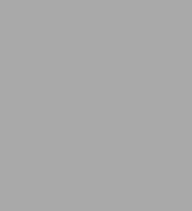
Computational Strong-Field Quantum Dynamics: Intense Light-Matter Interactions
290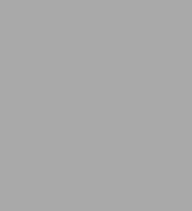
Computational Strong-Field Quantum Dynamics: Intense Light-Matter Interactions
290Paperback
-
PICK UP IN STORECheck Availability at Nearby Stores
Available within 2 business hours
Related collections and offers
Overview
Contents
How to propagate a wavefunction?
Calculation of typical strong-field observables
Time-dependent relativistic wave equations: Numerics of the Dirac and the
Klein-Gordon equation
Time-dependent density functional theory
The multiconfiguration time-dependent Hartree-Fock method
Time-dependent configuration interaction singles
Strong-field approximation and quantum orbits
Microscopic particle-in-cell approach
Product Details
ISBN-13: | 9783110417258 |
---|---|
Publisher: | De Gruyter |
Publication date: | 04/24/2017 |
Series: | De Gruyter Textbook |
Pages: | 290 |
Product dimensions: | 6.69(w) x 9.45(h) x (d) |
Age Range: | 18 Years |
About the Author
Table of Contents
Preface ix
List of abbreviations xi
I How to propagate a wavefunction? Dieter Bauer Thomas Fennel 1
1 Time-dependent Schrödinger equation 2
1.1 Time propagation and stability 3
1.2 Spatial discretization 3
1.3 Imaginary-time propagation 10
1.4 More dimensions: Operator splitting 13
1.5 Expansion in spherical harmonics 18
2 Scaled cylindrical coordinates 36
3 Employing second-quantization notion 38
3.1 Grid hopping 40
4 Summary 43
II Calculation of typical strong-field observables Dieter Bauer 45
1 Ionization rates 45
2 Photoelectron spectra 48
2.1 Energy window operator method 50
2.2 Spectral method 56
2.3 Time-dependent surface flux method 64
2.4 Pros and cons of the various methods for photoelectron spectra 68
3 Emitted radiation and high-harmonics spectra 69
III Time-dependent relativistic wave equations: Numerics of the Dirac and the Klein-Gordon equation Heiko Bauke 77
1 From nonrelativistic to relativistic quantum mechanics 77
1.1 Relativistic quantum mechanical equations of motion-a naive attempt 77
1.2 The Klein-Gordon equation 79
1.3 The Dirac equation 82
2 Free particles and wave packets 85
2.1 Free-particle solution of the Klein-Gordon equation 85
2.2 Free-particle solution of the Dirac equation 88
3 Numerical solution of the Dirac equation 93
3.1 General methods for time-dependent quantum mechanics 93
3.2 The split operator method 97
3.3 The Fourier split operator method for the Schrödinger equation 99
3.4 The Fourier split operator method for the Dirac equation 101
4 Numerical examples 104
IV Time-dependent density functional theory Dieter Bauer 111
1 A few general remarks on time-dependent many-particle methods 112
2 DFT for effective single-electron potentials 114
2.1 KS spin-DFT 114
2.2 Actual implementation 121
3 Time-dependent calculations 128
3.1 Time-dependent KS solver with spherical harmonics and multipole expansion 129
3.2 Low-dimensional benchmark studies 134
3.3 Where TDDFT fails in practice 140
V The multiconfiguration time-dependent Hartree-Fock method Chris R. McDonald Gisela Poplau Thomas Brabec 145
1 Multiconfiguration time-dependent Hartree-Fock 146
2 Implementing the MCTDHF method 149
2.1 Uniform grids 149
2.2 Computation of the mean-field operator 150
2.3 Restricted vs unrestricted 151
2.4 Time integration 152
2.5 Computing the ground state 153
3 Applications of MCTDHF 153
3.1 Calculation of highly correlated ground states 153
3.2 Nonsequential double ionization 155
3.3 High-harmonic generation 157
4 Extending MCTDHF to nonuniform grids 158
4.1 Differentiation on a nonuniform grid 159
4.2 Integration on nonuniform grids 160
4.3 Treatment of the two-body terms 162
4.4 Ground state of small sodium clusters 163
5 Conclusion 165
VI Time-dependent configuration interaction singles Stefan Pabst Robin Santra 169
1 Introduction 169
2 Basics of TDCIS 171
2.1 TDCIS wavefunction 171
2.2 The N-body Hamiltonian 173
2.3 Equations of motion 175
2.4 Limitations 176
3 Implementation of TDCIS 177
3.1 Symmetries and orbital representations 177
3.2 Evaluating matrix elements 179
3.3 Spin-orbit interaction 181
3.4 Grid representation 183
3.5 Hartree-Fock 184
3.6 Complex absorbing potential 186
3.7 Expectation values 187
3.8 Ion density matrix 188
4 Strong-field applications of TDCIS 189
4.1 Subcycle ionization dynamics and coherent hole motion 189
4.2 Multiorbital and collective excitations in HHG 195
VII Strong-field approximation and quantum orbits Dejan B. MiloSevic 203
1 S-matrix elements 204
2 Strong-field approximation 206
3 Harmonic generation rate and ionization rate 208
4 Ground-state wavefunctions, rescattering potential, and multielectron effects 209
5 Numerical examples for harmonic and electron spectra 211
6 Saddle-point method 214
7 Classification of the saddle-point solutions 216
8 Numerical results for HATI spectra obtained using the SPM and uniform approximation 218
9 Quantum orbits 220
10 Summary 222
VIII Microscopic particle-in-cell approach Christian Peltz Charles Varin Thomas Brabec Thomas Fennel 227
1 Basic concept 228
1.1 Physical problem 228
1.2 Particle representation 229
1.3 PIC approximation 230
1.4 MicPIC force decomposition 230
1.5 The MicPIC approximation 232
2 Numerical aspects of MicPIC 233
2.1 Electromagnetic field propagation with the FDTD method 233
2.2 Particle representation on the PIC level 244
2.3 Local correction 247
2.4 Particle propagation 250
2.5 Implementation of ionization 252
2.6 MicPIC parameters and scaling 254
2.7 MicPIC system energy calculation 256
3 Applications 256
3.1 Laser excitation of a solid-density foil: A simple MicPIC example 256
3.2 Time-resolved x-ray imaging 259
4 Summary 267
Index 271