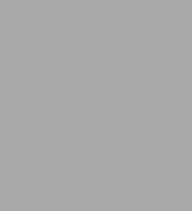
Functional Group Chemistry
171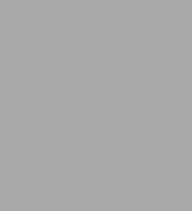
Functional Group Chemistry
171Paperback
-
PICK UP IN STORECheck Availability at Nearby Stores
Available within 2 business hours
Related collections and offers
Overview
About the Author
Professor Abel is an Emeritus Professor at the University of Exeter.
Read an Excerpt
Functional Group Chemistry
By James R. Hanson
John Wiley & Sons
ISBN: 0-471-22480-4Chapter One
General PrinciplesAims The aims of the first chapter of this book are to provide the foundations for functional group chemistry. By the end of this chapter you should be able to understand:
The relationship between bonding and structure of organic compounds
The oxidative and substitutive relationship between functional groups
The relationship between electronegativity differences and the reactivity of functional groups
The reactivity of nucleophilic, electrophilic and radical reagents
The role of acids and bases in the catalysis of organic reactions
The influence of electronic and steric factors on reactivity
The kinetic and thermodynamic control of reaction products
1.1 The Structure of Functional Groups
A functional group is a chemically reactive group of atoms within a molecule. Each functional group has its characteristic reactivity, which may be modified by its position within the molecule or by the presence of other neighbouring functional groups.
1.1.1 Hybridization and Bonding
An isolated carbon atom possesses two electrons in its 1s orbital, two electrons in its 2s orbital and two electrons in its 2p orbitals. The types of bonding found in carbon compounds arise from various hybrids of the 2s and 2p orbitals. Combination of one 2s and three 2p orbitals leads to four equivalent [sp.sup.3] hybridized orbitals directedtowards the apices of a tetrahedron (see 1.1). Each of these orbitals can be occupied by one of the four available electrons from the carbon atom. These can each pair with one electron from another atom to produce, for example, methane (1.2). The energy required for the reorganization of the orbitals is gained from the formation of the four covalent bonds.
Alternatively, the 2s and two 2p orbitals may be hybridized to give a planar [sp.sup.2] system accommodating three electrons from the carbon, one in each hybrid orbital. Three bonds may then be formed with other atoms (see 1.3). The remaining electron, which is in a p orbital at 90° to the plane of the [sp.sup.2] system, may overlap with a comparable p orbital from a second atom to form a [pi]-bond, leading to a double bond between the carbon and this atom as in ethene (1.4).
A further way of making four bonds from the carbon is to hybridize the 2s and one 2p orbital to give two sp hybrids in which the orbitals are at 180° to each other. The remaining two 2p orbitals are used to form two [pi]-bonds at 90° each other (see 1.5). In this case there is a triple bond between the carbon and another atom as, for example, in ethyne (acetylene, 1.6).
These hybridizations have several consequences. Since an s orbital is closer to the nucleus than the corresponding p orbital, the increasing s character in the orbitals in changing from [sp.sup.3] to [sp.sup.2] and then sp leads to a decrease in bond length: [sp.sup.3] C-C, 0.154 nm; [sp.sup.2] C=C, 0.134 nm; sp C=C, 0.120 nm. Secondly, the increasing s character means that the bonding electrons in the sp and [sp.sup.2] orbitals are held more tightly to the carbon than in an sp3 bond. This is reflected in the increase in the polarity and acidity of a [C.sup.-]-H+ bond and in the ease of formation of organometallic compounds containing the [C.sup.-]-[M.sup.+] bond. These follow the order [sp.sup.3] < [sp.sup.2] < sp. On the other hand, there is an increase in the difficulty of breaking a carbon-halogen bond in the ionic sense [C.sup.+]-[X.sup.-], in changing from an alkyl to an alkenyl (vinyl) halide. Thirdly, whereas the maximum electron density of a [sigma]-bond lies between the atoms forming the bond, that of a [pi]-bond lies above and below the plane of the bonding atoms, i.e. a [pi]-bond is more exposed for reaction.
A further consequence lies in the opportunity for one [pi]-bond to interact with another suitably oriented [pi]-bond to give a conjugated system. When a carbon-carbon double bond is separated from another carbon multiple bond by one carbon-carbon single bond so that overlap is possible between the [pi]-bonds, they are said to be conjugated. Thus in butadiene (1.7) molecular orbitals may be written embracing all four atoms. These conjugated double bonds often behave as one functional group rather than as two isolated double bonds. Electronic effects are relayed through the conjugated system.
A cyclic conjugated system containing (4n + 2)[pi] electrons has an extra stability over that of a comparable number of isolated double bonds. This extra stabilization, known as aromaticity, leads to a characteristic pattern of reactivity which distinguishes the reactions of benzene (1.8) from, for example, the linear hexatriene (1.9) or cyclooctatetraene (1.10) (4n electrons, n = 2). The aromatic sextet may arise not just from the overlap of three double bonds as in benzene (1.8) or pyridine (1.11) but also from the participation of the lone pair of electrons on a heteroatom. Thus pyrrole (1.12), with effectively six [pi]-electrons, shows some aromatic character. In allene (1.13) the double bonds are at 90° to each other and conjugation does not occur.
1.1.2 Bonding and Structure
The tetrahedral arrangement of the bonds of an [sp.sup.3] hybridized carbon atom, the planar trigonal [sp.sup.2] arrangement and the linear sp system each have structural and geometrical consequences. The existence of free rotation about a single bond means that in a molecule such as ethane the methyl groups are free to take up a range of different conformations relative to each other. There are two extreme conformations, one in which the hydrogen atoms are staggered (see 1.14) and the other in which they are eclipsed (see 1.15). In the former the interactions between the hydrogen atoms of the methyl group are minimized, and the structure is of a lower energy than that in which the hydrogen atoms are eclipsed. When these ideas are extended to butane, there are three rotamers about the central C-C bond which need to be considered. Not only are there the extremes of the staggered (1.16) and eclipsed conformations (1.17), in which the methyl group interactions are at a minimum and a maximum respectively, but there is also a gauche conformation (1.18) which is intermediate between these.
When the carbon chain is constrained in a cyclohexane ring, there are two extreme conformations known as the boat (1.19) and chair (1.20) forms. The former is destabilized by eclipsed interactions whilst in the latter the interactions are gauche. This conformation is more stable.
The C-H bonds on the chair cyclohexane ring are of two types. One set of six C-H bonds are parallel to the axis of the ring and are known as axial bonds (see 1.21). The other set of six bonds point out of the horizontal plane of the ring and are known as the equatorial bonds (see 1.22). When these C-H bonds are replaced by substituents, the substituents experience different interactions depending on their conformation. These steric relationships play an important role in the influence of the carbon skeleton of a particular compound on the reactivity of its functional groups.
In an alkene such as ethene, the presence of the [pi]-bond prevents rotation about the C=C bond. The hydrogen atoms on the separate carbons are either cis or trans to each other. When the alkene bears substituents on the separate carbon atoms, these are cis or trans to each other. Distinct geometric isomers are possible. These compounds have different properties. Thus cis-ethenedicarboxylic acid is maleic acid (1.23). The carboxyl groups are close together in space and react together to form a cyclic anhydride (1.24). On the other hand, trans-ethenedicarboxylic acid is fumaric acid (1.25) and no such interaction is possible.
1.1.3 The Inter-relationship of Functional Groups
Functional groups may be regarded in a systematic, formal sense to be related by a series of redox and substitutive transformations. Replacement of a hydrogen atom on the carbon atom at the end of the four-carbon chain of butane (1.26) by a hydroxyl (OH) group gives the primary alcohol butan-1-ol (1.27), and when one of the methylene (C[H.sub.2]) hydrogen atoms of butane (1.26) is replaced we obtain the secondary alcohol butan-2-ol (1.28). Replacement of the central hydrogen of the [C.sub.4] isomer 2-methylpropane (1.29) by an OH group gives 2-methylpropan-2-ol (1.30), a tertiary alcohol. In each of these isomeric alcohols there is a hydroxyl (OH) group conferring similar properties. However, the alcohols differ in the number of hydrogen atoms attached to the carbon atom and hence in the properties associated with these atoms. Insertion of the oxygen between the two central carbon atoms gives a further [C.sub.4][H.sub.10]O isomer, ethoxyethane (diethyl ether, 1.31), lacking the characteristic OH of the alcohol and thus containing a different functional group, the ether group.
Oxidation of butan-1-ol gives butanal (1.32) which is characterized by a C=O, a carbonyl group, in this case an aldehyde group. Oxidation of the secondary alcohol butan-2-ol (1.28) gives butan-2-one (1.33), a ketone. There are many common properties of aldehydes and ketones, and others that differ because of the aldehydic C-H.
Oxidation of butanal leads to a carboxylic acid, butanoic acid (1.34). The distinctive properties of a carboxylic acid [C(=O)OH] can be considered as combining those of a carbonyl group modified by an attached hydroxyl group and those of a hydroxyl group modified by an attached carbonyl group. Replacement of the hydrogen atom of a carboxylic acid by an alkyl group gives an ester, for example ethyl acetate (ethyl ethanoate, 1.35).
The functional group which contains two alkoxy groups attached to the same carbon atom occurs, for example, in dimethoxymethane (1.36), and is known as an acetal. A compound containing three alkoxy groups (see 1.37) is an ortho ester.
If another atom such as a halogen, a sulfur or a nitrogen is substituted in place of the hydroxyl group, further functional groups are generated (see Box 1.1).
The alkyl halides (halogenoalkanes), thiols and amines are at the same oxidation level as the alcohols, while acyl halides, thioacids and amides are similarly related to the carboxylic acids. Like oxygen, sulfur can be inserted into a chain to generate the equivalent of an ether such as the thioether.
The tervalency of nitrogen does not permit simple insertion; another group such as hydrogen or an alkyl group must be added to nitrogen, producing for example [(C[H.sub.3]).sub.2]NH (dimethylamine, a secondary amine) or [(C[H.sub.3]).sub.3]N (trimethylamine, a tertiary amine). Note that the terms primary, secondary and tertiary are used in different ways when referring to alcohols and amines.
Tertiary amines can form stable quaternary ammonium salts ([R.sub.4][N.sup.+][X.sup.-]), while thioethers form sulfonium salts ([R.sub.3][S.sup.+][X.sup.-]), but stable oxonium salts ([R.sub.3][O.sup.+][X.sup.-]) are less common.
Dehydrogenation of alkanes such as ethane (1.38) relates them to alkenes such as ethene (ethylene, 1.39). The same functional group may be obtained by dehydration of ethanol (1.40). Further dehydrogenation of ethene would generate an alkyne, ethyne (acetylene, 1.41). In terms of oxidation level, the alkene is related to the alcohol and the alkyne is related to the ketone.
1.1.4 Electronegativity
Having considered the oxidative and substitutive relationships between functional groups, we now consider the factors that contribute to their reactivity. The electronegativity of an element is a measure of the power of an element to attract electrons to itself in a chemical bond. It increases across a period in the Periodic Table from lithium to fluorine and decreases down a group. Electronegativity differences between atoms lead to an unequal sharing of the bonding electrons between the atoms concerned and consequently to regions of electron deficiency and electron excess in a molecule. Some electronegativities on the Pauling scale (lithium = 1 and fluorine = 4) are given in Table 1.1.
The halogens, oxygen and nitrogen are more electronegative than carbon and hence the alkyl halides, carbonyl and imine groups may be represented as in 1.42-1.44, where the full-headed arrows represent the shift of an electron pair.
However, this is not a complete picture of the factors that contribute to the reactivity of functional groups. For example, the electronegativity difference between carbon and iodine is relatively small. In the much larger iodine atom the bonding orbitals are further from the nucleus than in chlorine and are more polarizable during the course of a reaction. These differences affect both the [sigma]- and [pi]-bonds. Thus many of the reactions of the alkyl halides and of carbonyl compounds may be rationalized in terms of the polarization of the bonds and the polarizability of the component atoms.
1.1.5 The Role of Lone Pairs
The non-bonding 'lone pairs' of electrons, particularly on oxygen and nitrogen, are far from inert and play an important role in directing the outcome of many reactions. They may accept a proton or a Lewis acid and thus increase the electron deficiency of the carbon atom to which they are attached. Secondly, they are available for donation to an attached electron-deficient carbon, and thus they may reduce the sensitivity of this carbon to nucleophilic attack. Thirdly, they are available for conjugation with the [pi]-electrons of an alkene or arene, thus increasing its electron-rich character. These effects may be summarized in 1.45-1.47. When two oxygen atoms, each possessing lone pairs, are attached to the same carbon atom, the interactions between the lone pairs become important in determining the stereochemistry of reactions.
1.1.6 Resonance
The structures of a number of compounds that contain a conjugated [pi]-system can be written as the combination of a number of contributory valence bond structures. Thus benzene can be written as a combination of the two valence bond structures 1.48 and 1.49. These contributory but non-isolable structures are known as resonance structures. The delocalization of the [pi]-electrons, arising from the combination of these valence bond structures, leads to enhanced stability.
Continues...
Excerpted from Functional Group Chemistry by James R. Hanson Excerpted by permission.
All rights reserved. No part of this excerpt may be reproduced or reprinted without permission in writing from the publisher.
Excerpts are provided by Dial-A-Book Inc. solely for the personal use of visitors to this web site.
Table of Contents
Chapter 1 General Principles: The Structure of Functional Groups; Reagents and Reactions; Learning Organic Functional Group Chemistry. Chapter 2 Chemistry of the σ-Bond: Alkanes; Alkyl Halides; Alcohols; Epoxides and Ethers; Organosulfur Compounds; Aliphatic Amines. Chapter 3 Chemistry of the π-Bond: Alkenes; Alkynes; Carbonyl Compounds; Carboxylic Acids and their Relatives; Enolate and Related Carbanion Chemistry; Nitriles, Imines and Nitro Compounds. Chapter 4 Chemistry of Aromatic Compounds: Aromatic Substitution; Aromatic Functional Groups; Heteroaromatic Compounds. Answers to Problems; Subject Index.What People are Saying About This
"...recommend the book as an useful guide for students in organic chemistry at different levels."