1932; Schouten, 1954), the simplest of which are function, vector field, metric, and differential form. We shall develop these concepts in this chapter to the extent needed for the analysis of homogeneous cosmologies (see the flow diagram in Figure 2.2) in a form independent of coordinate systems. Definitions will be short and many proofs will be omitted (see standard works on differential geometry, e.g., Helgason, 1962, or Hicks, 1965). We shall end the chapter with a description of the three-sphere, a manifold we shall meet later on in the Friedmann-Robertson-Walker (FRW) universes. We shall also give a short table of useful formulas which have been developed in the chapter.
Definition of Manifold – Topology and Differentiability
A manifold is a set of points, basic subsets of which are labeled open sets. The open sets obey the property that any union of open sets is open (the set 0 < x < 1 is an open set in the real line). Some of the subsets of the manifold will be closed (the complement of an open set; example 0 ≤ x ≤ 1) and some neither open nor closed (0 < x ≤ 1). A set such as a manifold upon which open and closed subsets are defined is a topological space. An open set containing the point P is a neighborhood of P.
(Continues...)
Excerpted from Homogeneous Relativistic Cosmologies by Michael P. Ryan Jr., Lawrence C. Shepley. Copyright © 1975 Princeton University Press. Excerpted by permission of PRINCETON UNIVERSITY PRESS.
All rights reserved. No part of this excerpt may be reproduced or reprinted without permission in writing from the publisher.
Excerpts are provided by Dial-A-Book Inc. solely for the personal use of visitors to this web site.
Table of Contents
- Frontmatter, pg. i
- Preface, pg. vii
- Table of Contents, pg. ix
- 1. Cosmology: The Study of Universes, pg. 1
- 2. Geometry In the Language of Forms, pg. 11
- 3. Spacetime and Fluid Flow, pg. 40
- 4. Friedmann-Robertson-Walker Models: Begin with a Bang, pg. 57
- 5. Singularities in a Spacetime, pg. 74
- 6. Isometries of Space and Spacetime, pg. 96
- 7. Universes Homogeneous in Space and Time, pg. 118
- 8. T-Nut-M Space - Open to Closed to Open, pg. 132
- 9. The General Spatially Homogeneous Model in the Synchronous System, pg. 147
- 10. Singularities in Spatially Homogeneous Models, pg. 163
- 11. Hamiltonian Cosmology, pg. 182
- 12. Type I Models and Type IX Models - The Simplest and the Most Interesting, pg. 201
- 13. Numerical Techniques, pg. 221
- 14. Astrophysical Studies in Anisotropic Type I Models, pg. 237
- 15. Final Remarks: What Is, What Is Not, and What Should Be, pg. 260
- Exercises and Problems, pg. 273
- Bibliography, pg. 288
- Index, pg. 316