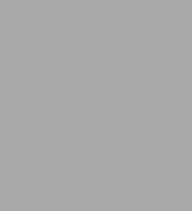
Complementary Strategies to Study Virus Structure and Function
349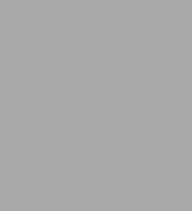
Complementary Strategies to Study Virus Structure and Function
349eBook
Available on Compatible NOOK devices, the free NOOK App and in My Digital Library.
Related collections and offers
Overview
Complementary Strategies to Study Virus Structure and Function, Volume 104, the latest release in the Advances in Virus Research series, highlights new advances in the field, with this new volume presenting interesting chapters on X-ray structures from crystals of viral proteins grown in cellula, NMR and SAXS to study protein dynamics and natively disordered viral proteins, Mass spectrometry to study virus particle assembly, Atomic force microscopy to study virus particles, Non-enveloped viruses and interactions with antibodies, Non-enveloped viruses and their mechanism of entry into cells, Structures of enveloped virions by electron cryo-microscopy and cryo-tomography, and many other interesting topics.
- Provides the authority and expertise of leading contributors from an international board of authors
- Presents the latest release in the Advances in Virus Research series
- Includes the latest information on virus structure and function
Product Details
ISBN-13: | 9780128184578 |
---|---|
Publisher: | Elsevier Science |
Publication date: | 09/13/2019 |
Series: | ISSN |
Sold by: | Barnes & Noble |
Format: | eBook |
Pages: | 349 |
File size: | 52 MB |
Note: | This product may take a few minutes to download. |
About the Author
Read an Excerpt
CHAPTER 1
The viral replication organelles within cells studied by electron microscopy
Martin Sachse, Isabel Fernández de Castro, Raquel Tenorio, Cristina Risco
Contents
1. Introduction 2
2. Live cell imaging 5
3. General aspects of sample preparation for transmission electron microscopy 7
4. Correlative light and electron microscopy 9
5. High-pressure freezing and freeze-substitution 12
6. Frozen-hydrated sections: Cemovis and cryo-lamella 15
7. Immunogold labeling 16
8. 3D imaging/tomography 19
9. Clonable tags for electron microscopy 22
10. Conclusions and future perspectives 24
Acknowledgments 25
References 25
Abstract
Transmission electron microscopy (TEM) has been crucial to study viral infections. As a result of recent advances in light and electron microscopy, we are starting to be aware of the variety of structures that viruses assemble inside cells. Viruses often remodel cellular compartments to build their replication factories. Remarkably, viruses are also able to induce new membranes and new organelles. Here we revise the most relevant imaging technologies to study the biogenesis of viral replication organelles. Live cell microscopy, correlative light and electron microscopy, cryo-TEM, and three-dimensional imaging methods are unveiling how viruses manipulate cell organization. In particular, methods for molecular mapping in situ in two and three dimensions are revealing how macromolecular complexes build functional replication complexes inside infected cells. The combination of all these imaging approaches is uncovering the viral life cycle events with a detail never seen before.
Abbreviations
CEMOVIS cryo-electron microscopy of vitreous sections
CLEM correlative light and electron microscopy
EM electron microscopy
ET electron tomography
FIB focused-ion beam
FS freeze-substitution
GFP green fluorescence protein
HPF high-pressure freezing
LM light microscopy
METTEM metal-tagging TEM
MT metallothionein
RO replication organelle
RT room temperature
SEM scanning electron microscopy
STEM scanning-transmission electron microscopy
3D three-dimensional
TEM transmission electron microscopy
2D two-dimensional
VF virus factory
VRC viral replication complex
vRNP viral ribonucleoprotein
Virus names
BUNV Bunyamwera virus
DENV dengue virus
FHV flock house virus
FMDV foot-and-mouth disease virus
HCV hepatitis C virus
HIV human immunodeficiency virus
SARS CoV severe acute respiratory syndrome coronavirus
SFV Semliki forest virus
TBSV tomato bushy stunt virus
VV vaccinia virus
1. Introduction
The common hallmark of all viruses is their dependency on a host cell to infect and multiply. During this cycle the virus utilizes and transforms the machinery of the cell for the biosynthesis of the own genetic material, proteins, and lipids. This can lead to vast reorganization in the subcellular organization inside the cell (Risco et al., 2014; Romero-Brey and Bartenschlager, 2014). The size of most viruses is beyond the resolution limit of optical microscopy, and only electron microscopy (EM) provides the resolution required to study viruses and their life cycles (Pelchen-Matthews and Marsh, 2007).
The technical specifications of an electron microscope are, however, only one part of the equation to deliver insight into the viral life cycle within the host cell. The first obstacle comes from the dynamic nature of the virus cycle. Infection, replication, virus particle morphogenesis and egress are different, consecutive steps. But in contrast to optical microscopy, EM does not permit the observation of the same object over time. This aspect can be solved with the study of different samples after finding the right time post-infection for each step. Next to the dynamics of these different steps during viral infection, another challenge arises from their topology: only entry and release of the viral particle take place at the surface of the host cell. All other steps utilize machineries inside the cell and their study requires the analysis of the morphology of the cell interior. For this reason, transmission electron microscopy (TEM) combined with thin sections of infected cells has been for a long time the standard approach to study the intracellular aspects of virus life cycles. However, the morphology of a cell is three-dimensional and thus random thin sections provide a limited view. As pointed out more than 60 years ago, the impact of EM for the morphological analysis of viruses depends highly on technological progress on sample preparation (Hillier, 1950). Since this time, sample preparation as well as the sensitivity of electron microscopes and their detectors has evolved to a great extent and different approaches for tomography were developed to study samples in 3D. But the key point raised at that time has remained: each sample preparation/imaging technique produces artifacts and it is important to be aware of them and be critical about the amount of information that can be extracted. Simultaneously with the technological development, our knowledge about the life cycle of viruses has increased, and the diversity of the questions addressed has increased concomitantly. It is often required to combine different approaches to answer the question at hand. Therefore, this review will focus on the different preparation and imaging techniques that are used to visualize the cell biology of viral infections (Fig. 1). We will discuss the technological basis of the different approaches and the findings they made possible. In particular, we will focus on the characterization of virus factories and virus replication organelles. Viruses express their fundamental biological properties during the intracellular stage of their life cycle, which in most cases takes place within virus factories (Fernández de Castro et al., 2013). Viral factories (VF) are intracellular membranous compartments harboring viral replication organelles (RO) that contain viral replication complexes (VRC) and the sites of virus particle assembly (Harak and Lohmann, 2015; Novoa et al., 2005). VRCs are often located in single-membrane vesicles known as spherules, but also in double-membrane vesicles (DMVs), flat membranes and cubic/convoluted membranes (CM) (Fernández de Castro et al., 2013). To build these neoorganelles, viruses manipulate lipid synthesis and flows to create platforms with particular lipid composition and biophysical properties that facilitate viral genome replication (Altan-Bonnet, 2017; Fernández de Castro et al., 2016; Fernández-Oliva et al., 2019). Due to their complex composition and functions, virus factories could be contemplated as the living state of viruses (Fridmann-Sirkis et al., 2016). Two main pathways have been identified so far as being responsible for viral factory biogenesis: aggresome formation for DNA viruses and autophagy for RNA viruses (Abernathy et al., 2019; Jackson et al., 2005; Kajitani et al., 2013; Wileman, 2007).
Mitochondria and cytoskeleton elements are often found around replication organelles building a large structure that usually transforms the whole cell content (Fernández de Castro et al., 2013). Viral factories are dynamic structures that change over time and maintain communication with intracellular transport pathways such as endocytosis and exocytosis, which are necessary in order to incorporate essential factors into the factories and to facilitate virus egress (Altan-Bonnet, 2017; Fontana et al., 2010). Some viruses build factories inside the nucleus (Erickson et al., 2012) but most of the structural information available to date comes from cytoplasmic viral factories (Risco et al., 2014).
2. Live cell imaging
Despite the focus on EM of this review we must highlight light microscopy (LM) as a great ally of electron microscopists. This is due to the ability of LM to characterize dynamic changes in the cell associated to viral infection, albeit with less resolution but with shorter times for sample preparation. Furthermore, new advances in fluorescent probes and LM methods are providing new insights into the cell biology of viruses. Very interesting recent reviews deal with light microscopy of viral infections (Alonas et al., 2016; Dirk et al., 2016; Hanne et al., 2016; Witte et al., 2018). However, microscopy of fixed cells provides static views of complex, dynamic events. To study multi-step processes such as a virus infection, time-course assays are useful and provide data that we try to organize sequentially. Nevertheless, during viral infections what we perceive as a logical sequence of events might not correspond to reality, and many findings would have never been uncovered without dynamic, real time, live cell microscopy. Live-cell imaging of viral infections has been mainly focused on processes of entry and release of viral particles (Baktash et al., 2018; Francis and Melikyan, 2018; Jouvenet et al., 2008) but has shown great potential to study all steps of the virus replication cycle (Campbell and Hope, 2008). Live cell microscopy can also reveal a heterogeneous response of cells to infection (Movie 1 in the online version at https://doi.org/10.1016/bs.aivir.2019.07. 005, Fig. 2). When mammalian cells infected with a fluorescent bunyavirus (Shi et al., 2010) are studied by live cell imaging, it can be observed that some cells rapidly build a virus factory and produce many progeny viruses (Fig. 2, cell 1), a few cells die soon (Fig. 2, cell 2) and some others apparently do not get infected (Fig. 2, cell 3). Indeed, some cells receive the fluorescent virus and pass it to neighboring cells while remaining apparently non-infected (Fig. 2, cell 4). These findings demonstrate that cells in the cultured cell monolayer are not identical and respond to virus infection heterogeneously.
An interesting case was reported in a study of mosquito cells infected with an arbovirus (López-Montero and Risco, 2011). Video-microscopy showed that all infected cells went through three different stages of infection, early, acute and persistent, something that can be fully appreciated only when following each single cell in real time (López-Montero and Risco, 2011). Live-cell imaging has been used to study the biogenesis, dynamics and trafficking of viral replicative structures. Remarkably, these studies have shown that unrelated viruses such as Vaccinia Virus, Hepatitis C Virus, rotaviruses and the mouse hepatitis coronavirus, assemble small replication organelles that move through the cytoplasm in a microtubule-dependent manner, together with larger replication platforms that are rather immobile (Baktash and Randall, 2019; Eichwald et al., 2012; Eyre et al., 2014; Hagemeijer et al., 2010; Schramm et al., 2006; Wölk et al., 2008). Live-cell imaging has also shown the transformation of cell compartments induced by viruses to build their replication organelles. This is the case of the enterovirus coxsackievirus B3 that disassembles the Golgi complex (Van der Schaar et al., 2016) or the human reovirus that remodels the endoplasmic reticulum to build viral inclusions (Tenorio et al., 2018). New probes for imaging viral RNA will be powerful tools to study viral replication and assembly in live cells (Alonas et al., 2016).
For obtaining reproducible results by live cell imaging, the experiment must be carefully designed and adequate controls have to be included. Before starting, a number of important factors should be taken into account. Fluorescent probes must be compatible with in vivo conditions (Galas et al., 2018). Cells have to be grown on special plates with a clear bottom made of plastic or glass and plated with an appropriate confluence, usually 70% or less. A previous time-course study by confocal microscopy of fixed cells is important to determine the best time post-infection for launching the live cell imaging recording. To limit potential effects caused by phototoxicity, the right choice of fluorescent proteins, light intensity, exposure time and time interval between frame acquisitions will be critical (Magidson and Khodjakov, 2013; Icha et al., 2017).
For a detailed understanding of events, either expected or rare, live cell imaging is a powerful partner of EM through the methods we know as Correlative Light and Electron Microscopy (CLEM) (see Section 4).
(Continues…)
Excerpted from "Advances in Virus Research"
by .
Copyright © 2019 Elsevier Inc..
Excerpted by permission of Elsevier.
All rights reserved. No part of this excerpt may be reproduced or reprinted without permission in writing from the publisher.
Excerpts are provided by Dial-A-Book Inc. solely for the personal use of visitors to this web site.
Table of Contents
1. The viral replication organelles within cells studied by electron microscopyMartin Sachse, Isabel Fernández de Castro, Raquel Tenorio and Cristina Risco
2. Structures of enveloped virions determined by cryogenic electron microscopy and tomography
Robert Stass, Weng M. Ng, Young Chan Kim and Juha T. Huiskonen
3. CryoEM reconstruction approaches to resolve asymmetric features
Daniel J. Goetschius, Hyunwook Lee and Susan Hafenstein
4. Structural insights into coronavirus entry
Maria Alejandra Tortorici and David Veesler
5. Structural analysis of pleomorphic and asymmetric viruses using cryo-electron tomography and subtomogram averaging
Martin Obr and Florian KM Schur
6. The application of atomic force microscopy for viruses and protein shells: Imaging and spectroscopy
Pedroa J. de Pablo
7. Structural mass spectrometry goes viral
Jasmin Dülfer, Alan Kadek, Janine-Denise Kopicki, Boris Krichel and Charlotte Uetrecht
8. Illuminating the virus life cycle with single-molecule FRET imaging
Maolin Lu, Xiaochu Ma and Walther Mothes
9. Polyhedra, spindles, phage nucleus and pyramids: Structural biology of viral superstructures
Fasséli Coulibaly