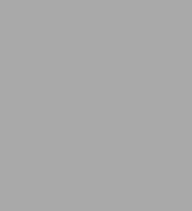
Food Contact Materials Analysis: Mass Spectrometry Techniques
273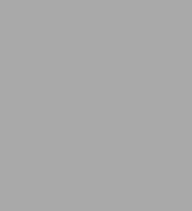
Food Contact Materials Analysis: Mass Spectrometry Techniques
273eBook
Available on Compatible NOOK devices, the free NOOK App and in My Digital Library.
Related collections and offers
Overview
Mass spectrometric techniques have developed over recent years to offer ever increasing solutions to solving problems in food processing and packaging. Even the smallest amount of contamination in food can cause a problem for food production companies, thus they are keen to find speedy and efficient quality control methods.
This book outlines how ingredients and their interrelationship with processing and packaging have developed with the exploitation of mass spectrometry and gives practical protocols to stake holders showing the flexibility of this technique.
With huge relevance worldwide, this book will appeal to food packaging scientists and mass spectrometry practitioners alike.
Product Details
ISBN-13: | 9781788017190 |
---|---|
Publisher: | Royal Society of Chemistry |
Publication date: | 01/18/2019 |
Series: | ISSN |
Sold by: | Barnes & Noble |
Format: | eBook |
Pages: | 273 |
File size: | 9 MB |
Read an Excerpt
CHAPTER 1
Risk Assessment of Plastic-based Food Contact Materials: Focus on Polyolefins
FABIO TESTONI AND INES MINGOZZI
1.1 Introduction
Polyolefins is a term indicating a class of polymers commonly used for, among other applications, food packaging. They are produced starting from one or more monomers, polymerization aids (catalysts, peroxides, etc.) and polymer production aids (i.e. in-process antistatic agents). Solvents can also be present. The resulting base polymer is then formulated with additives. Polymers in their final form (generally pellets, granules or flakes) are sold to converters to produce articles.
Monomer(s), additives, polymerization aids (PAs) and polymer production aids (PPAs) are considered intentionally added substances (IASs). Impurities within starting raw materials and reaction products from the manufacturing process (i.e. oligomers, additive by-products) are defined as non-intentionally added substances (NIASs) and are subjected to risk assessment, in addition to PAs and PPAs if not listed in EU or National Food Contact Regulations.
Regulation (EC) No. 1935/2004 of the European Parliament and of the Council of 27 October 2004 provides general principles of safety and inertness for all food contact materials (FCMs). In addition, EU Regulation No. 10/2011 as amended sets out rules for plastic materials in contact with food. EU Regulation No. 10/2011 describes requirements for migration tests. There are two types of limits: OML (overall migration limit) and SML (specific migration limit). OML is based on the total mass of substances that can migrate (gravimetric analysis), whereas SML is based only on quantification of one specific substance that can migrate into food.
EU Regulation No. 10/2011 is based on the principle of a "positive list" and Annex 1 contains the list of authorized chemicals (monomers, additives and certain PPAs). Some listed substances can have an SML. Other substances that are not required to be listed, such as PAs and NIASs, need to be risk assessed.
When a polymer is used for food contact applications, there are important material features that must be considered before approaching any further analytical challenge related to risk assessment:
ODOUR: According to current regulation: "... any material or article intended to come into contact directly or indirectly with food must be sufficiently inert to preclude substances from being transferred to food in quantities large enough to endanger human health or to bring about an unacceptable change in the composition of the food or a deterioration in its organoleptic properties." This means that it is necessary to monitor packaging material components that could migrate into food, deteriorating safety and quality, starting from a low molecular weight.
NIASs: According to EFSA (European Food Safety Association), reaction products and impurities (NIASs) that are not included in the EU list of authorized substances in EU Regulation No. 10/2011 are required to be risk assessed, irrespective of their source or intended function. Oligomers are also considered as NIASs. Their identification and quantification are required for risk assessment by Art. 19 of EU Food Contact Regulations 10/2011/EC, specifically for those oligomers with molecular weight (MW) <1000 dalton (Da), as potential migrants of concern which, after transfer (migration) into food, may be physiologically resorbed after ingestion.
1.2 Evaluation of Analytical Approaches
Polymer characterization usually consists of fractionation/extraction or thermal desorption. Fractionation/extraction can be carried out using different solvents and techniques. Aiming to investigate all the species present in an FCM, a combination of analytical methods is used. With polypropylene, a commonly used approach is the separation of the insoluble crystalline part (XITL[i) from the soluble atactic fraction and oils. Methylene chloride (CH2Cl2) is another solvent that is commonly used. The fractionated or extracted part can be analysed using different instrumental techniques: NMR (nuclear magnetic resonance), GPC (gel permeation chromatography), GC-FID (gas chromatography coupled with flame ionization detection), GC-MS (gas chromatography coupled with mass spectrometry), HPLC-MS or HPLC-DAD (high-performance liquid chromatography coupled with mass spectrometry or diode-array detection, respectively). The polymer as such can also be thermally desorbed and studied by GC-MS and GC-FID (see Figure 1.1).
What emerges is a very complex matrix, with species covering a very wide MW range, starting from a few daltons (residual monomer) up to millions of daltons (high-MW polymers with high crystallinity), as shown in Figure 1.2. This scheme is characteristic and different for each individual polymer.
Considering a very common FCM, i.e. a printed film to wrap food, a deeper investigation reveals a very complicated situation (see Figure 1.3). At least four different polymers were used, laminated with a printed surface and glue.
Analysis of a final item is very difficult and the result is a sum of the contributions from each individual layer component, including the printed layer (with or without paper, when present) and glue, and heating effects during extrusion/lamination. Moreover, it is worth remembering that each film layer can be formed by more than a single polymer.
Very complex matrices, due to different plastic materials and printing and lamination processes, are usually challenging to analyse if the whole production chain is unknown. The final item is analysed to characterize NIASs, but it would often be desirable to obtain more information about the origin of the NIASs.
For the above reasons, to achieve a full characterization and understanding of FCMs, each individual polymer should be analysed as such, together with the laminated final film with and without the printed and glued layer.
1.3 GPC: First Approach
Polymer analysis is usually carried out by GPC, in particular to describe finally the MW distribution. It could also be considered as a first approach to characterizing oligomers with MW <1000 Da. The separation path is based on the different retention times of molecules of a polymer in solution. Chemical interaction between the particles and the stationary phase can be considered negligible. In addition, no information about the chemical nature of compounds is obtained.
Starting from polypropylene homopolymers (PP HOMOs), Figure 1.4 shows three different materials with the same average MW but different MW distributions, due to different synthesis conditions.
The resulting GPC traces are shown at top left in Figure 1.4 and the NIAS–oligomers region is the grey circled zone. Even if clear differences in the MW distributions for the three samples can be measured in terms of average MW (Mn, Mw, Mz), no accurate speculations are feasible for the NIAS region via GPC. To overcome the poor resolution and separating power of GPC, GC must be considered.
1.4 GC-MS and HPLC-MS Approaches
For comprehensive and full characterization of ODOUR, NIAS (or IAS) substances, including oligomers, a deep and more sophisticated analytical approach is needed. Generally, an extraction step of the potential migrants from the matrices is applied and then GC or LC separation is performed. Thermal desorption (static or dynamic) is applied for volatile compounds, whereas solvent extraction is needed for the characterization of less volatile species. Capillary GC and HPLC provide the required separation, selectivity and efficiency.
As for the detector to be used, not only should it give as much information as possible for each separated organic compound, but it should also have good sensitivity. A mass spectrometer coupled with capillary GC or HPLC fits all these requirements.
Capillary GC, coupled with FID, is also widely used in many studies as it provides a relatively easy and universal quantitative approach. The FID response for hydrocarbons can be considered as weight percent response, even without any use of external standard (ESTD) or internal standard (ISTD) calibration.
Within capillary GC, the main technique used for volatile organic compounds (VOCs), three different sample analyses are necessary, starting from static headspace (SHS) for the C3–C24 range, passing through a dynamic head-space–thermal desorption system (TDS) for C9–C48, and arriving at solvent extraction for C9–C60 or heavier compounds. Hydrocarbons up to C100 can also be eluted, if high-temperature-resistant capillary columns are used. Cn roughly indicates the number of carbon atoms in the possible eluted species.
To detect additives (IASs) or some high-MW additive by-products (NIASs), HPLC-DAD-MS is necessary. HPLC cannot be used to quantify oligomers because, owing to their chemical nature, they are not detectable with either MS or DAD.
APCI (atmospheric pressure chemical ionization), ESI (electrospray ionization) or APPI (atmospheric pressure photoionization) sources are not effective in aliphatic hydrocarbon ionization. Different extraction methods have been described, but essentially they are based on UASE (ultrasonic-assisted solvent extraction), OSM (one-step microwave-assisted extraction) or TSM (two-step microwave-assisted extraction).
In order to detect NIASs or IASs with SML using HPLC-MS, a quadrupole instrument is not sufficient. APCI, ESI and APPI are considered soft ionization techniques, so they do not produce EI (electron ionization)-like spectra, with fragmentation patterns of analytes, but only a few ions for each compound (i.e. molecular ion and one or two fragments). These few ions and their relative abundances (fragmentation pattern) depend on the solvent and temperature. Consequently, these fragmentation patterns differ considerably from those available in the database currently used for species recognition analysis and they are not searchable in commercially available mass spectral libraries. Only by using the exact mass (2 ppm or better resolution) it is possible to establish the empirical formula of the analyte. If CAD (collisionally activated dissociation) is used both in single-stage MS and multi-stage mass spectrometry (MSn), it is possible to obtain a fragmentation pattern.
Since DAD is not a destructive detection method, it can be used before MS with very good results. Coupling DAD with MS (TOF), identification of some IASs (see Figure 1.5) or NIASs (see Figures 1.6 and 1.7) can be carried out using exact mass calculation. The UV spectrum, when reported in the literature, can also be helpful.
1.5 Samples
Samples suitable for GC-MS or GC-FID investigation can be spheres or particles (polymer before extrusion), pellets, films, items or food as such. Usually, the sample amount is 1 g, for the SHS method a few grams (2–5 g) for solvent extraction and less than 1 g for TDS. If solvent extraction or SHS is used, spheres and pellets require grinding before treatment. An FCM (final item) requires cutting or grinding. TDS can be used with a few spheres or pellets, but manufactured items and films need to be cut into small pieces before thermal desorption.
Any sample manipulation should be carried out wearing cotton gloves, to avoid sample contamination. Plastic gloves should not be used, to avoid "ghost peaks." Contamination due to ubiquitous substances or interfering compounds should be considered in solvents used for HPLC and/or extraction.
1.6 Characterization: SHS
SHS is wrongly considered a relatively easy technique. Reality is different, and the results should be carefully evaluated before drawing any conclusion. Together with TDS-GC-MS, it is the main technique used to investigate odour.
Very exhaustive discussions about SHS, the amount sample and its treatment, relative vial pressure, etc., can be found in the literature. In the following, a few practical considerations are discussed.
Mechanisms of odour formation and its transport, together with the odour threshold, have been described in the literature. If food as such is analysed, useful references can be found in a flavour reference handbook.
SHS does not require any particular equipment except a glass vial that can be closed with an inert septum. Vials of size 20 mL are the most commonly used, sealed with silicone or a butyl rubber internally PTFE-coated septum. Sample heating, vial gas-phase injection and chromatogram evaluation complete the analysis (see Figure 1.8).
The sample amount should not be greater than 1–2 g. The maximum and analyte quantitation are described by the equation
peak area [varies] CG = C0/K + β (1.1)
where CG = concentration of the analyte in the headspace, C0 = original sample concentration of the analyte, K (partition coefficient) = CS/CG, CS = concentration of analyte remaining in sample, β (phase ratio of the vial) = VG/VS, VG = volume of the gas phase and VS = volume of the liquid–solid sample.
If peak identification only is required, a search of mass spectral libraries is the first necessary step. If quantitation is also necessary, it is very important not to forget some fundamental concepts.
For each individual component, starting from residual monomer(s), up to oxygen-based or odorous substances, passing through hydrocarbons (C2–C24), the partition coefficient K must be considered in order to avoid incorrect analytical results.
The first approach in material investigation can be carried out by heating the closed vial containing the sample without any grinding. GC-MS and interpretation of the mass spectra give a rough indication of the compounds present in the gas phase. Chromatographic separation can be achieved with apolar columns with a thick film phase (VOC-like) or polar columns (e.g. Carbowax-like).
Of course, the gas-phase concentration and K differ not only according to the heating temperature but also to the nature of the matrix (different polymers give different K values for the same substance), matrix particle size, nature of the analyte [polar, non-polar, its boiling point (bp), etc.] and equilibration time. The heating temperature and K are the limiting factors.
If an internal standard (ISTD) is used, it must be selected to be as similar as possible to the substance or chemical clusters to be quantitated (i.e. comparable K or similar bp). An example, obtained using FID, is shown in Figure 1.9.
If toluene is used, hydrocarbons with similar bp (C9) can be quantified. If hexadecane is used, C12–C15 hydrocarbons can be studied. Of course, a single ISTD must not be used to quantify the whole chromatogram.
To determine the effect of different post-polymerization treatments on the VOC population, SHS coupled with GC-MS can be used. An effective comparison can be obtained by heating the same sample type and amount at the same temperature for the same time (see Figure 1.10).
Grinding the cooled polymer before applying the SHS technique is strongly suggested. For some analytes, too long a period of time would be necessary to reach equilibrium with the gas phase if the sample has not been milled. Acetone and TBA (tert-butyl alcohol), typical by-products of some peroxides used for "visbreaking" high-MW distribution polymers, are good examples (see Figure 1.11). Visbreaking is a process commonly used in polymer production. A peroxide is used to decrease the average MW of the polymers (viscosity reduction). It involves radical scission of high-MW chains.
Consider further that not only do acetone and TBA have different K values, but also K depends strongly on the heating temperature and on the polymeric matrix. A homopolymer (Base 1) and a heterophasic copolymer (HECO 2), both milled, were studied at different SHS temperatures (60 and 100 °C). The K values obtained for acetone (together with a co-eluted by-product interferent) and TBA are given in Table 1.1.
(Continues…)
Excerpted from "Food Contact Materials Analysis"
by .
Copyright © 2019 The Royal Society of Chemistry.
Excerpted by permission of The Royal Society of Chemistry.
All rights reserved. No part of this excerpt may be reproduced or reprinted without permission in writing from the publisher.
Excerpts are provided by Dial-A-Book Inc. solely for the personal use of visitors to this web site.