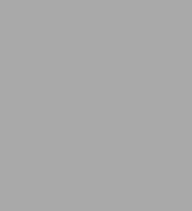
Fluid Mechanics and Thermodynamics of Turbomachinery
477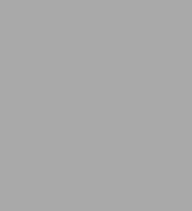
Fluid Mechanics and Thermodynamics of Turbomachinery
477eBook
Available on Compatible NOOK devices, the free NOOK App and in My Digital Library.
Related collections and offers
Overview
Turbomachinery is a challenging and diverse field, with applications for professionals and students in many subsets of the mechanical engineering discipline, including fluid mechanics, combustion and heat transfer, dynamics and vibrations, as well as structural mechanics and materials engineering. Originally published more than 40 years ago, Fluid Mechanics and Thermodynamics of Turbomachinery is the leading turbomachinery textbook. Used as a core text in senior undergraduate and graduate level courses this book will also appeal to professional engineers in the aerospace, global power, oil&gas and other industries who are involved in the design and operation of turbomachines. For this new edition, author S. Larry Dixon is joined by Cesare Hall from the University of Cambridge, whose diverse background of teaching, research and work experience in the area of turbomachines is well suited to the task of reorganizing and updating this classic text.
- Provides the most comprehensive coverage of the fundamentals of turbomachinery of any text in the field
- Content has been reorganized to more closely match how instructors currently teach the course, with coverage of fluid mechanics and thermodynamics moved to the front of the book
- Includes new design studies of several turbomachines, applying the theories developed in the book
Product Details
ISBN-13: | 9780080962597 |
---|---|
Publisher: | Elsevier Science |
Publication date: | 02/17/2010 |
Sold by: | Barnes & Noble |
Format: | eBook |
Pages: | 477 |
File size: | 12 MB |
Note: | This product may take a few minutes to download. |
About the Author
Dr. Hall has been University Lecturer in turbomachinery at the University of Cambridge since 2005. His current research with the university’s Silent Aircraft Initiative has led to the development of radical new ideas for aircraft engine design. Prior to teaching, he worked at Rolls-Royce as a turbomachinery aerodynamicist.
Read an Excerpt
Fluid Mechanics and Thermodynamics of Turbomachinery
By S. L. Dixon C. A. Hall
Butterworth-Heinemann
Copyright © 2010 S. L. Dixon and C. A. HallAll right reserved.
ISBN: 978-0-08-096259-7
Chapter One
Introduction: Basic Principles
Take your choice of those that can best aid your action. Shakespeare, Coriolanus
1.1 DEFINITION OF A TURBOMACHINE
We classify as turbomachines all those devices in which energy is transferred either to, or from, a continuously flowing fluid by the dynamic action of one or more moving blade rows. The word turbo or turbinis is of Latin origin and implies that which spins or whirls around. Essentially, a rotating blade row, a rotor or an impeller changes the stagnation enthalpy of the fluid moving through it by doing either positive or negative work, depending upon the effect required of the machine. These enthalpy changes are intimately linked with the pressure changes occurring simultaneously in the fluid.
Two main categories of turbomachine are identified: firstly, those that absorb power to increase the fluid pressure or head (ducted and unducted fans, compressors, and pumps); secondly, those that produce power by expanding fluid to a lower pressure or head (wind, hydraulic, steam, and gas turbines). Figure 1.1 shows, in a simple diagrammatic form, a selection of the many varieties of turbomachines encountered in practice. The reason that so many different types of either pump (compressor) or turbine are in use is because of the almost infinite range of service requirements. Generally speaking, for a given set of operating requirements one type of pump or turbine is best suited to provide optimum conditions of operation.
Turbomachines are further categorised according to the nature of the flow path through the passages of the rotor. When the path of the through-flow is wholly or mainly parallel to the axis of rotation, the device is termed an axial flow turbomachine [e.g., Figures 1.1(a) and (e)]. When the path of the through-flow is wholly or mainly in a plane perpendicular to the rotation axis, the device is termed a radial flow turbomachine [e.g., Figure 1.1(c)]. More detailed sketches of radial flow machines are given in Figures 7.3, 7.4, 8.2, and 8.3. Mixed flow turbomachines are widely used. The term mixed flow in this context refers to the direction of the through-flow at the rotor outlet when both radial and axial velocity components are present in significant amounts. Figure 1.1(b) shows a mixed flow pump and Figure 1.1(d) a mixed flow hydraulic turbine.
One further category should be mentioned. All turbomachines can be classified as either impulse or reaction machines according to whether pressure changes are absent or present, respectively, in the flow through the rotor. In an impulse machine all the pressure change takes place in one or more nozzles, the fluid being directed onto the rotor. The Pelton wheel, Figure 1.1(f), is an example of an impulse turbine.
The main purpose of this book is to examine, through the laws of fluid mechanics and thermodynamics, the means by which the energy transfer is achieved in the chief types of turbomachines, together with the differing behaviour of individual types in operation. Methods of analysing the flow processes differ depending upon the geometrical configuration of the machine, whether the fluid can be regarded as incompressible or not, and whether the machine absorbs or produces work. As far as possible, a unified treatment is adopted so that machines having similar configurations and function are considered together.
1.2 COORDINATE SYSTEM
Turbomachines consist of rotating and stationary blades arranged around a common axis, which means that they tend to have some form of cylindrical shape. It is therefore natural to use a cylindrical polar coordinate system aligned with the axis of rotation for their description and analysis. This coordinate system is pictured in Figure 1.2. The three axes are referred to as axial x, radial r, and tangential (or circumferential) rθ.
In general, the flow in a turbomachine has components of velocity along all three axes, which vary in all directions. However, to simplify the analysis it is usually assumed that the flow does not vary in the tangential direction. In this case, the flow moves through the machine on axi symmetric stream surfaces, as drawn on Figure 1.2(a). The component of velocity along an axi-symmetric stream surface is called the meridional velocity,
cm = [square root of c2x + c2r]. (1.1)
In purely axial-flow machines the radius of the flow path is constant and therefore, referring to Figure 1.2(c) the radial flow velocity will be zero and cm = cx. Similarly, in purely radial flow machines the axial flow velocity will be zero and cm = cr. Examples of both of these types of machines can be found in Figure 1.1.
The total flow velocity is made up of the meridional and tangential components and can be written
[MATHEMATICAL EXPRESSION NOT REPRODUCIBLE IN ASCII] (1.2)
The swirl, or tangential, angle is the angle between the flow direction and the meridional direction:
α = tan-1 (cθ/cm). (1.3)
Relative Velocities
The analysis of the flow-field within the rotating blades of a turbomachine is performed in a frame of reference that is stationary relative to the blades. In this frame of reference the flow appears as steady, whereas in the absolute frame of reference it would be unsteady. This makes any calculations significantly more straightforward, and therefore the use of relative velocities and relative flow quantities is fundamental to the study of turbomachinery.
The relative velocity is simply the absolute velocity minus the local velocity of the blade. The blade has velocity only in the tangential direction, and therefore the relative components of velocity can be written as
wθ = cθ - U, wx = cx, wr = cr. (1.4)
The relative flow angle is the angle between the relative flow direction and the meridional direction:
β = tan-1(wθ/cm). (1.5)
By combining eqns. (1.3), (1.4), and (1.5) a relationship between the relative and absolute flow angles can be found:
tanβ = tan α - U/cm. (1.6)
1.3 THE FUNDAMENTAL LAWS
The remainder of this chapter summarises the basic physical laws of fluid mechanics and thermodynamics, developing them into a form suitable for the study of turbomachines. Following this, some of the more important and commonly used expressions for the efficiency of compression and expansion flow processes are given.
The laws discussed are
(i) the continuity of flow equation;
(ii) the first law of thermodynamics and the steady flow energy equation;
(iii) the momentum equation;
(iv) the second law of thermodynamics.
All of these laws are usually covered in first-year university engineering and technology courses, so only the briefest discussion and analysis is given here. Some textbooks dealing comprehensively with these laws are those written by Çengel and Boles (1994); Douglas, Gasiorek, and Swaffield (1995); Rogers and Mayhew (1992); and Reynolds and Perkins (1977). It is worth remembering that these laws are completely general; they are independent of the nature of the fluid or whether the fluid is compressible or incompressible.
1.4 THE EQUATION OF CONTINUITY
Consider the flow of a fluid with density ρ, through the element of area dA, during the time interval dt. Referring to Figure 1.3, if c is the stream velocity the elementary mass is dm = ρcdtdA cosθ, where θ is the angle subtended by the normal of the area element to the stream direction. The element of area perpendicular to the flow direction is dAn = dA cosθ and so dm = ρcdAndt. The elementary rate of mass flow is therefore
d[??] = dm/dt = ρcdAn. (1.7)
Most analyses in this book are limited to one-dimensional steady flows where the velocity and density are regarded as constant across each section of a duct or passage. If An1 and An2 are the areas normal to the flow direction at stations 1 and 2 along a passage respectively, then
[??] = ρ1c1An1 = ρ2c2An2 = ρcAn, (1.8)
since there is no accumulation of fluid within the control volume.
1.5 THE FIRST LAW OF THERMODYNAMICS
The first law of thermodynamics states that, if a system is taken through a complete cycle during which heat is supplied and work is done, then
[MATHEMATICAL EXPRESSION NOT REPRODUCIBLE IN ASCII] (1.9)
where [??] dQ represents the heat supplied to the system during the cycle and [??] dW the work done by the system during the cycle. The units of heat and work in eqn. (1.9) are taken to be the same.
During a change from state 1 to state 2, there is a change in the energy within the system:
E2 - E1 = ∫21 (dQ - dW), (1.10a)
where E = U + 1/2mc2 + mgz.
For an infinitesimal change of state,
dE = dQ - dW. (1.10b)
The Steady Flow Energy Equation
Many textbooks, e.g., Çengel and Boles (1994), demonstrate how the first law of thermodynamics is applied to the steady flow of fluid through a control volume so that the steady flow energy equation is obtained. It is unprofitable to reproduce this proof here and only the final result is quoted. Figure 1.4 shows a control volume representing a turbomachine, through which fluid passes at a steady rate of mass flow [??], entering at position 1 and leaving at position 2. Energy is transferred from the fluid to the blades of the turbomachine, positive work being done (via the shaft) at the rate [??]x. In the general case positive heat transfer takes place at the rate [??], from the surroundings to the control volume. Thus, with this sign convention the steady flow energy equation is
[MATHEMATICAL EXPRESSION NOT REPRODUCIBLE IN ASCII], (1.11)
where h is the specific enthalpy, 1/2c2, the kinetic energy per unit mass and gz, the potential energy per unit mass.
For convenience, the specific enthalpy, h, and the kinetic energy, 1/2c2, are combined and the result is called the stagnation enthalpy:
h0 = h + 1/2c2. (1.12)
Apart from hydraulic machines, the contribution of the g(z2 - z1) term in eqn. (1.11) is small and can usually ignored. In this case, eqn. (1.11) can be written as
[MATHEMATICAL EXPRESSION NOT REPRODUCIBLE IN ASCII] (1.13)
The stagnation enthalpy is therefore constant in any flow process that does not involve a work transfer or a heat transfer. Most turbomachinery flow processes are adiabatic (or very nearly so) and it is permissible to write [??] = 0. For work producing machines (turbines) [??]x > 0, so that
[MATHEMATICAL EXPRESSION NOT REPRODUCIBLE IN ASCII] (1.14)
For work absorbing machines (compressors) [??]x < 0, so that it is more convenient to write
[MATHEMATICAL EXPRESSION NOT REPRODUCIBLE IN ASCII] (1.15)
(Continues...)
Excerpted from Fluid Mechanics and Thermodynamics of Turbomachinery by S. L. Dixon C. A. Hall Copyright © 2010 by S. L. Dixon and C. A. Hall. Excerpted by permission of Butterworth-Heinemann. All rights reserved. No part of this excerpt may be reproduced or reprinted without permission in writing from the publisher.
Excerpts are provided by Dial-A-Book Inc. solely for the personal use of visitors to this web site.
Table of Contents
Chapter 1. Introduction: Basic Principles
Chapter 2. Dimensional Analysis: Similitude
Chapter 3. Two-Dimensional Cascades
Chapter 4. Axial-Flow Turbines: Mean-Line Analysis and Design
Chapter 5. Axial-Flow Compressors and Ducted Fans
Chapter 6. Three-Dimensional Flows in Axial Turbomachines
Chapter 7. Centrifugal Pumps, Fans, and Compressors
Chapter 8. Radial Flow Gas Turbines
Chapter 9. Hydraulic Turbines
Chapter 10. Wind Turbines
What People are Saying About This
This market leading text provides comprehensive and balanced coverage of the theory and applications of turbomachinery – now updated with modern tools and practices used in industry.