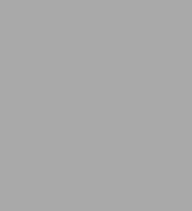
Football Physics: The Science of the Game
288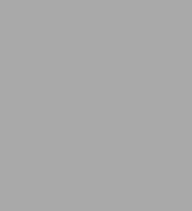
Football Physics: The Science of the Game
288eBook
Available on Compatible NOOK devices, the free NOOK App and in My Digital Library.
Related collections and offers
Overview
What effect does altitude have on the flight of a kicked ball? How do Newton's laws of motion apply to blocking and tackling? What does the science of physics reveal to us about the optimal chase strategies for defensive backs? In this illuminating , enormously entertaining book, a physics professor with a rare gift for making science easy to understand-and fun-for the lay reader reveals the unseen natural laws that govern football. And in so doing, Dr. Timothy Gay broadens and deepens our appreciation for the strategic nuances of this deceptively simple game.
The success of such well received titles as The Physics of Baseball and The Physics of Golf has already demonstrated fans' fascination with the science underlying their favorite sport. But never before have basic physical principles been explained so clearly for sports fans with no scientific background. Readers will relive the game's enthralling moments and legendary feats-Franco Harris's Immaculate Reception, Joe Montana's scrambling pass for The Catch, Dick Butkus's bone-crunching, game-saving tackles, and many more-as they gain new insight into the dynamics of blocking and tackling, open field running, kicking, passing, the line of scrimmage, and the role played by equipment, turf, and the decibels of sound in stadiums.
Illustrated with classic black-and-white NFL action photos along with engaging popular-science diagrams, the book is an outgrowth of Dr. Gay's playful, brilliant lectures on the physics of football that have been adapted by the NFL for videos shown on the TV show Blast! which airs in 190 foreign countries.
Product Details
ISBN-13: | 9781609616120 |
---|---|
Publisher: | Harmony/Rodale |
Publication date: | 09/04/2004 |
Sold by: | Random House |
Format: | eBook |
Pages: | 288 |
File size: | 6 MB |
About the Author
Read an Excerpt
CHAPTER 1
BLOCKING AND TACKLING
In football, there is a name for teams that fail to execute the fundamentals: losers. And there is nothing more fundamental in football than blocking and tackling. Incredible catches and jaw-dropping runs are fun to watch and can make the difference in a game or two over the course of a season, but they ultimately mean little if the team is failing at the basics.
A superb athlete who has been well coached and has the aggressive desire to make an impact on the game will consistently make solid tackles and blocks-- the kind that make his team's plays work and force those of his opponents to fail. A classic example of this kind of tackler is Chicago Bears middle linebacker Dick Butkus. Butkus, a Hall of Famer who played in eight straight pro bowls, had the work ethic and technical skills of a consummate professional along with the heart of a warrior. Hanging back from the line of scrimmage to get a feel for where a play was going, he would close with ferocious speed on a runner as soon as he saw him hitting a gap in the line. Looming in the runner's path with his head up and shoulders squared, he would drive through the ball carrier with an incredibly beautiful, fluid motion that often resulted in the ball coming loose and the runner lying flat on his back and driven into the turf.
The organized mayhem that occurs on a football field is governed by the fundamental laws of classical physics: Sir Isaac Newton's three laws of motion. By breaking down blocks and tackles using these laws, we can appreciate how they should be made while discovering some truly astounding aspects of such collisions. An example of this is the force that two players exert on each other during a big hit.
The Bears' ferocious Dick Butkus, number 51, wraps up the entire Green Bay front line--and makes the tackle.
FIRST, NEWTON'S FIRST LAW
We begin to gain insight into the mechanics of football collisions by considering Newton's First Law. This is the one with which you are perhaps most familiar. It says simply that mass wants to continue doing what it's doing, whether it's at rest or in motion.
While this seems pretty straightforward, the First Law is actually counterintuitive. When you think about it, our everyday experience suggests that objects want to come to a stop. If you are driving along on a level street and take your foot off the gas pedal and put the car in neutral, you'll slowly coast to a halt. We say to ourselves, "Well, sure, as soon as I stop having the engine apply a force to the car to push it along, it stops." Or if you are playing a game of pool, you can smack the cue ball and cause any of the other balls to move for a bit too, but soon they either drop into a pocket or bounce aimlessly off a bumper and slow to a stop. These everyday physics experiments seem to tell us that the natural state of matter is to be at rest.
But our naive analysis fails to factor in the braking effects of frictional forces. We may attribute the slowing and stopping of moving objects to a natural tendency on their part, but in fact the friction generated by the car's tires making contact with the road, and the pool balls rolling on the tabletop, causes this action. Newton, being the genius that he was, saw through the apparent reality to the underlying truth: Unless acted on by an external force, the natural state of matter is to continue on its initial, straight-line path indefinitely.
Think of a receiver who has caught the ball on the run and is comfortably out in front of all defenders, or a running back who has broken through the line into the secondary and finds himself in the open field. Typically, in this situation, the ball carrier will make a beeline for the end zone, stopping only after he's crossed it. (And sometimes not even then. Many fans will recall one of the most memorable plays in the history of Monday Night Football, on November 30, 1987. Bo Jackson, who was playing for the Los Angeles Raiders and celebrating his 25th birthday, took a handoff late in the second quarter from quarterback Marc Wilson and accelerated past the entire Seattle Seahawk team for an explosive 91-yard touchdown run. He crossed the goal line and kept on running--with the ball--right up into the locker-room tunnel.)
The First Law also says that the more massive an object is, the more it wants to continue doing what it's doing and the less likely it is to be deflected, slowed down, or sped up by an outside force. One example of this mass effect in action is the touchdown scored by William "The Refrigerator" Perry in Super Bowl XX. Chicago Bears head coach Mike Ditka had sent in the Fridge as a fullback on a third-and-goal running play from the 1-yard line in the third quarter as the Bears were dismantling the overmatched New England Patriots. Starting in the backfield to work up momentum, the Fridge took the handoff from quarterback Jim McMahon and surged into the end zone. Considering that Perry, normally a defensive tackle himself, weighed in at 318 £ds that day, only a massive force applied by a very large, well- placed defender would have altered his course substantially and stopped the touchdown. Not surprisingly, that didn't happen.
Newton's First Law also provides the reasoning behind why quarterback sneak plays work if well timed. The offense uses the huge mass of its interior linemen as a battering ram against the defensive line. The surge forward can be slowed or stopped, but often not before the one or two yards necessary for the play to succeed are gained.
You may be saying to yourself, "Gee, this sounds like he's telling me that it's better to be a big fast guy than a small slow guy. Duh! I knew that already." Of course you did. Not everything in physics is counterintuitive. But having said this, the First Law does have some not-so-obvious consequences. Consider an experiment I do as a lecture demonstration for my first-year physics classes. Take two bricks, one made of wood, the other of lead. If they're the size of typical bricks, the lead one will weigh about 30 £ds; the wood brick, 2 £ds. Now support each brick carefully on two 8-ounce Styrofoam coffee cups set mouth down. (You'll have to be careful to avoid crushing the cups as you place the lead brick on them.) From a height of 1 meter, drop a 1-£d weight straight down on the center top of both bricks. Which brick will do a better job of crushing the coffee cups under the blow of the falling weight?
When I ask my students to predict the answer, typically more than 90 percent say that the lead brick will do more damage. That's just plain old common sense, right? But remember what Newton tells us: The more massive an object is, the more it wants to do what it was doing before. In the case of these bricks, what they were doing was sitting at rest before being hit. The lead brick, being about 15 times more massive than the wooden one, is much less interested in moving. As a result, it accelerates less violently into the coffee cups and does a lot less damage.
So while, generally speaking, it is better to be a big fast guy than a small slow guy, that isn't the whole story. Incidentally, for those of you who like to bet on football games, you may want to consider a new moneymaking opportunity. The next time you and your buddies are watching a game together, get out some Styrofoam cups and a couple of bricks . . .
THE FRIDGE'S ESSENTIAL CHARACTERISTIC: MASS
Newton's Second Law, which is really the basis of all classical physics, says that the force applied to an object is the product of the object's mass and its acceleration. Mathematically, we say that F = ma. We can use this formula to appreciate just how much force is expended when one player hits another, and how much force exerted over a given time would have been needed to stop Refrigerator Perry from scoring on that running play. First, though, we must define our terms.
The mass (m) of an object is basically the amount of matter--the number of atoms--it has in it. Mass and weight are connected, but they are not the same thing. An astronaut who weighs 250 £ds on earth has a mass of 114 kilograms. On the moon, the same guy weighs only 40 £ds, but he has the same number of atoms and, hence, the same mass: 114 kilograms. While mass is a measure of how much matter an object has, weight is a measure of how strongly this mass is attracted by gravity to whatever planet or asteroid the object happens to be sitting on.
We talk about how big an offensive guard or defensive tackle is in terms of his weight, but when we are trying to determine how hard he can hit the guy across the line of scrimmage, what we actually care about is his mass. Fortunately, in the earth's gravitational field, the player's weight and mass are linked by a simple conversion formula: 2.2 £ds is the equivalent of 1 kilogram. We will routinely use the term £d (or £d- mass) when referring to mass, for the sake of sanity and simplicity. Just remember that a £d of mass is the mass associated with an object that weighs 1 £d on earth.
ACCELERATION, SPEED, AND POSITION: KINEMATICS
After mass (m), the next variable in our equation F = ma is acceleration (a). Acceleration should not be confused with speed. Speed tells us how rapidly an object is changing its position, while acceleration tells us how rapidly this speed is changing in time. Notice that this definition of speed is no more complicated than the one you probably used (or attempted to use) to solve the old "two trains leaving the station" problem in high school: the distance you travel is equal to your average speed multiplied by how long you've been going. In football, we use speed to measure the time a player needs to travel a certain distance: "He runs a 4-7 40." This means he can run 40 yards in 4.7 seconds from a standing start. His average speed is thus 40 yards divided by 4.7 seconds, or 8.5 yards per second. (This is equivalent to 25.5 feet per second.) We talk about a player's "average" speed because over the course of a run he is likely to speed up or slow down.
Another term related to speed is velocity. Velocity is a speed specified in connection with a direction. Put another way, speed tells you how fast you're moving along the path you're taking, while velocity tells you your speed and the direction in which you're going. The units of both speed and velocity are feet per second, abbreviated "ft/s." The units of acceleration are feet per second per second. Since "feet per second" is a speed, a rate of change of speed over time must have another "per second" in it. In physics we write this as "ft/s2," but we can also spell it out as "feet per second squared."
To put all these terms in football perspective, let's consider Jerry Rice running a deep pattern, moving to our left. We'll use the yard markers that he passes to help us keep track of things. Starting from rest at the line of scrimmage, his own 10-yard line, he increases his speed until he reaches his top end--say, 32 feet per second. During the time that his speed is increasing, he runs from the 10 to about the 20. His acceleration is roughly 16 feet per second squared over this stretch. This means that after the first second, he is running with a speed of 16 feet per second. After 2 seconds he's running at his maximum speed of 32 feet per second. In other words, his speed is increasing at the rate of 16 feet per second every second. Rice's top-end speed of 32 feet per second tells us that he will pass two 5-yard markers in a little less than a second once he's running flat out. While his speed is now 32 feet per second, his velocity is 32 feet per second to the left.
These quantities--speed, velocity, and acceleration--are the elements that make up the branch of physics called kinematics, or the science of motion. Galileo was the first scientist to make a comprehensive study of kinematics. Newton's Second Law is important to us here because it connects motion (kinematics) with the "dynamical" quantities of force and mass.
FIGURING OUT THE FORCE OF A BIG HIT
Remember that we entered this whole discussion about acceleration and speed to begin to appreciate, using Newton's Second Law, the force involved in a big hit from the likes of a Jack Tatum, "Mean" Joe Greene, Rod Woodson, Ronnie Lott, John Lynch, or any of the many other superior blockers and tacklers that have played the game or do today.
So let's get down to it. What is force? Simple. According to Newton's Second Law, force is the thing that speeds mass up or slows it down--in other words, gives it an acceleration or deceleration. (This is strictly true only for one-dimensional motion. In two-dimensional motion, it is possible for forces to change an object's direction but not its speed. For example, when a running back is given a sharp, impulsive blow from the side by a defensive tackle. the hit doesn't necessarily slow him down, but it will deflect his path. We'll discuss this issue more in chapter 3.)
A force must be exerted by something that is in contact with the object being accelerated--some active agent like a string, a spring, a finger, or an enraged Dick Butkus. This may sound trivially obvious, but the misunderstanding of this point is the cause of much confusion. When talking to my students in introductory physics classes, I often hear things like "The force of the truck accelerating causes the collision," or "His speed exerts a force on the opposing player." Acceleration and speed do not produce force--only things in contact with the object in question can exert a force on it. Strings, springs, fingers, Butkus!