Feynman Lectures On Gravitation / Edition 1 available in Hardcover, Paperback, eBook
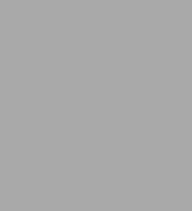
Feynman Lectures On Gravitation / Edition 1
- ISBN-10:
- 0813340381
- ISBN-13:
- 9780813340388
- Pub. Date:
- 06/20/2002
- Publisher:
- Taylor & Francis
- ISBN-10:
- 0813340381
- ISBN-13:
- 9780813340388
- Pub. Date:
- 06/20/2002
- Publisher:
- Taylor & Francis
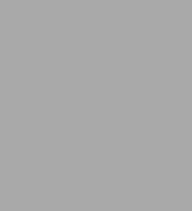
Feynman Lectures On Gravitation / Edition 1
Buy New
$71.99Overview
Product Details
ISBN-13: | 9780813340388 |
---|---|
Publisher: | Taylor & Francis |
Publication date: | 06/20/2002 |
Series: | Frontiers in Physics |
Pages: | 280 |
Product dimensions: | 6.00(w) x 9.00(h) x (d) |
About the Author
Read an Excerpt
Lecture 13
13.2 ON THE POSSIBILITY OF A NONUNIFORM AND NONSPHERICAL UNIVERSE
.Since all our conclusions have been so heavily based on the postulate of a uniform cosmology, we might review the nature of the evidence. If we examine a region of the universe within 1.3 x 108 light-years from us, we find simply one cluster, the Virgo cluster-in other words, the matter distributed in a strongly unsymmetric fashion. The lack of symmetry in this region is so large that it can't be explained away. For example, it cannot be due to obscuring by galactic dust, which could affect only that region of the sky lying within a few degrees of the galactic equator. The uniformity must show up in examining regions which are large compared to 108 ly, since the number of galaxies in the usual cluster is much too large to be comfortably described as a fluctuation of the density. What is encouraging is that in very faraway regions the sky seems to be populated by very regular and compact clusters of clusters, involving perhaps thousands of nebulae, all swarming about as bees, relative to the center of gravity of the supercluster. The dispersion of the red shifts shows that the velocities relative to the center of mass must be of the order of 1000 km/sec. This dispersion serves as a very useful measure of the mass of the clusters. It is evident that the clusters are formed by gravitational attractions, and that they are entities which have a long life-they are stable bound systems. From this information we deduce the mass, because we know that velocities of 1000 km/sec are insufficient for escape. There are some worries in this correction; for example if we make a similar computation for the mass of the Virgo cluster, we get a mass 30 times smaller than by other means. Yet the faraway superclusters are larger so that the computation may be more reliable.
The very existence of clusters shows that nebulae attract each other with sufficient strength that these systems are held together for times comparable to the age of the universe. It is very interesting to note that almost all galaxies are in clusters; only a very small fraction appear to exist not in a cluster. The conclusion is that nearly all of the visible matter of the universe does not have sufficient kinetic energy to escape from other matter nearby. In view of this fact, it seems to me that it is very unlikely that the average density of the universe is much smaller than the critical density. If the density is much smaller, the formation of the clusters must be ascribed to local fluctuations which made matter denser in some regions. But on a statistical basis it would be very difficult that there should be enough local fluctuations of the right kind so that nearly all matter occurs in clusters. It might be argued that at an earlier time when the density was greater such fluctuations might be easier-but I have not seen a quantitative argument based on this idea. The inescapable conclusion is that most matter gets pulled into clusters because the gravitational energy is of the same order as the kinetic energy of the expansion-this to me suggests that the average density must be very nearly the critical density everywhere.
The preceding guesses about the average density do not lend any support to the hypothesis of uniformity. It could still be that the question as to whether the matter in given regions holds together is purely local, and the situation varies from region to region. The cosmological principle can be studied only by making a detailed comparison of the density of matter and the nonlinearity of the red shift, which represents an acceleration of the galaxies. These are quantities which are in principle independently measurable, yet a definite relation between them is predicted by theories adopting the cosmological principle. If the variation of density with radial distance were to be measured with great accuracy, we might find that maybe matter is too dense in the inner region, in such a way that the cosmological principle cannot hold.
Even if the cosmological principle were wrong, it would be possible for the universe to have a spherical symmetry-a prejudice in favor of the cosmological principle reflects our surprise at finding that we are at what looks like the center of the universe. Let us assume that the visible region is nearly symmetric but that outside there is more matter, distributed in a lopsided fashion. What would be the difference? We would expect a first order correction to the motion of the distant nebulae to be due to the tidal forces as illustrated in Figure 13.1. (If the visible region were accelerating as a whole we would not be aware of the acceleration!) The result would be a correction to the red shift having a quadrupole character; the red shift should be redder in two polar regions and bluer in a equatorial region.
The preceding discussions show us how little the relativistic theory of gravitation tells us about cosmology. The central problems of cosmology can be solved only when we actually know what the universe "really" looks like, when we have accurate plots of red shifts and densities as a function of distance and position in the galactic sky.
13.3 DISAPPEARING GALAXIES AND ENERGY CONSERVATION
Let us briefly mention some of the other interesting puzzles of cosmology and cosmological models. Is it possible for certain nebulae to vanish from view forever? With our present theory, the answer is no; regardless of whether the density is greater or smaller than critical, no galaxy which is now visible will ever disappear entirely, although it may get redder and redder. It makes no sense to worry about the possibility of galaxies receding from us faster than light, whatever that means since they would never be observable by hypothesis.
In Hoyle's theory, the nebulae fax away do vanish; his expression for the recession velocity is such that the volume of a cube defined by eight given galaxies doubles in every time interval of a certain size. The velocities are thus postulated to increase so that some do accelerate to speeds faster than c. However, Hoyle's theory does not pretend to explain the motion of the galaxies in terms of forces. It simply states a kinematic rule for calculating specified positions as a function of time, and the specifications are such that galaxies do disappear from view eventually. There is little point in searching for a force in nature which might result in Hoyle's expansion-It would have to be of an unfamiliar type, since no finite forces could ever result in such a motion in the framework of the present mechanics.
I have inherited a prejudice from my teacher, Dr. Wheeler, to consider it against the rules to explain a result by making a convenient change in the theory, when the present theory has not been fully investigated. I say this because there has been speculation that a force of expansion, which would tend to accelerate galaxies as Hoyle wants, would result if the positive charges were not exactly equal to the negative charges. Since we have an excellent theory of electrodynamics in which e+ is precisely the opposite of e-, such a speculation seems useless. We are not going to overthrow a beautiful theory of electrodynamics to provide a mechanism for a kinematic model which might easily be found wrong as soon as we make accurate observations. It is still quite possible for Hoyle to be right but we shall find it out first of all from observations on the universe as it is.
Let me say also something that people who worry about mathematical proofs and inconsistencies seem not to know. There is no way of showing mathematically that a physical conclusion is wrong or inconsistent. All that can be shown is that the mathematical assumptions are wrong. If we find that certain mathematical assumptions lead to a logically inconsistent description of Nature, we change the assumptions, not Nature.
I say all this because I am not sure in which ways the theory of the Universe according to Hoyle may not quite coincide with many other assumptions we physicists ordinarily make. For example, there may be some trouble in the possibility of signalling which is so much a part of the thinking in relativistic theories. If a given galaxy has disappeared from view, is it really out of the universe, for us? Is it possible that we may ask a friend in the outer fringe how this galaxy is doing, while he is still in our view and the other galaxy is in his view? Or is there a general conspiracy of the detailed kinematics to preserve the relativistic rule, that a velocity near c in a coordinate system moving relative to us with a speed near c always yields a sum less than c?
Let us speak for a moment about the conservation of energy. In Hoyle's theory matter is created at rest at the "center" of the universe and we have said that there is no over-all energy creation because the negative gravitational energy just balances the rest mass energy. This kind of conservation of energy is that if we take a box of finite size anywhere, no matter appears inside except by an energy flow from the outside. In other words, only a law of local conservation is meaningful. If energy could disappear in one place and simultaneously reappear in some other place, without the flow of anything in between, we could deduce no physical consequences from the over-all "conservation." Let us therefore interpret Hoyle's creation of matter as follows. We imagine a finite universe having large masses distributed in a spherical shell as illustrated in Figure 13.2. We imagine pairs of particles of zero energy dropping in from the outside of the shell toward the inside. These we might think of as photons or gravitons or neutrinos, or maybe some new particles, some shmootrinos which don't worry about baryon conservation. When they meet another shmootrino dropping in from the other side with opposite momentum, these can have enough energy to create a hydrogen atom. In this way we can have both Hoyle's matter creation and a local energy conservation, since the matter is created from energy flowing in by the shmootrinos. If the flux of shmootrinos were very high, and the cross section for matter production be finite just above threshold, we can understand why matter should be created at rest relative to the average of the nebulae. The idea is that if the flux is high enough, as soon as a shmootrino has enough energy it will find another coming in the opposite direction and proceed to create matter; if the maximum energy which shmootrinos can acquire in falling is barely the same as the threshold, the matter will be created "at rest." In some such manner we can have simultaneously local energy conservation and Hoyle's theory. Of course, there are a great many remaining problems, such as we still have to consider why baryon number may not be conserved....