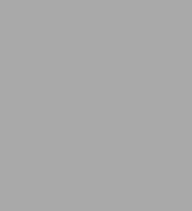
Evolution of the Igneous Rocks: Fiftieth Anniversary Perspectives
602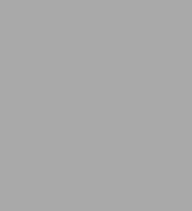
Evolution of the Igneous Rocks: Fiftieth Anniversary Perspectives
602Paperback(Anniversary)
-
PICK UP IN STORECheck Availability at Nearby Stores
Available within 2 business hours
Related collections and offers
Overview
Originally published in 1979.
The Princeton Legacy Library uses the latest print-on-demand technology to again make available previously out-of-print books from the distinguished backlist of Princeton University Press. These editions preserve the original texts of these important books while presenting them in durable paperback and hardcover editions. The goal of the Princeton Legacy Library is to vastly increase access to the rich scholarly heritage found in the thousands of books published by Princeton University Press since its founding in 1905.
Product Details
ISBN-13: | 9780691601953 |
---|---|
Publisher: | Princeton University Press |
Publication date: | 03/08/2015 |
Series: | Princeton Legacy Library , #1712 |
Edition description: | Anniversary |
Pages: | 602 |
Product dimensions: | 6.10(w) x 9.10(h) x 1.30(d) |
Read an Excerpt
The Evolution of the Igneous Rocks
Fiftieth Anniversary Perspectives
By Hatten Schuyler Yoder Jr.
PRINCETON UNIVERSITY PRESS
Copyright © 1979 Princeton University PressAll rights reserved.
ISBN: 978-0-691-08224-0
CHAPTER 1
THE PROBLEM OF THE DIVERSITY OF IGNEOUS ROCKS
G. MALCOLM BROWN
Department of Geological Sciences, University of Durham, Durham, England
The science of igneous petrogenesis is a study of the origin and evolution of rocks that have formed through the generation of magmas by melting processes, and the cooling of those magmas from a liquid, or liquid and crystalline state, into a glassy to crystalline state. Field and textural observations do not constitute sufficient evidence for defining a rock as igneous in origin (Barth, 1962, p. 51) but when allied with experimental studies on silicate melts similar in composition to the rock in question, the origin of the rock can be verified. Experimental studies, primarily by N. L. Bowen, formed the only firm basis from which the science of igneous petrogenesis could develop. If there were no diversity of igneous rocks, there would be no science of igneous petrogenesis. Large-volume flows of apparently homogeneous basalt are problematical, but only when viewed in relation to the more common type of diverse flow. The "problem" of the diversity of igneous rocks is more a matter of "problems" that will not be solved until all the complex processes of igneous petrogenesis have been understood. Bowen was as aware of that, in 1928, as one is now, and selected the topic of diversity for the opening chapter of his book. He stressed the importance of igneous rock associations and the need to explain differences in mineral assemblages and rock compositions, both between and within those associations, according to definite physicochemical processes. One should first consider, briefly, what type of diversity led Bowen to make the investigations that were described in the rest of his book, and then view the subject in the light of contemporary knowledge.
Bowen endorsed the concept of "petrographic provinces" (Judd, 1886), but pointed to the importance of time as well as place in such groupings and introduced "rock associations" as a preferable name. Such an association of rocks, sharing certain properties and connected by a "community of origin," was said to have been derived from a single original magma. He favored a basaltic magma in the parental role but did not think it was requisite in all cases. The question of whether more than one type of parental magma was involved did not tempt Bowen to provide an opinion. A division into alkaline and subalkaline types he thought useful, yet he did not quibble with R. A. Daly's view that there were no essential differences between the basaltic magmas of the various associations. At that time, Bowen was much more concerned with diversity produced within, rather than between rock associations. He stressed the continuous nature of variations in composition within a rock association, such that the division of such a series into specific members could only be arbitrary. Then followed his major discussion on the processes likely to be responsible for diversity within series, i.e., differentiation processes. He discounted compositional gradients in the liquid phase (temperature or gravity controlled), except insofar as pressure gradients could affect the concentration of volatile components. His support was entirely for processes involving separation of distinct phases either by gaseous transfer, liquid immiscibility, or crystallization. He went on to demonstrate the dominant role of fractional crystallization (associated sometimes with the additional effects of assimilation).
Petrologists would agree that there have been few major changes in emphasis since Bowen's exposition, regarding the general concept of igneous rock associations and the main processes responsible for variation within the associations. They would emphasize that their main concern is still with the diversity of what are now called primary, parental, and derivative magmas and their rock products, but particularly with the definition of what constitutes a primary magma. Since 1928 the subject has been expanded enormously through access to information on ocean-floor igneous rocks, and the theory of global plate tectonics has required a reappraisal of what are now called petrogenic provinces. The vast amounts of experimental data on multicomponent systems, referred to in subsequent chapters, have led to an expansion of knowledge about the deep regions of primary magma generation in the Earth's upper mantle, and about the processes of crystal fractionation, liquid immiscibility, and the role of volatile components in further promoting diversity in the patterns of magmatic evolution. Further advances relate to the application of thermodynamics to an understanding of the liquid state, rates of crystallization and nucleation, solid-liquid-gas equilibrium relations, and the effects of temperature and pressure on mineral equilibria. Extensive studies on the distribution of chemical elements and certain isotopes, and on element partitioning between crystalline and liquid phases, have been accompanied by consideration of the relative importance of fractional crystallization, fractional melting, and equilibrium partial melting in the derivation of particular natural silicate liquids (e.g., Gast, 1968; Shaw, 1970).
In order to recognize diversity in any population it is necessary first to define the criteria for distinctions and the limits of tolerance beyond which uniformity becomes diversity. Igneous rocks as a whole are uniform according to the criteria and broad limitations defined in the opening sentence of this chapter, and thus diverge from metamorphic and sedimentary rocks. The introduction of sets of criteria such as liquidus temperature, silica content, or locality within a specified area of the Earth's crust would lead to the recognition of diversity likely to have petrogenic significance, whereas criteria such as rock grainsize or precise age of crystallization would have less significance. As more is learned about field associations and the physicochemical processes governing the evolution of magmatic rocks, so one is better able to discard irrelevant distinguishing criteria and introduce more significant ones. Early geologists recognized a significance in the diverse chemical compositions of basalt, trachyte, and rhyolite. Later, chemical diversity even among basalts was recognized, initially only as tholeiitic and alkalic basalt magma types and chiefly according to SiO2 and Na2O + K2O contents (Bailey et al., 1924; Kennedy, 1933) but subsequently, according to quartz-orthopyroxene-olivine-nepheline normative ratios, as quartz tholeiite, tholeiite, olivine tholeiite, olivine basalt, and alkalic basalt (Yoder and Tilley, 1962). A further refinement in basalt classification has developed from the use of certain trace element and isotopic data. In the early days of ocean-floor exploration, the few dredged basalt samples from ocean-ridge regions were classed as uniform, olivine tholeiites rich in alumina (Engel, Engel, and Havens, 1965). They were called oceanic (or abyssal) tholeiites, but subsequent studies have shown that oceanic tholeiites are of two types (e.g., Schilling, Anderson, and Vogt, 1976), those from the ridges being more depleted in K, light rare-earth elements, and radiogenic Sr and Pb isotopes than those from shallow oceanic platforms such as the Azores, Hawaii, Iceland, and the Galapagos islands. Since Schilling (1975) has discovered chemical gradations between the two types along the axis of the Mid-Atlantic Ridge, one must recognize diversity even among oceanic (abyssal) tholeiites. The story of andesite is similar in that as more sophisticated criteria were applied, such rocks were subdivided into hawaiite of alkalic, icelandite of tholeiitic, and orogenic andesite of caic-alkaiic affinities. It was then customary to assume that island-arc andesites were all of calc-alkalic type and similar in composition and source, but along the axis of the Lesser Antilles arc, three chemically distinct types of andesite have been erupted over the past 3 million years, which show appreciable diversity in, for example, Ni, Cr, and K contents (Brown et al., 1977). The basalts of the Moon provide another example of how increasing diversity was recognized as more samples were collected and more sophisticated methods were applied during the mission program (summary in Taylor, 1975). Similar developments have taken place in the recognition of diversity among acidic and ultramafic rock suites.
Lest one falls into the trap of giving almost every rock a different name according to its major and trace element composition and locality, limits need to be drawn and the assumption made that in time, those limits will be redefined. One can only apply limits according to the present state of knowledge on the processes involved in igneous-rock evolution.
If the Earth has been formed by the homogeneous accretion of solar-nebula matter, or was homogenized by total melting of a heterogeneous accretion of such matter, then the starting mixture would have been uniform and probably close to a chondritic composition. Diversity began with the formation of a core and mantle. The two earliest Earth melts would thus have been metallic and ultramafic, so the first differentiation process was probably due to the immiscibility of metal-sulphide and silicate liquids. One still does not know how and when the Earth's primitive crust was formed, or what its composition was. It could perhaps have been andesitic (e.g., by sequential accretion to give a veneer of low-temperature, volatile-rich condensates; Larimer and Anders, 1967), ultramafic (by uniform crystallization from the surface downward), or a complex assemblage such as the anorthosites, norites, troctolites, and gabbros of the Moon's crust. On the Moon, the major process of fractional crystallization began shortly after formation, as evidenced by crystallization ages of 4.5 × 109 years for dunite and troctolite fragments (Papanastassiou and Wasserburg, 1976). On Earth, the only unmetamorphosed basic or ultrabasic igneous rocks that one can examine in any detail are not part of a primitive crust but are younger rocks related to the melting of a source in the Earth's upper mantle. Although some can be categorized as the products of "primary" magmas, no such magmas can be viewed as being chronologically "primitive." The younger the magma, the less likely it is to have been derived from mantle material unmodified by earlier melting events.
The starting composition, in a consideration of diversity from a common parentage, is best referred to as a primary magma. The source of primary magmas is universally accepted as the upper mantle, but because the source is not likely to be homogeneous in composition and because the pressure-temperature conditions of magma generation will vary, a range in the composition of primary magmas is to be expected. If the magma is truly primary, its composition will reflect the conditions of crystal-liquid equilibrium at the stage when the melt was initially removed from the residual crystalline phases at source. Source depths will vary according to the tectonic environment, as shown by various studies summarized by Yoder (1976, Ch. 3). These suggest that the source depths for most primary magmas are probably about 100 ± 50 km. It would seem unlikely that such magmas (e.g., basaltic) could reach high levels in the crust without becoming modified in composition by lower-pressure crystal fractionation. If this has occurred, then the basalt compositions will not reflect the conditions of primary generation. Such non-primary basalt magmas would, however, possess compositions such that on cooling they can give rise to derivative magmas, and in that sense they may be called parental magmas. Although, in the stricter sense, such basalts are derivative magmas, their role as parents to a wide range of lower-temperature derivative magmas within the crustal environment is far more significant.
If extensive olivine fractionation has occurred prior to basaltic magma eruption, then the primary magmas would have been picritic rather than basaltic in composition (O'Hara, 1968). Hence the only candidates for primary-magma products within the crust would be rocks such as komatiites (Viljoen and Viljoen, 1969). This view is not shared by Ringwood (1975), who advances arguments, chiefly based on nickel and chromium distribution in basalts, against extensive olivine fractionation during the ascent of basaltic magmas within the mantle. An extensive discussion of this critical problem is provided by Yoder (1976, Ch. 8), mainly by reference to melting studies on eclogites, two olivine tholeiites, and a nepheline-normative olivine basanite. In no case was olivine produced in the high-pressure mineral assemblages. This and other experimental evidence is advanced to favor the hypothesis that the compositions of basaltic magmas have been modified during ascent from their mantle source. Hence they are derivatives of the primary magmas. Yoder excludes from this category those alkalic basalts that have transported mantle nodules to the surface; such rapid ascent would imply the inability to fractionate smaller olivine crystals and hence imply a closer approach to a primary composition for the basalt host. He does not include reaction between nodules and the host basalts which could, during ascent to lower P-T conditions, have modified the primary basalt compositions slightly in certain cases.
The compositions of basalt magmas are such that they possess high liquidus temperatures relative to most other crustal magmas, and hence cooling and differentiation will give rise to a range of lower-temperature derivative magmas (e.g., Tilley, Yoder, and Schairer, 1963, 1965). The experimental evidence will be discussed in subsequent chapters. Field and analytical data indicate spatial and chemical relationships that support the experimental data, such as the basaltic chilled margins (and basaltic pore material in the basal layers) of strongly fractionated intrusions, and the relative abundance of those chemical elements, in basalts, that are depleted by crystal-liquid fractionation.
There is compositional diversity among basalt magmas, much of the variation being gradational. The distinctions are often subtle, and are more easily recognized by reference to the composition of the derivative magmas of each apparently basaltic parent. Consideration of such "igneous suites" (Harker, 1909), "magma series" (Bailey et al., 1924), or "rock associations" (Bowen, 1928) often shows that the derivative magmatic offspring possess chemical characteristics inherited from the parent magma (called consanguinity by Iddings, 1892). A subtle compositional difference between two basalts may imply a difference in the composition of the mantle source material (including variation in volatiles content), varying amounts of partial melting, variation in the degree of equilibrium versus fractional melting, or a different history of fractionation and reaction during ascent from the mantle source. A combination of several of these effects seems more likely than a single process in the generation of basalt-magma diversity. Experimental studies help in distinguishing between liquid-solid equilibria at varying pressures, but more information on the partitioning of trace elements at varying pressures would also be of value.
Tholeiitic and alkalic basalt magmas are generally recognized as major parental types. It is still difficult to decide whether specific olivine tholeiite, (alkali) olivine basalt, quartz tholeiite, and high-alumina basalt magmas are to be viewed as having a parental rather than derivative status, but olivine tholeiite in oceanic-ridge regions and the type of high-alumina tholeiitic basalt found in several island-arc regions appear to qualify. Ultrapotassic and nephelinitic magmas are difficult to attribute to a derivative origin in regions where alkalic basalts are rare or absent. Special conditions that gave rise to high thermal activity in localized belts during the Precambrian are advocated to explain the genesis of Archaean komatiite magmas (e.g., Nesbitt and Sun, 1976) and mid-Proterozoic, labradoritic anorthosite magmas (Bridgewater and Windley, 1973). This type of association of magma types with chronology, applicable also to the abundance of tholeiites and scarcity of basanites among Precambrian volcanics, needs consideration in petrogenic modeling. It may well indicate long-term changes in the thermal regimes and compositions at the mantle sources.
On a global scale, it is no longer logical to draw a sharp distinction between oceanic and continental magma provinces unless related to a particular period in the configuration of lithospheric plates. Subcontinental mantle will become suboceanic mantle through the effects of sea-floor spreading. The abyssal oceanic-ridge tholeiites, however, and the alkalic basalts of ocean-floor platforms and oceanic islands, belong to a definable petrogenic province where the environment is established at a particular period of tectonic evolution. Ultrapotassic and nephelinitic magmas are most abundantly associated with kimberlites and carbonatites where rifting of thick continental lithosphere has disturbed an environment in which continental stability has persisted for a long time (e.g., East Africa), but oceanic islands may contain potassic rocks (Tristan da Cunha) and nephelinitic rocks (Hawaii). Some continental tholeiites have probably suffered from contamination by sialic crust, but those generated during ancient lithospheric rifting need not have been affected, so the observed spectrum of compositions, in terms of dispersed elements and 87Sr/86Sr, is to be expected. Alkalic basalts may occur in a similar continental environment (such as the Scottish Tertiary Province). The basalts of island arcs may range from nepheline-normative basanites to quartz-normative tholeiites within a short period of arc evolution. Arcs such as the Lesser Antilles, formed along a north-south zone between plates of oceanic lithosphere to the west and east, are not to be viewed as the products of a continental environment.
(Continues...)
Excerpted from The Evolution of the Igneous Rocks by Hatten Schuyler Yoder Jr.. Copyright © 1979 Princeton University Press. Excerpted by permission of PRINCETON UNIVERSITY PRESS.
All rights reserved. No part of this excerpt may be reproduced or reprinted without permission in writing from the publisher.
Excerpts are provided by Dial-A-Book Inc. solely for the personal use of visitors to this web site.
Table of Contents
- Frontmatter, pg. i
- CONTENTS, pg. vii
- PREFACE, pg. xi
- Chapter 1. THE PROBLEM OF THE DIVERSITY OF IGNEOUS ROCKS, pg. 1
- Chapter 2. SILICATE LIQUID IMMISCIBILITY IN MAGMAS, pg. 15
- Chapter 3. FRACTIONAL CRYSTALLIZATION AND PARTIAL FUSION, pg. 59
- Chapter 4. CRYSTALLIZATION IN SILICATE SYSTEMS, pg. 77
- Chapter 5. THE REACTION PRINCIPLE, pg. 133
- Chapter 6. FRACTIONAL CRYSTALLIZATION OF BASALTIC MAGMA, pg. 171
- Chapter 7. THE LIQUID LINE OF DESCENT AND VARIATION DIAGRAMS, pg. 205
- Chapter 8. GLASS AND THE GLASSY ROCKS, pg. 233
- Chapter 9. ROCKS WHOSE COMPOSITION IS DETERMINED BY CRYSTAL ACCUMULATION AND SORTING, pg. 245
- Chapter 10. EFFECTS OF ASSIMILATION, pg. 307
- Chapter 11. THE FORMATION OF SILICEOUS POTASSIC GLASSY ROCKS, pg. 339
- Chapter 12. THE FELDSPATHOIDAL ALKALINE ROCKS, pg. 351
- Chapter 13. MELILITE-BEARING ROCKS AND RELATED LAMPROPHYRES, pg. 391
- Chapter 14. THE FRACTIONAL RESORPTION OF COMPLEX MINERALS AND THE FORMATION OF STRONGLY FEMIC ALKALINE ROCKS, pg. 413
- Chapter 15. FURTHER EFFECTS OF FRACTIONAL RESORPTION, pg. 423
- Chapter 16. THE IMPORTANCE OF VOLATILE CONSTITUENTS, pg. 439
- Chapter 17. PETROGENESIS AND THE PHYSICS OF THE EARTH, pg. 483
- Chapter 18. PARTITIONING BY DISCRIMINANT ANALYSIS: A MEASURE OF CONSISTENCY IN THE NOMENCLATURE AND CLASSIFICATION OF VOLCANIC ROCKS, pg. 521
- AUTHOR INDEX, pg. 533
- SUBJECT INDEX, pg. 544
- SYSTEMS INDEX, pg. 586