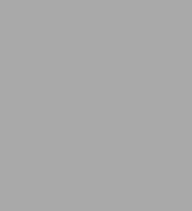
Conservation Paleobiology: Science and Practice
336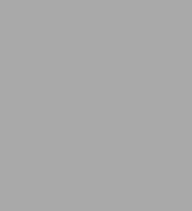
Conservation Paleobiology: Science and Practice
336eBook
Available on Compatible NOOK devices, the free NOOK App and in My Digital Library.
Related collections and offers
Overview
Studying endangered species is difficult. They are by definition rare, some exist only in captivity, and for those still in their native habitats any experimentation can potentially have a negative effect on survival. Moreover, a lack of long-term data makes it challenging to anticipate biotic responses to environmental conditions that are outside of our immediate experience. But in the fossil and prefossil records—from natural accumulations such as reefs, shell beds, and caves to human-made deposits like kitchen middens and archaeological sites—enlightening parallels to the Anthropocene can be found that might serve as a primer for present-day predicaments. Offering both deep-time and near-time perspectives and exploring a range of ecological and evolutionary dynamics and taxa from terrestrial as well as aquatic habitats, Conservation Paleobiology is a sterling demonstration of how the past can be used to manage for the future, giving new hope for the creation and implementation of successful conservation programs.
Product Details
ISBN-13: | 9780226506869 |
---|---|
Publisher: | University of Chicago Press |
Publication date: | 11/17/2017 |
Sold by: | Barnes & Noble |
Format: | eBook |
Pages: | 336 |
File size: | 19 MB |
Note: | This product may take a few minutes to download. |
About the Author
Read an Excerpt
CHAPTER 1
THE YOUNGEST FOSSIL RECORD AND CONSERVATION BIOLOGY: HOLOCENE SHELLS AS ECO-ENVIRONMENTAL RECORDERS
MICHAL KOWALEWSKI
Department of Geosciences, Virginia Tech, Blacksburg, VA 24061 USA
*
ABSTRACT. — This chapter reviews eco-environmental information that can be extracted from the youngest (surficial) fossil record. The main focus of this review are shell-producing macro-invertebrates, which are abundant at (or near) the sediment surface in many aquatic and terrestrial habitats. As demonstrated by geochronologic and sclerochronologic analyses, such Holocene shell accumulations provide direct, often continuous, records of the most recent centuries and millennia. They offer us a broad spectrum of data, including multiple taxonomic, ecologic, taphonomic, geochronologic, and geochemical parameters that can inform us about the recent history of organisms, communities, ecosystems, and environments. Eco-environmental analyses of shells and other bio-remains provide us thus with a long-term historical perspective; a quantitative baseline for understanding pre-industrial ecosystems, evaluating anthropogenic changes, and assessing restoration efforts. This research strategy, sometimes referred to as "conservation paleobiology," is often based on dead remains only, and consequently, represents a biologically non-invasive approach to conservation issues. The recently dead — the youngest fossil record of environmental and ecological processes at local, regional, and global scales — can help us to protect the living and should allow us to manage the future of our biosphere more effectively.
*
INTRODUCTION
SKELETAL REMAINS of dead organisms are copious in many modern environments: trillions of mollusk shells contour ocean shorelines, coastal dunes are streaked with dense layers of land snail conchs, and countless mussel valves litter streams and rivers. Even dolphin bones — a valuable collector's item and a non-exportable national commodity — may occur in large numbers in more remote areas (e.g., Liebig et al., 2003) yet undiscovered by beachcombers.
A few examples of surficial accumulations highlighted here on Figure 1 illustrate their exceptional qualities. Among others, these accumulations represent biomineralized remains that are easy to access and do not require expensive sampling machinery (Fig. 1.1, 1.2, 1.4, 1.5, and 1.6). Also, they often provide us with copious quantities of specimens that can be sampled from a single spot (e.g., Fig. 1.2, 1.3, 1.6, and 1.7), and the material is typically preserved exceedingly well; for example, articulated skeletons of vertebrates (Fig. 1.1) or ligament-preserving shells of freshwater mussels (Fig. 1.4) are not uncommon. Finally, these accumulations can be found in a broad spectrum of environmental and depositional settings — from eolian dunes (Fig. 1.5 and 1.6), through river banks (Fig. 1.4), down to various marine habitats, from supratidal mud flats (Fig. 1.1) to open-shelf subtidal seafloors (Fig. 1.7).
To be sure, the wanton abundance of bioskeletal remains has been appreciated since the dawn of science. Starting with Aristotle and da Vinci, biologists and geologists have paid continuing attention to those surficial graveyards. However, only recently, have we begun to appreciate in full the wealth of historical data contained within shells, tests, bones, and other bio-remains scattered on the surface of our planet. This is for two reasons. First, geochronologic and sclerochronologic efforts of the last decades have demonstrated, repeatedly, that the youngest fossils yield continuous, multi-centennial to multi-millennia records of the most recent geological past (e.g., Flessa et al., 1993; Lazareth et al., 2000; Kowalewski et al., 2000; Carroll et al., 2003; Kidwell et al., 2005; Schöne et al., 2005; Kosnik et al., 2007; Yanes et al., 2007). Second, rapid advances in analytical instrumentation have provided us with increasingly diverse, sophisticated, and affordable techniques for dating, analyzing, and interpreting bio-remains left behind by dead organisms (e.g., Dettman and Lohmann, 1995; Kaufman and Manley, 1998; Riciputi et al., 1998; Lazareth et al., 2000; Rodland et al., 2003; Fiebig et al., 2005; Carroll et al., 2006; Fox et al., 2007; Schiffbauer and Xiao, in press). These and related analytical and conceptual advancements make it now possible to use the dead to help the living. That is, skeletal remains offer a quantifiable reference baseline that can help us to understand the living communities, assess the efficacy of restoration efforts, and, ultimately, protect their future. Just as important, this "Conservation Paleobiology" approach — as some refer to it nowadays (e.g., Flessa, 2002) — has a virtue of being partly or completely non-invasive. This is because conservation paleobiology involves sampling efforts that often do not require killing, or even disturbing notably, living biota.
Here, I review briefly eco-environmental information that can be extracted from the youngest (surficial) fossil record, with particular focus on shell-producing macro-invertebrates, although other types of macroscopic remains (bones, plants, etc.) will be discussed briefly when relevant. However, micropaleontological approaches to conservation paleobiology (subfossil pollen, test of plankton, etc.) are not discussed in this chapter. In addition, multiple case studies will be used to illustrate ways in which skeletal remains can be employed to augment our understanding of eco-environmental changes over centennial-to-millennial time scales. The main goal here is to highlight the unique historical potential of the data that can be provided by the youngest fossil record (e.g., Kowalewski et al., 2000; Jackson et al., 2001; Brown et al., 2005; Kidwell, 2007). After all, the historical perspective that reaches back in time far beyond the industrial revolution is necessary for the realistic assessment and effective mitigation of widespread anthropogenic changes occurring on our planet today (see especially Jackson et al., 2001; Pandolfiet al., 2003; Flessa et al., 2005; Flessa and Jackson, 2005).
A comment on citations cited in this chapter
Scientists care a great deal about fair and comprehensive accounting of previous work, as attested by the increasing importance of the Science Citation Index and the ever increasing emphasis that peer-review journals place on adequate referencing. Therefore, it is appropriate to comment on how cited references were selected here. Many of the thematic areas discussed below (e.g., sclerochronology, amino-acid dating, taphonomy, and stable isotopes) represent active research directions with dozens, or hundreds, of new publications appearing every year. It would be arguably suitable to cite tens of papers in each sentence of the text below. Regrettably, this is not feasible. The citations used here are, therefore, an inevitably biased, subjective, and unrepresentative sample of the existing literature. This caveat is trivial, but necessary, given goals of short course publications such as this one. An effort has been made to select references that either provide synthetic and well-referenced overviews of topics, or are directly pertinent to the theme of this chapter, or represent up-to-date examples of some relevance. Nevertheless, the reader should appreciate the fact that the references cited here are neither free of the author's idiosyncratic parochialisms (e.g., ignorance, subjective preferences, inherent self-centeredness, etc.) nor satisfactorily representative of the existing literature. Those who use short course volumes such as this one to augment their research should bear in mind that this caveat likely applies to most chapters of the type presented here and in other such publications.
Fossils, "fossils", or subfossils?
It is fair to notice that Holocene fossils are not really fossils sensu stricto, being from the epoch that is geologically synonymous with the Recent. Some authors refer to such bioskeletal remains as "subfossils", some use "fossils" with quotation marks, and some use the term "fossil" without quotation marks. In my view, from all practical standpoints (taphonomic, logistic, methodological, etc.), Holocene bioskeletal remains do not differ from older fossils. Moreover, it makes little sense to call a specimen dated at 9,000 yrs BP a subfossil or "fossil", while calling another specimen from the same sample a true fossil, just because its radiocarbon date came back from the lab dated at 12,500 yrs BP. Here, for the sake of expedience and clarity, I will follow the simplest approach and refer to all specimens (Holocene or not) as fossils (without quotation marks). Terminological purists have every right to vehemently disagree with this terminological flippancy.
MAJOR TYPES OF DATA RECOVERABLE FROM SKELETAL REMAINS
With the right set of tools, diverse data can be obtained from skeletal remains. In this section, 9 types of data retrievable from shells, bones, and other biomineral debris are summarized briefly. This list is certainly not exhaustive, but rather serves to highlight the remarkable plethora of parameters and variables preserved in the youngest fossil record. These variables can be organized as follows (although this scheme is arbitrary as numerous ways of organizing those data types may be possible).
Intrinsic biological variables
These include shell parameters that relate directly to biological characteristics of skeletal remains. The four variables most commonly extracted include the following:
1. Taxonomic identity. — With few exceptions, geologically recent (late Pleistocene to Holocene) skeletal remains represent extant genera and species. This makes species identification possible for most specimens. The bulk sampling of skeletal remains can therefore provide quantitative datasets about taxonomic diversity and relative abundance of species. From the conservation biology perspective, this type of data can be used to carry out a fidelity analysis, in which skeletal remains ("death assemblage") are compared to sympatric living communities ("life assemblage"). This approach is used increasingly to detect recent anthropogenic changes in local communities (e.g., Greenstein et al., 1998; Simões et al., 2005; Kidwell, 2007; Terry, 2007; Ferguson and Miller, 2007; Ferguson, 2008).
2. Ecological characteristics. — Because most skeletal remains belong to organisms that are still around, ecological characteristics of those dead organisms can also be inferred with high accuracy. Thus, skeletal remains can provide information about relative abundance of various ecological groups, with specimens grouped by mode of life, feeding type, or even preferred bathymetry expressed in meters (e.g., Scarponi and Kowalewski, 2004). Absolute abundance of skeletal remains of a given species may also carry ecological information about population dynamics, bioproductivity, or intensity of trophic interactions in pre-industrial times (e.g., Walbran et al., 1989a, b; Kowalewski et al., 2000; see also later in the text).
3. Size/age/biomass. — Skeletal remains (unless too fragmented) can also often provide reasonably accurate estimates of size at the time of death. In many cases, this information can be used to estimate the ontogenetic age of the specimen, its biovolume, or its soft-tissue biomass (e.g., Staff et al., 1985; Zuschin et al., 1999; Novack-Gottshall, 2008; see also Kosnik et al., 2006; Krause et al., 2007).
4. Sclerochronology and growth patterns. — In organisms that secrete skeletal elements via incremental accretionary processes, growth rings or other comparable time-sequential structures can be often recognized and analyzed. Because rates of growth are influenced by environmental variables such as temperature, nutrient levels, and other parameters (e.g., Kennish and Olsson, 1975; Sato, 1997; Schöne et al., 2003a, 2005; and numerous references therein), statistical analyses of growth increments can not only provide geochronological estimates, but also inform us about past environmental conditions. This approach is particularly powerful when used in conjunction with geochemical data (e.g., Goodwin et al., 2001; Ivany et al., 2003; multiple papers in Schöne and Surge, 2005). In some organisms, growth lines can offer daily or even sub-daily resolution (e.g., Berry and Barker, 1968; see also Rodland et al., 2006a), which is particularly remarkable when considering that some long-lived shellfish, including both marine and freshwater forms (e.g., Schöne et al., 2004, 2005; Dunca et al., 2005) can live (and grow intermittently or continuously) for decades or even centuries (see also below).
Secondary biological variables
These are mostly represented by trace fossils and skeletonized encrusters that provide direct or indirect records left on skeletal remains of shell-producing organisms. Two major types of records can be distinguished:
1. Syn-vivo records. — These are various traces and structures left by other organisms that interacted with the original owner of a given skeletal remain. Repair scars on skeletal remains recording failed predatory attacks (e.g., Vermeij et al., 1981; Vermeij, 1987; Cadeé et al., 1997; Alexander and Dietl, 2003; Voight and Sigward, 2007), drill holes made by carnivorous snails in shells of their prey (e.g., Carriker, 1981; Kitchell et al., 1981; Vermeij, 1983, 1987; Kitchell, 1986; Kelley and Hansen, 1993, 2003; Kowalewski, 2002; Leighton, 2002; Alexander and Dietl, 2001; Dietl and Herbert, 2005; Huntley and Kowalewski, 2007; Casey and Chattopadhyay, 2008; Schiffbauer et al., 2008), or syn-vivo domicile borings left by polychaetes (e.g., Rodrigues, 2007; Rodrigues et al., 2008; see also Martinell and Domènech, 2009) are all examples of ecological interactions recorded on skeletal remains of prey/host organisms. These records make it possible, for example, to assess trophic importance of prey organisms that produced those skeletal remains (e.g., Cintra-Buenostro et al., 2005; Stempien, 2005, 2007), estimate seasonal changes in intensity of predator-prey interactions (e.g., Kowalewski and Flessa, 2000), or evaluate predation pressures across environmental gradients (e.g., Dietl and Alexander, 2009).
2. Post-mortem records. — Organisms that colonize or mine skeletal remains of other organisms can produce various traces and biomineral structures that can be identified and interpreted. They can occur postmortem (i.e., after the substrate-producing shell owner died), although some may also represent syn-vivo interactions. These records include diverse variety of encrustation structures left by biomineralizing organisms that colonize skeletons of other organisms (e.g., Lescinsky, 1997; Taylor and Wilson, 2003; Rodland et al., 2004, 2006b) and macroscopic and microscopic traces made by bioeroding organisms (e.g., Bromley et al., 1990; Cutler, 1995; Bromley, 1996; Walker et al., 1998; Taylor and Wilson, 2003). Encrusting and bioeroding organisms are sensitive to various environmental parameters and, as some argue, may be useful for assessing bioproductivity and eutrophication (e.g., Lescinsky, 1997; Holmes et al., 2000; Lescinsky et al., 2002).
(Continues…)
Excerpted from "Conservation Paleobiology"
by .
Copyright © 2017 The Paleontological Society.
Excerpted by permission of The University of Chicago Press.
All rights reserved. No part of this excerpt may be reproduced or reprinted without permission in writing from the publisher.
Excerpts are provided by Dial-A-Book Inc. solely for the personal use of visitors to this web site.
Table of Contents
Foreword by Thomas E. Lovejoy
Introduction
Section One: Conservation Paleobiology in Near Time
1. The youngest fossil record and conservation biology: Holocene shells as eco-environmental recorders
Michał Kowalewski
2. Conservation biology and environmental change: A paleolimnological perspective
John P. Smol
3. Vertebrate fossils and the future of conservation biology
Elizabeth A. Hadly and Anthony D. Barnosky
4. Paleoecology and resource management in a dynamic landscape: Case studies from the Rocky Mountain headwaters
Stephen T. Jackson, Stephen T. Gray, and Bryan Shuman
5. Historical ecology for the paleontologist
Jeremy B. C. Jackson and Loren McClenachan
6. The isotopic ecology of fossil vertebrates and conservation paleobiology
Paul L. Koch, Kena Fox-Dobbs, and Seth D. Newsome
7. Evaluating human modification of shallow marine ecosystems: Mismatch in composition of molluscan living and time-averaged death assemblages
Susan M. Kidwell
8. Using a macroecological approach to the fossil record to help inform conservation biology
S. Kathleen Lyons and Peter J. Wagner
Section Two: Conservation Paleobiology in Deep Time
9. Seven variations on a recent theme of conservation
Geerat J. Vermeij
10. Metaphor, inference, and prediction in paleoecology: Climate change and the Antarctic bottom fauna
Richard B. Aronson
11. Ecological modeling of paleocommunity food webs
Peter D. Roopnarine
12. Paleobiology and the conservation of the evolving web of life
Gregory P. Dietl
13. Speciation and shifting baselines: Prospects for reciprocal illumination between evolutionary paleobiology and conservation biology
Warren D. Allmon
Section Three: Conservation Paleobiology at Work
14. Putting the dead to work: Translational paleoecology
Karl W. Flessa
15. Conservation paleobiology roundtable: From promise to application
Alison G. Boyer, Mark Brenner, David A. Burney, John M. Pandolfi, Michael Savarese, Gregory P. Dietl, and Karl W. Flessa
Epilogue: Conservation Paleobiology in the Anthropocene
Contributors
Acknowledgments
Index