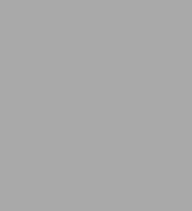
British Bat Calls: A Guide to Species Identification
204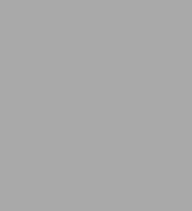
British Bat Calls: A Guide to Species Identification
204Paperback(First Edition)
-
PICK UP IN STORECheck Availability at Nearby Stores
Available within 2 business hours
Related collections and offers
Overview
Includes the properties of sound, how bats use sound, bat detectors and recording devices, call analysis. For each species in the British Isles, information is given on distribution, emergence times, flight and foraging behaviour, habitat, echolocation calls and social calls.
Product Details
ISBN-13: | 9781907807251 |
---|---|
Publisher: | Pelagic Publishing |
Publication date: | 07/02/2012 |
Series: | Bat Biology and Conservation |
Edition description: | First Edition |
Pages: | 204 |
Product dimensions: | 6.10(w) x 9.10(h) x 0.60(d) |
About the Author
Read an Excerpt
CHAPTER 1
Introduction
In 1793, Lazzaro Spallanzani, an Italian Catholic priest, biologist and physiologist, demonstrated that bats were able to avoid obstacles without the aid of vision. He stretched thin wires with small bells attached across a completely darkened room and observed that bats were able to fully navigate between them without causing the bells to ring. Blinding the bats also did not impair their ability to manoeuvre around them. Meanwhile, a Swiss zoologist, Charles Jurine, revealed that blocking one of the ears of a bat spoiled its navigating abilities, a finding that Spallanzani then pursued. A series of experiments which involved blocking the ears or gluing the muzzle closed led him to conclude that while bats did not have much use for their eyes, any interference with their ears that adversely affected hearing was disastrous, resulting in them colliding with objects they could usually avoid and being unable to forage for prey. He concluded that 'The ear of the bat serves more efficiently [than the eye] for seeing, or at least for measuring distance'. At the time, Spallanzani's findings were met by his fellow scientists with ridicule and scepticism as bats were believed incapable of producing any sound and therefore such results defied logic.
Nearly 150 years after Spallanzani's work, Donald R. Griffin, while an undergraduate at Harvard University in the 1930s, took an interest in Spallanzani's 'bat problem'. New advances in technology allowed him to use a 'sonic receiver', designed and built by Harvard physics professor George Washington Pierce. This device captured high-frequency sounds that were beyond the range of human hearing, and reduced the pitch to an audible level. For the first time, it became apparent that bats emit short, loud, ultrasonic clicking sounds. Along with a fellow student, Robert Galambos, who was an expert in auditory physiology, Griffin designed a set of further experiments which showed that bats were avoiding obstacles by hearing the echoes of their ultrasonic cries. Further experimentation revealed that bats were able to adjust the structure of their calls for prey search and capture and for collision avoidance. Griffin named this acoustic orienting behaviour 'echolocation'.
A bat's echolocation system is highly sophisticated. By emitting short high-frequency pulses of sound from their mouths or noses, bats are able to use the information contained within the echoes returned from a solid object to construct a 'sound picture' of their environment. Not only are they able to identify the size, position and speed of objects within three-dimensional space, they are also able to differentiate forms and surface textures. However, as there is no single signal form that is optimal for all purposes, bats have evolved a large number of signal types. This diversity of echolocation signals is likely to reflect adaptations to the wide range of ecological niches occupied by different bat species. For example, in the UK, the noctule, which largely forages high over parkland, pasture and woodland in an uncluttered environment, tends to produce extremely loud low- frequency calls of relatively long duration, narrow bandwidth and low repetition rate. Conversely, Bechstein's bat, which often forages very close to or within woodland vegetation in a very cluttered environment, usually produces relatively quiet, very broadband calls of short duration with a high repetition rate. Thus the calls of different bat species are shaped by the habitats in which they usually forage and the resulting different call types can often be used to separate species in the field. However, echolocation call shape is not fixed for a species and shows a certain degree of plasticity depending on the habitat within which an individual is currently located. In addition, although habitat is a significant factor determining the 'shape' of bat echolocation calls, they may also vary with sex, age and body size, geographic location and presence of conspecifics. Finally, species that occupy similar niches may use similar echolocation call types and there is often significant overlap in calls between species. An understanding of these different levels of variation both within and between individuals and species is essential to the successful use of echolocation calls for bat species identification.
Social calls produced by bats are often more structurally complex than echolocation calls used for orientation. Social calls are used to communicate with other bats, and for many species they consist of a wide variety of trills and harmonics, comparable in many respects to bird song. It is often surprising to discover just how musical these creatures sound. Social calls may have a number of functions. Some are used to defend patches of insects against other bats or to sustain territorial boundaries. Others function in attracting a mate or, in the case of distress calls, to initiate a mobbing response. Perhaps the most astounding are the isolation calls emitted by young bats, which allow their mothers to identify them. At Bracken Cave in Texas, for example, millions of Mexican free-tailed bats (Tadarida brasilensis) cluster in a large maternity colony. After the mothers have given birth the walls of the cave are literally covered with young bats packed tightly together. Each of these young bats produces an individually specific call that is in some way different from that of all the other young bats. These variations enable a returning mother to distinguish her own offspring amongst all the others.
Since Griffin's discovery, a number of techniques have been developed to allow us to listen to the ultrasonic vocalisations of bats. These range from relatively cheap 'heterodyne' detectors which convert a narrow range of frequencies into an audible signal in the field to 'real time' recording that has become possible through the development of high-speed analogue to digital converters built into or connected to computers or solid-state recorders. These high-tech devices utilise a sufficiently high sample rate to enable the ultrasound to be captured digitally without any subsequent processing and allow later processing and analysis of recordings. More recently, bat enthusiasts and researchers have been taking advantage of the explosion in the availability of smartphones. Currently, these devices can be used as recording devices when connected to a bat detector that converts the ultrasound into the audible range. However, with the very recent development of small inexpensive USB ultrasonic microphones, the possibility of turning a smartphone into a 'real time' ultrasound recording device is not far off. Incorporation of classification algorithms (a small number of which are available or under development and which assign these calls to species) into such equipment will one day provide instant identification of bat species in the field in a readily available, cost-effective hand- held device.
Donald Griffin referred to his discovery of echolocation as 'opening a magic well' from which scientists have been extracting knowledge ever since. Echolocation provides a window into the lives of bats, giving us access to a previously unknown world. It has been used, for example, to help us identify individuals to species; locateroost sites, find commuting routes and foraging areas; study foraging behaviour; establish species distributions; and monitor annual variations in bat populations. In addition, the study of the social calls of bats has allowed us to investigate the vocabulary of bat communication. Not only can these calls be used to identify species of bat and individuals, some calls can also be used to assess male territoriality and female selection of mates as well as providing a measure of male reproductive success while others can give us an insight into female and young interactions, food competition at foraging sites and levels of distress. However, although a great deal has already been learned about the vocalisations of bats, much still remains to be discovered.
The importance of sound to bats cannot be underestimated. They rely upon sound to locate food, to find their way around in the dark, and to seek out and communicate with other bats. By using ultrasonic detectors to eavesdrop on them we are able to investigate their behaviour in the field without disturbing and endangering these remarkable mammals. In this book, I provide a guide to listening to, recording and analysing the echolocation and social calls of bat species found in the UK. Although we cannot always reliably identify all bat species from their echolocation calls, I have tried to give as much information as possible on how to identify bats from their calls using different types of bat detectors.
CHAPTER 2Bats and sound
2.1 Properties of sound
Sound is a form of energy, which travels through a medium such as a solid, liquid or gas. It is produced when the medium is disturbed in some way by a moving surface such as a loudspeaker cone. As the cone moves forward, the air immediately in front is compressed, causing a slight increase in air pressure. It then moves backwards, past its rest position, and causes a reduction in the air pressure (rarefaction). The process continues so that a wave of alternating high and low pressure is radiated away from the speaker cone at the speed of sound in air (340 m·s-1) (Figure 2.1a). This process can also be thought of as a wave travelling through the air (Figure 2.1b). The wavelength (λ) is the length of one cycle of the wave (e.g. one high-pressure peak to the next high-pressure peak) and the amplitude is the height of the wave, which is related to the amount of energy the wave contains. Although the word 'amplitude' is used in this book, it is more of a relative measurement that is representative of scientific measurements such as intensity, pressure and voltage. The most commonly used is the pressure or sound pressure level (SPL) which is measured in decibel units (dB). The decibel is a logarithmic unit, used in a number of scientific disciplines. In acoustics the decibel is most often used to compare sound pressure in air, with a reference pressure.
2.1.1 Amplitude
The amplitude is related to the amount of energy contained within thewave (Figure 2.2). In other words, the energy or 'power' of the wave is proportional to the amplitude (A) squared (i.e. A). In terms of the human voice it is the difference between a loud (large amplitude) and a quiet (low amplitude) voice. To produce a wave with higher amplitude, the cone of the loudspeaker moves further away from the rest point in both directions (and therefore more energy is required to move the cone).
2.1.2 Frequency
If instead of altering the distance moved by the cone, we increase the rate at which it moves back and forward, in effect decreasing the wavelength, the frequency of the wave will increase, i.e. the number of waves (or areas of high and low pressure) that are produced per unit of time will increase (Figure 2.3). Two waves of the same duration and amplitude but with different frequencies will contain the same amount of energy (Figure 2.4).
2.1.3 Attenuation
In reality, as we move away from the loudspeaker the amplitude of a wave becomes smaller in size as the energy dissipates (Figure 2.5). Increasing the amplitude of a sound means that the sound (pressure differences) can be detected further away. It is the difference between a quiet and a loud voice. This is why we need to raise the volume of our voices to enable someone to hear us at the end of a long room. The two main mechanisms behind the loss of sound energy are spherical spreading and absorption.
The drop in sound intensity as the sound spreads out from its source is due to spherical spreading. If we imagine sound propagating from a source as a sphere that expands as it moves away from that sound source, we can see that as the sphere expands, its area must increase. Therefore the number of molecules over which the fixed amount of energy must spread must increase. This means that the amount of energy transferred to any one molecule decreases as the sphere expands. The amount of energy per unit area decreases with the square of the distance from the sound source.
In addition to energy losses due to spherical spreading, each collision between air molecules propagating a sound results in some loss of energy to heat, viscous properties of the medium, and absorption of energy between the molecules. These losses are known as 'absorption'. The energy lost to the medium in each collision also depends on how rapidly the molecules are being forced to move to propagate the sound. The higher the frequency, the higher the velocity, and thus the higher the energy loss. In most situations, therefore, higher frequencies lose energy during propagation much faster than lower frequencies. The amount of absorption can be affected by the relative humidity. The higher the humidity (and frequency), the greater the absorption.
2.1.4 Doppler shift
Suppose you are facing a loudspeaker producing a sound wave of 10 Hz. Each wave 'peak' will be reaching you at a rate of 10 per second. Now, imagine you start moving towards the loudspeaker at speed. The rate at which the waves reach you will increase. Although the loudspeaker is still producing waves of 10 Hz (or 10 waves per second), to you they will appear to be reaching you at a greater number of waves per second so that the frequency appears to be higher. The opposite is true if you start to move away from the loudspeaker. The waves will be reaching you at a slower rate so the frequency will appear to be lower. This is similar to the sound of a siren on an ambulance as it is driving past, except it is the ambulance that is moving whereas you are stationary. As it moves towards you the frequency you hear is higher because the waves appear squashed together. When it is level with you, you hear the true frequency produced by the siren. Then, as it drives away, the waves appear further apart and the perceived frequency drops. For bats, an echolocating common pipistrelle bat using a pure 46 kHz signal and flying at an average speed of 3.9 m·s-1 would result in a Doppler shift of 0.54 kHz (towards observer 46.54 kHz, away from observer 45.46 kHz), whereas a Leisler's bat using a pure 24 kHz frequency and flying at 7.9 m·s-1 would result in a Doppler shift of 0.56 kHz (towards observer 24.56 kHz, away from observer 23.34 kHz). The effect depends on the speed of the bat and the frequency it is emitting and can be calculated by the following equations:
fd = [(c + v)/c]f for a bat flying towards an observer
fd = [(c - v)/c]f for a bat flying away from an observer
where fd is Doppler frequency change (Hz), c is speed of sound in air (m·s-1), v is speed of bat (m·s-1) and f is echolocating frequency (Hz).
Examples of this variation for common pipistrelles and Leisler's bats are presented in Figure 2.6.
2.1.5 Summary
Sound waves can be varied by altering the amplitude (volume) and the frequency (pitch). Increasing the frequency will result in a decrease in the wavelength:
Frequency = speed of sound/wavelength (λ)
Waves lose sound energy as they travel from a source due to spherical spreading:
Energy [varies] 1/(distance)2
Increasing the amplitude increases the total amount of energy contained within the wave allowing it to be detected at greater distances:
Amplitude (A)2 [varies] Energy or Power
Low-frequency calls suffer less from attenuation than high-frequency calls so will also be able to travel further.
When a sound source producing waves at frequency f and an observer move relative to one another, the frequency of the sound f measured by the observer (f') depends on the details of the relative motion; this change in frequency is known as the Doppler shift.
2.2 Signal acquisition
In order to analyse any type of sound the signal must be converted from an analogue signal (a continuous time-varying signal) to a digital one (which represents the sound in the form of discrete amplitude values at evenly spaced points in time). This digital signal is then available for manipulation and analyses using a computer software program. However, for this conversion, there are two important parameters when digitising sound that can affect the 'recorded' signal. These are the sampling rate and sampling size.
(Continues…)
Excerpted from "British Bat Calls"
by .
Copyright © 2012 Jon Russ.
Excerpted by permission of Pelagic Publishing.
All rights reserved. No part of this excerpt may be reproduced or reprinted without permission in writing from the publisher.
Excerpts are provided by Dial-A-Book Inc. solely for the personal use of visitors to this web site.